Drugs
Species
Elimination half-life
Volume of distribution
Total systemic clearance
Comments
References
Propofol
Dogs
322 min
6.5 L/kg
50.1 mg/kg/min
No premed, no co-infusion
Beagle dogs
[5]a
486.2 min
3.38 L/kg
34.4 mg/kg/min
Premedication medetomidine 10 μg/kg, IM
Mixed breed dogs
Hall et al. [6]a
85.3 min
3.27 L/kg
53.35 mg/kg/min
Co-infusion Fentanyl 0.1–0.5 μg/kg/min) LD 2 μg/kg, IV
Greyhound dogs
Hughes and Nolan [7]a
53 min
6.6 L/kg
34 mg/kg/min
Cockshott et al. [8]a
74 min
2.46 L/kg
40 mg/kg/min
No premed, no co-infusion
Greyhound dogs
Mandsager et al. [9]a
Cats
527.7 min
6.83 L/kg
17.2 mg/kg/min
No premed, no co-infusion
[10]b
bolus
453 min
7.737 L/kg
11.5 mg/kg/min
Co induction Propofol/Ketamine 2 mg/kg each, IV (Ketofol)
Co-infusion Propofol/Ketamine 0.17 mg/kg/min each, IV (ketofol)
Zonca et al. [11]a
Ponies
69 min
0.89 L/kg
33.1 mg/kg/min
Premed detomidine 20 μg/kg, IV
Induction with ketamine 2.2 mg/kg IV
Co-infusion ketamine 50 μg/kg/min, IV
Nolan et al. [12]a
Sheep
56.6
1.037 L/kg
85.4 mg/kg/min
Premed acepromazine/papaveretum
No co-infusion
[13]a
50.3 min
1.515 L/kg
128 mg/kg/min
Same premed as above
Ketamine co-induction 1 mg/kg
Ketamine co-infusion VRI
Swine
1.42–2.72 L/kg
23–32.8 mg/kg/min
Isoflurane anesthetized pigs
Egan et al. [14]a
Alfaxalone
Dogs
24 min
2.4 L/kg
59.4 mg/kg/min
Alfaxalone 2 mg/kg IV bolus; no premed
Beagle dogs
[15]b
34.3 min
2.4 L/kg
48.5 mg/kg/min
Alfaxalone 2 mg/kg IV bolus; no premed
Greyhound dogs
[16]b
42.1 min
2.3 L/kg
36.9 mg/kg/min
Same as above with acepromazine and morphine, 0.03 and 0.3 mg/kg, respectively, IM
Alfaxalone
Cats
45.2 min
1.8 L/kg
25.1 mg/kg/min
Alfaxalone 5 mg/kg, IV bolus
[17]b
76.6 min
2.1 L/kg
14.8 mg/kg/min
Alfaxalone 25 mg/kg IV, bolus
Horses
33.4 min
1.6 L/kg
37.1 mg/kg/min
Alfaxalone 1 mg/kg IV with GGE 35 mg/kg, IV
Premed acepromazine/xylazine 0.03/1 mg/kg, IV
[18]b
Neonatal foals
22.8 min
0.6 L/kg
19.9 mg/kg/min
Alfaxalone 3 mg/kg, IV bolus
Premed butorphanol 0.05 mg/kg, IV
[19]b
Ketamine
Dogs
61 min
1.95 L/kg
39.5 mg/kg/min
Ketamine 15 mg/kg, IV, bolus
Kaka and Hayton [20]b
Cats
78.7 min
2.12 L/kg
21.33 mg/kg/min
Ketamine 25 mg/kg, IV, bolus
[21]
Horses
67.4 min
1.03 L/kg
0.076 mg/kg/min
Ketamine 0.08–0.025 mg/kg/min (stepped infusion)
Conscious horses for IV analgesia
[22]a
42 min
1.63 L/kg
26.6 mg/kg/min
Xylazine premed; Ketamine 2.2 mg/kg, IV, bolus
Kaka et al. [23]b
66 min
2.72 L/kg
31.1 mg/kg/min
Xylazine premed 1.1 mg/kg, IV
Induction ketamine 2.2 mg/kg, IV; halothane anaesthesia
Waterman et al. [24]b
90 min
1.43 L/kg
23.9 mg/kg/min
Premed detomidine 20 μg/kg, IV
Induction with ketamine 2.2 mg/kg IV
Co-infusion ketamine 50 μg/kg/min, IV
Nolan et al. 1996 [12]a
Ponies
15 min
0.012 L/kg
0.6 mg/kg/min
Ketamine 0.02 mg/mg/min, LD 0.6 mg/kg, IV
Conscious horses for IV analgesia
[25]a
PK values for S-ketamine only
S-Ketamine
7.8 min
0.013 L/kg
1 mg/kg/min
S-Ketamine 0.01 mg/kg/min, LD 0.3 mg/kg, IV
Conscious horses for IV analgesia
Table 31.2
Examples of infusion protocols for analgesic adjuncts in different species
Drugs | Species | Loading dose IV | Infusion rate IV | Comments | Reference |
---|---|---|---|---|---|
α 2 adrenoceptor agonists | |||||
Xylazine | Horse | 0.035–0.07 mg/kg/min | With ketamine 0.09–0.15 mg/kg/min | [26] | |
Horse | 1.1 mg/kg | 1–1.5 mL/kg/h | GKX triple drip with 0.5 mg/mL of xylazine, 1 mg/mL ketamine and 50 mg/mL guaifenesin | [27] | |
Medetomidine | Horse | 0.007 mg/kg | 0.0035 mg/kg/h | With propofol 0.01–0.11 mg/kg/min | [28] |
Dog/cat | 0.001–0.002 mg/kg/h | [19] | |||
Dexmedetomidine | Dog/cat | 0.0005–0.001 mg/kg/h | [29] | ||
Horse | 0.0035 mg/kg | 0.0001–0.000175 μg/kg/h | [30] | ||
Ketamine | Dog/cat | 0.2–0.5 mg/kg | 5–20 μg/kg/min | The author usually uses 5–10 μg/kg/min to limit possible side effect resulting from ketamine infusion | [29] |
Horse | 40–50 μg/kg/min | With propofol 0.12–0.16 mg/kg min | |||
Opioids | |||||
Morphine | Dog | 0.1–0.2 mg/kg/h | [29] | ||
Horse | 0.05–0.15 mg/kg | 0.03–0.1 mg/kg/h | With or without anaesthesia | ||
Fentanyl | Dog | 0.005 mg/kg | 0.2–0.5 μg/kg/min | With propofol 0.1–0.3 mg/kg/min | [29] |
Feline | 0.001 mg/kg | 0.1 μg/kg/min | With propofol 0.1–0.3 mg/kg/min | ||
Remifentanil | Dog/cat | 0.2–0.6 μg/kg/min | With propofol 0.3–0.5 mg/kg/min | ||
Feline | 0.2–0.3 μg/kg/min | With propofol 0.3 mg/kg/min | |||
Miscellaneous | |||||
Lignocaine | Dog | 2–4 mg/kg | 25–80 mic/kg/min | [29] | |
Horse | 1.5–5 mg/kg | 0.025–0.1 μg/kg/min | [2] |
Table 31.3
Examples of infusion protocols for the most common intravenous hypnotics used for TIVA in veterinary medicine
Drugs | Species | Premedication | Induction IV | Infusion rate, IV | Comments | References |
---|---|---|---|---|---|---|
Propofol | Dogs | As required | Propofol 2–6 mg/kg | Propofol 0.1–0.5 mg/kg/min | Dose rate will vary depending on analgesia and procedure | [29] |
Dogs | Acepromazine 0.03 mg/kg, IM Methadone 0.2 mg/kg, IM | Target of 3 μg/mL | Target 2.7–3.4 μg/mL Dexmedetomidine plasma target of 0.85 ng/mL | TCI propofol Stepped infusion | [35] | |
Cats | As required | Propofol 4–8 mg/kg | Propofol 0.12–0.5 mg/kg/min | Dose rate will vary depending on analgesia and procedure | [29] | |
Horses | Detomidine | Propofol 2 mg/kg | Propofol 0.18 mg/kg/min | VRI | [36] | |
Horses | Xylazine 1 mg/kg, IV Midazolam 0.05 mg/kg, IV | Propofol 3 mg/kg Poor quality | Propofol 0.16 mg/kg/min Ketamine 3 mg/kg/h | VRI CRI | [32] | |
Horses | Medetomidine 0.007 mg/kg, IV | Ketamine 2 mg/kg | Propofol 0.1 mg/kg/min Medetomidine 3.5 μg/kg/h | VRI CRI | [28] | |
Horses | Medetomidine 0.005 mg/kg, IV | Ketamine 2.5 mg/kg Midazolam 0.04 mg/kg | Propofol 0.14 mg/kg/min Ketamine 1 mg/kg/h Medetomidine 1.25 μg/kg/h | VRI CRI CRI | [37] | |
Calves | Propofol 5 mg/kg | Propofol 0.6–0.6 mg/kg/min | VRI | [38] | ||
Goats | Propofol 2.5–6 mg/kg | Propofol 0.2–0.6 mg/kg/min | VRI | |||
Alfaxalone | Dogs | As required | Alfaxalone 2–3 mg/kg | Alfaxalone 0.07–0.15 mg/kg/min | VRI | |
Cats | As required | Alfaxalone 1.7–5 mg/kg | Alfaxalone 0.11–0.18 mg/kg/min | VRI | ||
Horses | Acepromazine 0.03 mg/kg, IV Medetomidine 0.007 mg/kg, IV | Guaiphenesin 35 mg/kg Alfaxalone 1 mg/kg | Alfaxalone 2 mg/kg/h Medetomidine 5 μg/kg/h | Colt castration | [46] | |
Sheep | Alfaxalone 2 mg/kg | 10 mg/kg/h | [47] | |||
Swine | Alfaxalone 4 mg/kg, IM Medetomidine 0.04 mg/kg, IM Butorphanol 0.4 mg/kg, IM | Alfaxalone 1.2 mg/kg | 0.08 mg/kg/min | VRI Placement of epidural catheter | [48] | |
Green Vervet monkeys | Ketamine 5 mg/kg, IM | Alfaxalone 0.4–1.2 mg/kg | 0.15 mg/kg/min | VRI Imaging study | ||
Ketamine | Dogs | 2–20 μg/kg/min | CRI to provide analgesia in order to reduce anaesthetic requirements | [29] | ||
Cats | 0.15–0.46 μg/kg/min | CRI to provide analgesia in order to reduce anaesthetic requirements | ||||
Horses | α2 agonist | Ketamine Diazepam | Triple drip with GGE and α2-adrenoceptor agonist | See main text |
Ideal drugs for intravenous anesthesia and analgesia should have a rapid onset of action, quick metabolism, and elimination time. Amongst the important PK parameters that veterinarians need to be familiar with, is the context sensitive half-time of elimination (CSHT) . This parameter highlights the fact that the elimination half time of a drug during an infusion may be dynamic and may vary with the duration of the infusion, the context. Very little information exists in veterinary medicine about the CSHT of the drugs used for infusion. In addition, like any other PK parameters in veterinary medicine it will also vary between species. For example CSHT for fentanyl in humans increases substantially after 2–3 h of infusion, while this has not been observed in dogs and cats where a steady state is reached after 2–4 and 6 h of infusion, respectively [54–58].
In addition to PK knowledge of the drugs, the (specialist) veterinary anesthetist must be aware of the pharmacodynamic (PD) differences that can exist between species for any given agent. In some cases, drugs which are considered safe for infusion in some species may have dramatic and even lethal effects in others. For example, lidocaine infusion may induce cardiovascular depression in the isoflurane anesthetized cat [59]. Still in the cat, propofol is suspected to be responsible of Heinz Body formation anaemia when infused daily for 2–3 consecutive days [60].
The total intravenous techniques used to maintain anesthesia in animals are not different in principle from those described for human (see Chaps. 6 and 25). Repeated bolus dose administration (intermittent administration) is the simplest technique and the most commonly used for short procedures such as skin biopsies, ultrasound or X-ray in small animals (dogs and cats), and field castration in the horse. When providing continuous infusion, the easiest method involves a fluid bag containing the hypnotic mixture and a giving set attached to an intravenous catheter. With this system, the flow rate can be adjusted varying the diameter of the infusion set using a regulating clamp (Fig. 31.1). The infusion rate can be calculated from knowledge of the volume of each drop of fluid. This methodology is commonly used for “field” anesthesia in the horse (Figs. 31.2 and 31.3). The veterinarian must remember that as the fluid disappears from the infusion bag, the infusion rate will reduce and will therefore need frequent re-adjusting. Although this method provides a more consistent level of anesthesia by comparison with intermittent administration, it has a high potential for miscalculation and overdose, mainly in smaller patient (i.e. cats) and burettes or micro drips are advised for use in those cases. The use of infusion pumps and syringe drivers brings more safety and precision to the continuous infusion. These devices have seen their technology and safety as well as their affordability increase over the last few decades and their use has therefore become more and more common in the veterinary world.
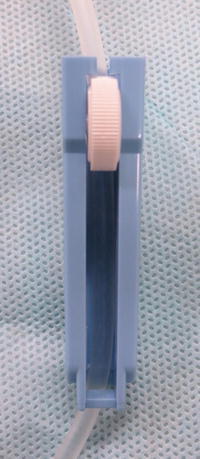
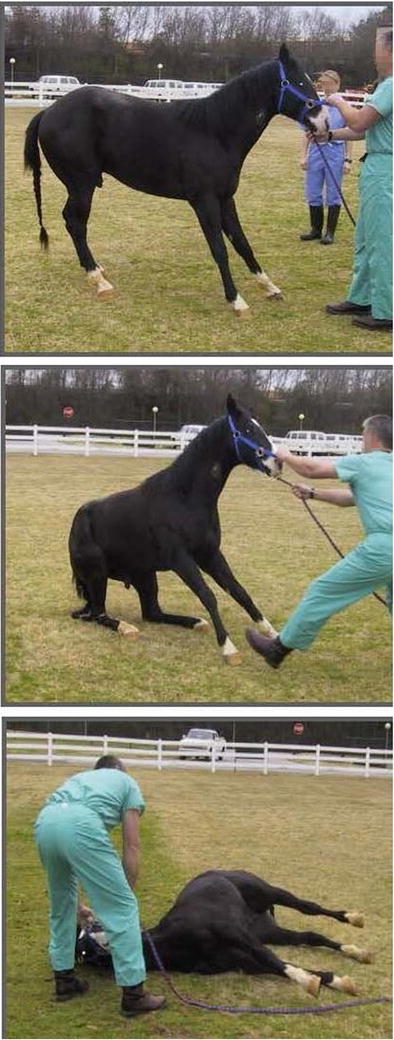
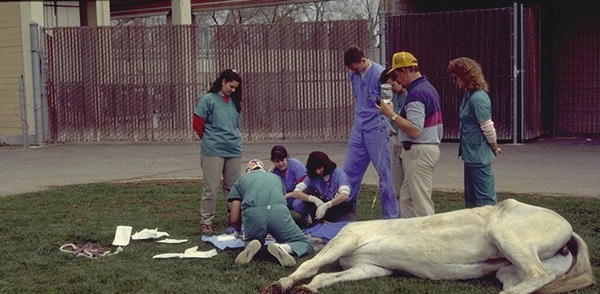
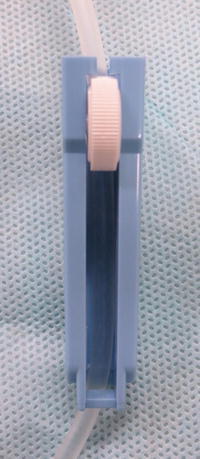
Fig. 31.1
Regulating clamp used to modify fluid rate through a giving set
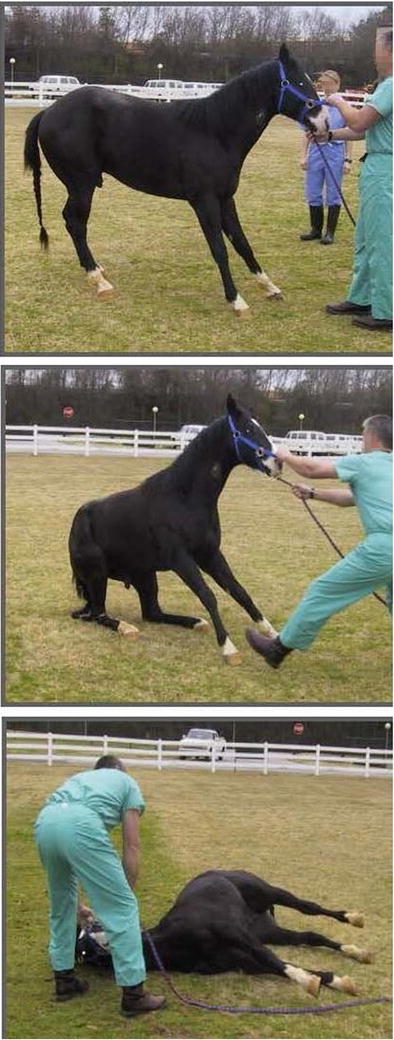
Fig. 31.2
Equine field anaesthesia —induction of anesthesia
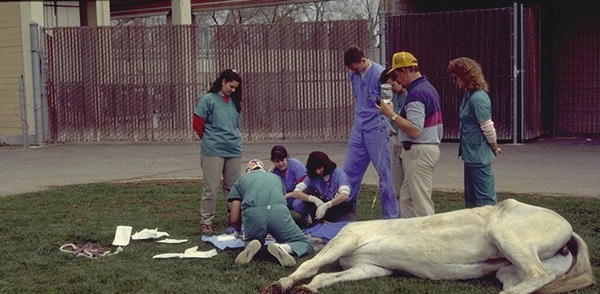
Fig. 31.3
Equine field anesthesia —maintenance of anaesthesia using a triple drip based protocol
While most of the veterinarians doing TIVA use recipes or published infusion rates (Table 31.2), veterinary anesthesia is seeing the development of pharmacokinetic-dependent infusion techniques to allow specific drug plasma concentrations to be reached. Although most of those PK dependent techniques are mainly used in teaching and referee centres for research purposes, their use has been described in a few clinical cases. Research groups have developed stepped infusion systems for different agents such as for medetomidine or dexmedetomidine in the dog [35] or ketamine in the horse [22]. Stepped infusion systems consist of a group of well-defined different infusion rates which are started at specific times and have been developed so that a desired plasma concentration is reached rapidly and with little accumulation or hangover. This system unfortunately is very rigid and does not allow for target plasma concentration changes. Therefore, the Target Controlled Infusion (TCI) system has been introduced where a computer programmed with the PK of a specific drug for a determined species drives the infusion. The TCI system will maintain a stable desired drug plasma target concentration while adapting the infusion rate to compensate for accumulation. Some TCI systems have been developed in veterinary medicine, but, they have not yet found their way into clinical anesthesia. This is mostly due to the limited availability of hardware and software and due to the paucity of evaluation of population pharmacokinetics. Different drugs have been studied and their PK parameters identified for use in TCI system for different species : propofol in the dog, and ketamine and detomidine (an α2-adrenoceptor agonist licenced in the horse) in the equine patient [61–63]. The ketamine TCI system for the horse is still in development and a team from Switzerland has recently determined and validated a set of PK parameters in ponies [63]. A few years earlier, in 2006, Knobloch et al., developed a physiologically based pharmacokinetic (PKPB) model for ketamine in ponies targeting low dose of ketamine (S-ketamine 1 μg/mL) to provide analgesia [64]. The models provided reliable prediction of plasma level of R- and S-ketamine. In the dog , a TCI system for propofol (Figs. 31.4 and 31.5) was developed and validated by Beths et al. in 2001 and has since been used in different clinical settings such as neurosurgery [61, 65]; cardiac surgery in a patient with ductus arteriosus [66]; and neutering procedures [67, 68]. In addition, combinations with other agents have been studied: remifentanil, medetomidine and dexmedetomidine [35, 67, 69]. Furthermore, Beths in 2008 and Beier et al., in 2009, looked at the influence of medetomidine and dexmedetomidine, and remifentanil infusion, respectively, on the minimum propofol target concentration needed to stop reaction to supramaximal stimulation in dogs (Table 31.4) [35, 67, 69].
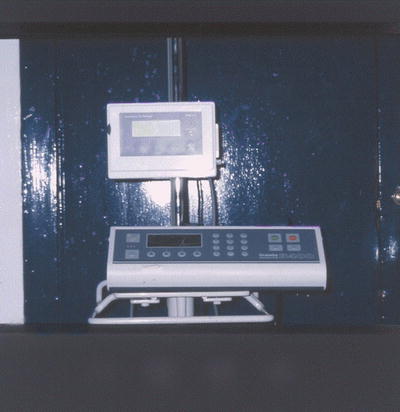
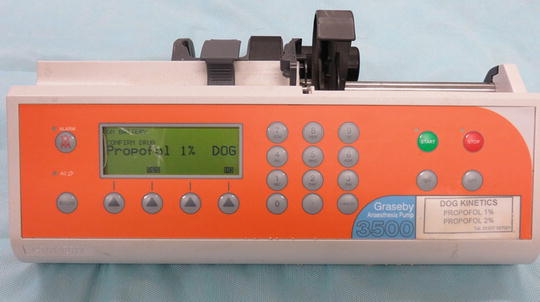
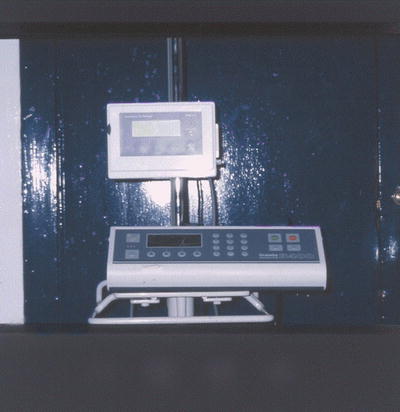
Fig. 31.4
TCI for propofol in the dog —first version
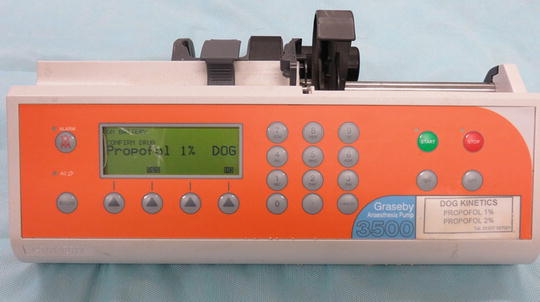
Fig. 31.5
TCI for propofol in the dog —second version
Table 31.4
Examples of minimum infusion rates (MIR) for propofol in different species
Species | Premedication | Induction IV | MIR IV | Comments | References |
---|---|---|---|---|---|
Dogs | Acepromazine, IM Methadone, IM | Propofol TCI Target 3 μg/mL | Propofol TCI, mCp 3.3 (±1.04) μg/mL | Co-infusion Dexmedetomidine, Measured Plasma concentration of 0.83 ng/mL | [35]a |
Propofol TCI, mCP 6.2 (±1.35) μg/mL | No co-infusion | ||||
None | Propofol 6 mg/kg | 0.51 (±0.08) mg/kg/min | No co-infusion | [70]a | |
0.42 (±0.08) mg/kg/min | Co-infusion Lidocaine 0.25 mg/kg min CRI (LD 1.5 mg/kg) | ||||
0.31 (±0.074) mg/kg/min | Co-infusion Lidocaine 0.25 mg/kg/min, CRI (LD 1.5 mg/kg) Ketamine 0.1 mg/kg/min, CRI (1 mg/kg) | ||||
None | Propofol 6 mg/kg | 0.76 (±0.1) mg/kg/min | No co-infusion | [71]a | |
Propofol 5 mg/kg Ketamine 2 mg/kg | 0.6 (±0.1) mg/kg/min | Co-infusion Ketamine 0.0.025 mg/kg/min | |||
Propofol 4 mg/kg Ketamine 3 mg/kg | 0.41 (±0.1) mg/kg/min | Co-infusion Ketamine 0.05 mg/kg/min | |||
Cats | None | Propofol 0.05–0.1 mg/kg/min | 0.21 (±0.02) mg/kg/min | No co-infusion | [51]b |
0.14 (±0.01) mg/kg/min | Co-infusion Ketamine 0.023 mg/kg/min | ||||
0.14 (±0.01) mg/kg/min | Co-infusion Ketamine 0.046 mg/kg/min | ||||
Horses | Medetomidine 0.007 mg/kg, IV | Propofol 2 mg/kg | 0.06–0.1 mg/kg/min | Co-infusion Medetomidine 0.0035 mg/kg/h | |
Xylazine 1 mg/kg, IV | Propofol 3 mg/kg | 0.1 (±0.02) or mCp 5.3 (±1.4) μg/mL | None | [74]a | |
None | Propofol 7 mg/kg | 0.2 (±0.03) or mCp 17.5 (±4.0) μg/mL | None | [75]a | |
Rabbit | None | Propofol 1 mg/kg/min | 0.64–1.19 mg/kg/min | None | [76]c |
To decrease the dose of drugs used and therefore reduce their potential side effects, anesthetists balance their anesthesia by combining different agents. Doing so, they manage to not only decrease the amount of drug used, but also give a more stable anesthesia with muscle relaxation, analgesia, hypnosis and cardiorespiratory function that are improved. The most commonly used agents in addition to the hypnotics to balance anesthesia are the α2-adrenoceptor agonists , ketamine, the opioids and lidocaine, to name a few (Table 31.2).
The α2-adrenoceptor agonists provide in most species a reliable dose-dependent analgesia, muscle relaxation and sedation. They are therefore ideal agents for balanced anesthesia and are the most widely used sedatives in veterinary anesthesia. Unfortunately their cardiovascular effects, characterised by an increase in blood pressure and systemic vascular resistance with reduced heart rate and cardiac output, are limitations to their use. While xylazine, romifidine and detomidine are most commonly used in the equine patient, medetomidine and its active enantiomer dexmedetomidine are used in dogs and cats. The sparing effect of the α2-adrenoceptor agonists has been shown on inhalant as well as IV agents such as propofol [35, 77].
Xylazine was synthesize in Germany in 1962 for use in human as an antihypertensive. It was the first α2–adrenoceptor used in veterinary medicine. Although its use is also licenced in dogs and in cats, it is mostly used in cattle and horses. Romifidine is licenced in horses and has a higher potency than xylazine. Detomidine and medetomidine are more potent than Romifidine. While detomidine is licenced for use in horses, medetomidine is licenced for use in dogs and cats. Medetomidine is a racemic mixture composed of dexmedetomidine, the active enantiomer and levomedetomidine the inactive one. While dexmedetomidine has been available in human medicine for a long time, it is only in the last decade that it became available to veterinarians. One of the advantages of this class of drugs is that their effect can be reversed with the use of α2-antagonists such as yohimbine and atipamezole. The former was developed to reverse the effects of xylazine in particular (dogs, cats, ruminants and exotic species), and the latter is used primarily to reverse the effects of medetomidine or dexmedetomidine in dogs, cats and several exotic species. The reversal agents are usually used to speed up the recovery and/or in an emergency situation. While their use is frequent in exotic species and with wild animals where prolonged recumbency may be associated with higher morbidity and mortality, in equine and small animal practices, veterinarians tend not to reverse it allowing the patient to recover slowly and calmly from the anesthesia. In fact, in horses, following inhalation anesthesia, it is recommended to give the horse a sedative, usually an α2-adrenoceptor agonist , while it is still anesthetised in the recovery box, to smoothen the recovery and to avoid any excitation which could result in self-induced wound or worse, limb fracture. Figure 31.6 shows some important phases of anesthesia in a horse, including recovery. When using a reversal agent, the analgesic effect of the α2-adrenoceptor agonists will be reversed and veterinarians must therefore ensure that analgesia is well under control using other agents such as opioids or local anesthetics.
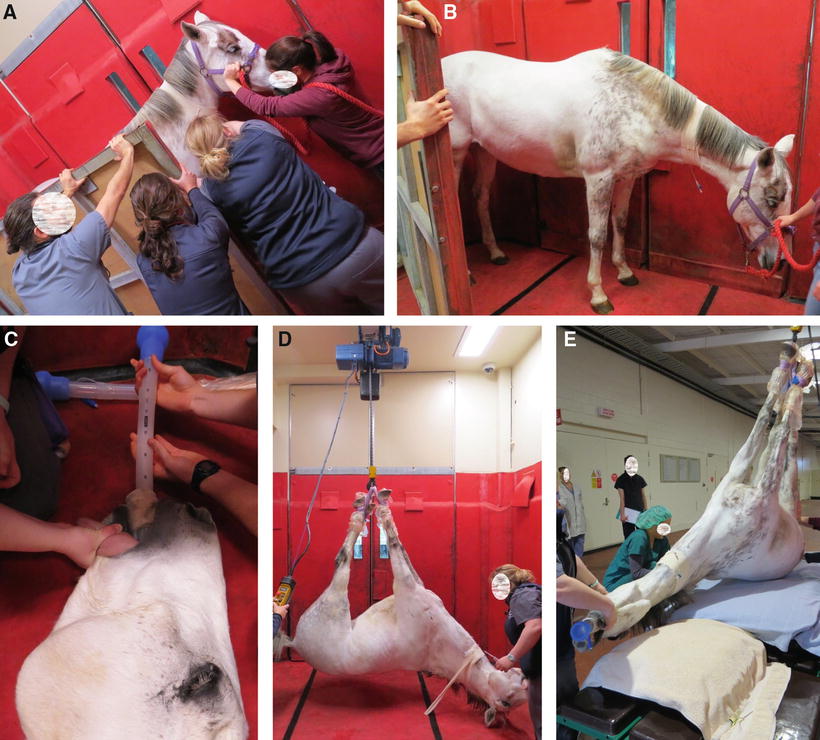
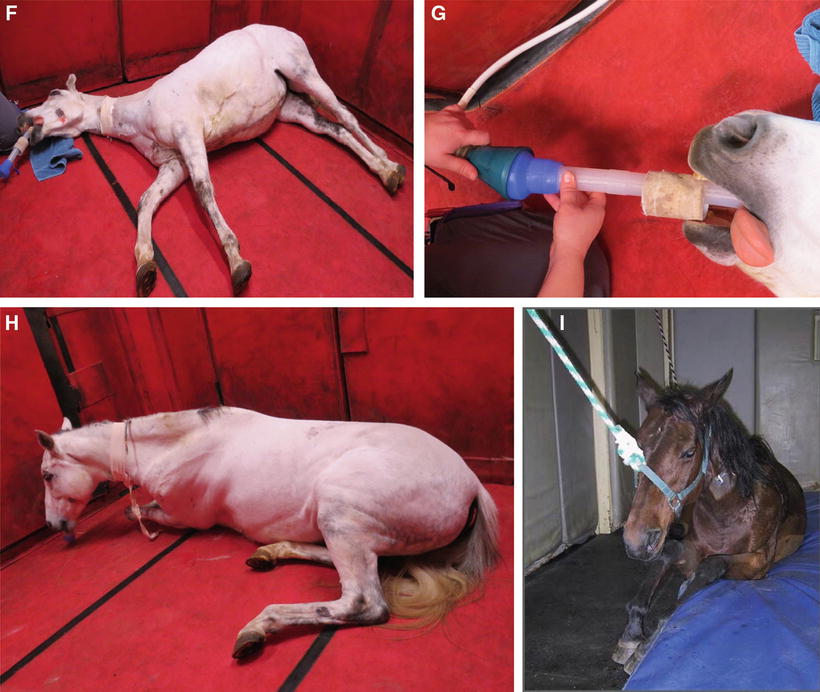
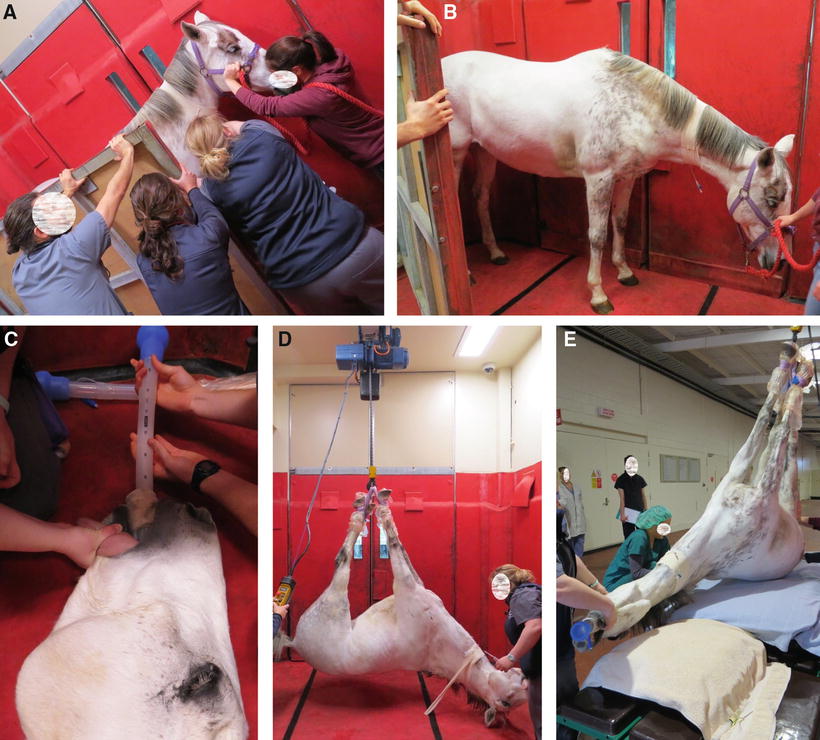
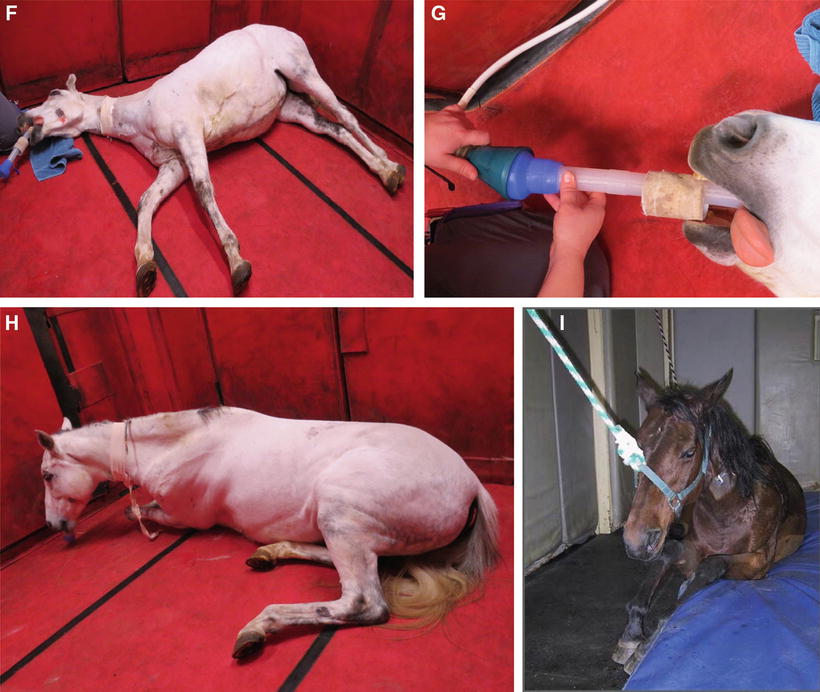
Fig. 31.6
Illustration of some important phases of equine anesthesia . (a) Sedation horse placed between a wall and a mobile panel to minimize lateral movement during induction; (b) induction of anaesthesia; (c) blind endotracheal intubation; (d) lifting of a horse under anaesthesia from the induction box to the surgery table; (e) placement of the horse on the surgery table; (f) horse recovering from anesthesia in a padded (wall and floor) recovery box; (g) same horse as previous, recovering from an anesthesia, still intubated and breathing 100 % O2 through a demand valve; (h) unassisted recovery; (i) assisted recovery
Ketamine is a dissociative agent which is used clinically to provide immobilization or anesthesia (wild animals, horses). In addition to its hypnotic effect, it also provides analgesia and is mostly used at low rate infusion for this effect in different species including dogs, cats and horses. NMDA antagonism is believed to be the most likely molecular mechanism responsible for most of ketamine’s clinical properties [78]. Through its NMDA antagonistic properties, ketamine is known as an antihyperalgesic agent that decreases wind-up and may provide pain relief in patients suffering from chronic pain. Infusion schemes have been developed in small animals as well as horses and its sparing effects have been well documented with inhalant as well as IV agents in different species (Table 31.2) [29, 31, 32, 34].
The opioids are known for their analgesic effects. Although agents characterised by short onset and short duration time such as fentanyl or remifentanil are more appropriate for constant rate infusion (CRI) , longer acting agent such as morphine or methadone have also been used in veterinary medicine (Table 31.1) [2, 29, 30, 33, 34]. Etorphine, a potent mu-opioid agonist (about 3000 times the potency of morphine), is mostly used for the restraint and the capture of wild animals. Its use as an infusion to maintain a patient under general anesthesia has been described in the elephant (Fig. 31.7) [79].
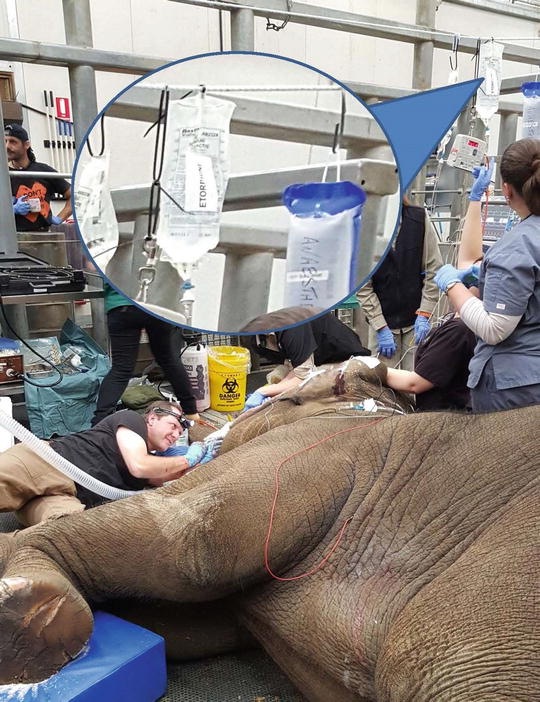
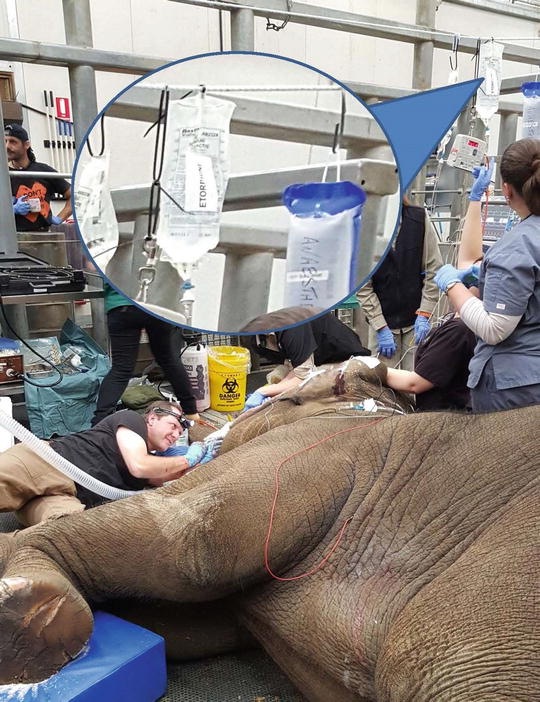
Fig. 31.7
Elephant undergoing tooth surgery and maintained anesthetized with etorphine infusion. The patient was intubated (Murphy style endotracheal tube ID = 30). The patient was breathing 100 % O2 and was ventilated (Intermittent Positive Pressure Ventilation) using a demand valve
Lidocaine is a sodium channel blocker local anesthetic that has been used intravenously to provide analgesia systemically. Its analgesic and anti-inflammatory effects are mediated, among others, by blockade of Na channels, inhibition of glycine receptors, inhibition of NMDA and neurokinin (NK) receptors, promotion of and the release of endogenous opioids. Although it is not recommended for infusion in the cat where severe cardiovascular depression has been observed when used as an infusion [59], it has been successfully used peri-and postoperatively in different species, including dogs and horses [2, 29].
Hypnotic Agents
Propofol
The properties of propofol differentiate it from other intravenous agents. A rapid onset of action, short duration due to high biotransformation, rapid redistribution and an apparent lack of accumulation made it the ideal agent for induction and maintenance of anesthesia in human as well as veterinary medicine.
It is a substituted isopropyl phenol which is insoluble in water and was first formulated with Cremophor EL (1977). Pain associated with injection as well as complement mediated adverse reactions to the Cremophor resulted in radical changes in its formulation [80]. Since 1986, it is formulated in an oil in water emulsion containing 1 % propofol, 10 % soybean oil, 2.25 % glycerol, and 1.2 % purified egg lecithin.
Although this formulation (macro emulsion ) is the most popular in human and veterinary medicine, pain on injection has still been reported in humans [81–83] as well as in dogs and cats [84, 85]. In addition, its lack of preservative allows for fungal and bacterial growths leading to a very short shelf life of about 12 h after which time any used vials and tubings (infusion) should be discarded (Label Diprivan® 451094 F/Issued: February 2014). To increase the shelf life of propofol and possibly decrease pain on administration, other formulations have been developed and tested such as a nano droplet formulation and a propofol containing 2 % benzyl alcohol preservative [10, 86]. Amongst the formulations developed to decrease the pain, the most successful one is fospropofol which is a prodrug of propofol. Unfortunately, PK studies in the dog and the rabbit have shown longer onset and duration of action when compared with the macro emulsion [76, 87].
Pharmacokinetics
In terms of pharmacokinetics, propofol’s rapid distribution and clearance are mostly responsible for its clinical success and make it the drug of choice for maintenance of anesthesia. In most species (but not cats) propofol does not seem to clinically accumulate after multiple injections or prolonged infusion resulting in rapid recoveries of relatively good quality. Following a single bolus IV administration, propofol rapidly reaches the brain and induces anesthesia. Then it will redistribute to other tissues, leaving the brain and terminating the anesthesia. In most species (but not cats) propofol is extensively and rapidly metabolised by the liver , resulting in the production of water soluble sulphide and glucuronide metabolites which will be excreted by the kidneys [88–90]. Propofol’s clearance from the plasma far exceeds the hepatic blood flow, which marks the importance of the peripheral tissue uptake as well as the possibility of extra hepatic metabolism (lung, kidney). The latter was confirmed when metabolites of propofol were still detected during the anhepatic phase of orthotopic liver transplantation in man [90]. Although lungs and kidneys have been suggested as possible sites for the extra hepatic metabolism, it is still debated and recent studies have confirmed the importance of the role of the kidneys over the lungs in the extra hepatic propofol clearance [91–95].
Pharmacodynamics
Regarding the central nervous system (CNS) , cerebral blood flow (CBF) regulation is not impaired by propofol administration while cerebral metabolic rate (CMR) is decreased which may protect neuronal function in compromised patients [96–98]. The decrease in CMR will also result in vasoconstriction, decreasing ICP (intracranial pressure). Therefore the use of propofol has been advised in humans as well as in dogs with intracranial diseases [99– 103].
The cardiovascular (CV) effects of propofol are a dose dependant decrease in myocardial contractility and a decrease in systemic vascular resistance (SVR) . The resulting decrease in blood pressure from baseline (no hypotension per se) does not usually trigger a compensatory increase in heart rate as observed with other agents such as thiopental. It has been speculated that in fact propofol resets the baroreceptor reflex set point [104].
Respiratory depression is an important side-effect of propofol and it will dose dependently decrease tidal volume and/or respiratory rate leading to apnea [84, 105]. The incidence of the latter is not only dose dependant, but also administration rate dependant [105]. The ventilatory response to hypoxemia and hypercarbia are decreased with propofol administration and this may be exacerbated with co-administration of opioids [18, 106, 107]. To avoid or limit the respiratory depression , it is advised to administer propofol slowly to effect [84, 108, 109].
It is a great muscle relaxant although myoclonic movements and muscle twitching as well as opisthotonos have been reported during anesthesia in the dog [84, 110–112]. The incidence of these reactions is greatly decreased with the use of preanesthetic tranquillisers, sedatives or opioids.
Propofol lacks analgesic properties, and therefore needs to be co-administered with analgesic agents such as opioids .
Propofol TIVA in Different Species (Table 31.3)
Canine
Although propofol may depress the CV system through myocardial depression and arteriolar and venous vasodilation, in healthy patient, hemodynamic stability is usually well maintained with a lower blood pressure than baseline and decrease in cardiac output but with minimal changes in heart rate [118, 119]. To avoid higher infusion rates to compensate for propofol’s lack of analgesia, analgesic agents (opioids, ketamine) are usually administered in the premedication (bolus) and/or the maintenance (bolus or more commonly as co-infusion period) phase of anesthesia. Although the aim is to decrease the propofol requirements and therefore the associated cardiovascular depression, in the healthy dog, the addition of potent opioids such as remifentanil during the maintenance period shows, in addition to the propofol sparing effect , a decrease in the heart rate (HR), stroke volume (SV) and cardiac output (CO) while still maintaining good arterial blood pressure [118, 120]. To limit those CV effects observed with opioid infusion, ketamine infusion has also been used during propofol based anesthesia: a combination sometimes referred to as Ketofol [119, 121]. Ketamine holds a particular place amongst anesthetic agents as it maintains or increases CO in the healthy patient as a result of increased sympathetic tone [122]. In a recent study in healthy beagle dogs, the combination resulted in a decrease of the amount of propofol used with an increase in HR and arterial blood pressure [119].
In the healthy dog, the main side-effect resulting from propofol administration is respiratory depression which tends to be exacerbated with the addition of opioids as well as ketamine and consequently the anesthetists must be ready to provide assisted ventilation [118, 119].
A recent retrospective study looking at myoclonus in dogs anesthetised with propofol TIVA reported a 1.2 % incidence [123]. This is much lower than the previously described incidences: 21 % [124], 7.5 % [111] or 46.1 % [125]. The difference may result from the fact that in the most recent study, myoclonus was precisely defined as involuntary muscle contractions which did not cease following bolus administration of propofol, and due to their intensity made continuation of anesthesia difficult without further treatment [123]. Any uncoordinated movements, tremor, paddling (running like movement) that could be explained by a lack of hypnosis or analgesia or were present during the awakening phase were not counted. For example, in the Hall and Chambers study [124] the dogs did not receive any analgesia in the premedication or during the procedure and therefore the observed movements could have been attributed to insufficient anesthesia and/or analgesia [124].
Pain on injection has been described in the dog and the incidence varies between 1 and 7.5 % [84, 85]. In a recent study (2012), Michou et al. reported a 3 % incidence with the macro emulsion compared with a 20 % incidence with a new lipid free formulation (propoClear®, Pfizer Animal Health, UK) [126]. Similar results with the lipid free emulsion were reported by Minghella in 2010 [127].
In a recent study, the induction and maintenance doses in unpremedicated animals were respectively 5.3 ± 1.1 and 0.6 ± 0.1 mg/kg [119]. In this study dogs were not undergoing any surgery and anesthesia was maintained for 60 min while the infusion rate was altered up or down following clinical assessment including ocular reflexes, jaw tone, swallowing reflexes and presence or absence of limb withdrawal following toe pinching. The authors did not observe any effect on the HR or the SpO2 and PaO2 by comparison to baseline while mean arterial blood pressure (MAP) was lower than baseline, but hypotensive events (MAP < 60 mmHg) were not reported by the authors (lowest MAP observed at was 78 ± 7 mmHg at time 40 min). Five of the ten dogs of the study had short (15 min) apneic events. The time to extubation was 9.4 min and the recovery quality was scored as smooth or with some paddling of short duration. Other infusion protocols are provided in Table 31.3.
The minimum infusion rate (MIR) and the minimum target concentration (TCI) for propofol necessary to block a response to a supramaximal stimulation (tetanic twitch) in 50 % of dogs has been determined with different co-infusions including dexmedetomidine, lidocaine and ketamine [35, 70, 61] (Table 31.4).
Feline
The CV and respiratory effects are very similar to those observed in the dog. The addition of analgesic agents such as potent opioids (fentanyl, alfentanil or sufentanil) in healthy un-premedicated cats undergoing ovariohysterectomy and maintained under anesthesia with propofol TIVA had similar effects as in dogs with a decrease in HR and arterial blood pressure as well as a respiratory depression (decrease respiratory rate, increase in EtCO2) [128]. On the other hand, Padilla and colleagues show that, in acepromazine premedicated cats also undergoing neutering procedures and receiving either remifentanil or alfentanil infusion in addition to propofol TIVA, HR and arterial blood pressure were equal or increased by comparison with baseline while ventilatory parameters were well maintained [129]. In earlier studies, it has been reported that HR and systolic arterial blood pressure (SAP) could increase in cats secondary to sympathetic activation in consequence to the administration of high doses of ultra-short acting opioids [130, 131]. While we cannot comment on the infusion rate for remifentanil, the alfentanil infusion rate was higher in the Padilla’s study at 0.5 versus 0.08 μg/kg/min and could have been responsible for the different CV parameters in that study. An additional explanation could be that as the cats underwent ovariohysterectomy in both studies, it is still possible that the surgical stimulation was different enough to have caused the observed difference.
In injured cats, cardiorespiratory variables were compared between propofol/fentanyl and isoflurane/fentanyl anesthesia. A higher requirement for respiratory system support through IPPV was found in the propofol group than in the isoflurane group (9/11 vs 1/11), but CV parameters such as arterial blood pressures in particular were better maintained in the propofol group [132].
In 2015, Campagna et al. reported that, in medetomidine premedicated cats undergoing ovariohysterectomy under propofol TIVA, 80 % of their patients required controlled ventilation [44]. In an earlier study in cats premedicated with morphine and medetomidine, undergoing neutering procedure, Beths et al. did not report post induction apnea or patients requiring controlled ventilation [49]. In the latter study, propofol based anesthesia was induced with less propofol (0.25 vs. 11.7 mg/kg). Although the propofol maintenance infusion rate was higher in the Beths et al. study (0.339 vs. 0.178 mg/kg/min), the recovery times were shorter (33.4 vs. 57 min). This was certainly the result of a shorter duration of anesthesia in the Beths et al. study (26.1 vs. 74 min) and the fact that it has been shown, in cats, that recovery time increases with longer propofol infusion time [133]. This is not really a surprise since we know that the PK of propofol in that species has a longer elimination half time which could result from slower metabolism. In humans, about 60 % of propofol metabolism is via glucuronidation through the glucuronyl transferase enzyme [134]. In the cat, the gene responsible for that enzyme has been classified as a pseudogene and is very likely non-functional [135]. Also in humans, a proportion of propofol undergoes phase one metabolism involving cytochrome P450 (CYP) microsomal enzyme followed by glucuronidation. While the specific enzyme has been identified in humans (CYP2B6) and in dogs (CYP2B11), this has not yet been done in the cat [136–138]. Moreover, Beths in 2008 did not observe much propofol related CYP activity in feline hepatic microsomal preparations by comparison to dogs and rats (Fig. 31.8) (unpublished data).
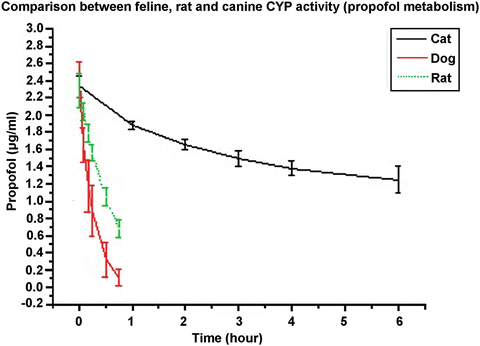
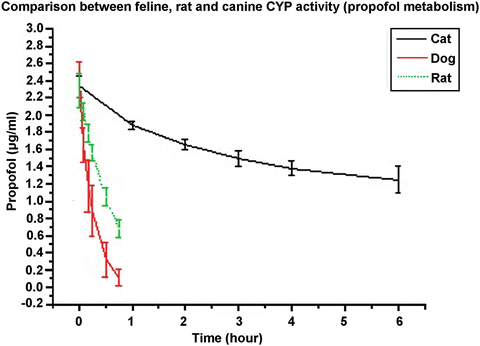
Fig. 31.8
Degradation of propofol concentrations (2.5 μg/mL) over time in rat, dog and cat hepatic microsomes. At each time, the mean value of two propofol determinations (HPLC) was calculated. Each point is the mean (± SD) propofol concentration from microsomes of six rats, dogs or cats. The incubation times in rats and dog were 0, 5, 10, 15 30 and 45 min while in cats the incubation times were 0, 1,2 3, 4 and 6 h. Isolation of the microsomes followed the methods described by Rutten et al. [139] and Correia [140]. Propofol was extracted from the microsomes and was analysed by HPLC with fluorescence following the method described by Plummer [141]
In addition to this slower metabolism, the use of propofol for TIVA in the cat is still controversial. In a study in 1989, Andress et al. reported, following repeated daily administration of propofol, oxidative injuries to feline red blood cells (Heinz Body formation ) as well as facial oedema, generalized malaise, anorexia and diarrhoea [60]. Recovery times were also increased following the second consecutive day of administration. A study performed 12 years later, in cats repeatedly anesthetised with propofol and undergoing radiotherapy, did not report any relevant hematologic changes [142]. While recovery times were not recorded, it was believed that they were not increased. The authors reported similar quality of recovery in all cases without any of the side-effects reported in the Andress study (anorexia and malaises).
Equine
Due to the lack of analgesic effect of propofol, the doses required to produce surgical anesthesia are such that while the CV function is more or less well maintained, respiratory depression is of concern and oxygen support is recommended as well as positive pressure ventilation [5, 31, 36, 143, 144].
As far as the author is aware, propofol is the only IV anesthetic which, thanks to its pharmacokinetic quality, is sufficiently non-cumulative to be used in the equine patient for procedures lasting longer than 2 h [73, 145]. Although the induction of anesthesia with propofol may be of poor quality, recoveries are usually good and smooth [116, 146]. In unpremedicated horses, a 4 mg/kg IV dose of propofol is necessary to induce anesthesia [116]. As propofol exists as a 1 % solution, that brings the volume of propofol for induction of anesthesia for a 500 kg horse to about 2000 mg or about 200 mL, which is a big and expensive volume. This and the unreliable quality of induction necessitate combinations of different drugs with propofol such as xylazine or detomidine but with little improvement in the quality of induction [26, 36, 116]. The best results so far seems to have been obtained with the addition of guaiphenesin (GGE) , an IV centrally acting muscle relaxant (74–100 mg/kg), or ketamine in the induction protocol or medetomidine in the premedication [37, 73, 147, 148]. Other authors have even recommended anaesthetising horses using a ketamine based protocol followed once anesthesia is induced, by a bolus of propofol and propofol TIVA [28, 31].
In two different studies, following premedication with medetomidine (7 μg/kg IV) and induction of anesthesia with propofol IV 2 mg/kg, anesthesia was maintained up to 4 h at an infusion rate between 0.07–0.11 mg/kg/min and 3.5 μg/kg/h for propofol and medetomidine, respectively [72, 73]. In both cases, although all the horses were breathing spontaneously, the rhythm was irregular in the majority of the horses, no apnea was noticed and PaCO2 did not change significantly, while hypoxemia was recorded in some ponies with PaO2 values as low as 35 mmHg [72]. In terms of CV parameters, those were relatively well maintained with a few cases of mild hypotension recorded in some ponies with the lowest value recorded for SAP of 78 mmHg [73]. Recoveries were smooth and of good quality with fewer attempts at standing and better overall recovery score when atipamezole (specific (dex)medetomidine reversal agent) was not used. The recovery time varied between 20 and 38 min between the two studies and was not significantly affected by the addition of atipamezole .
In a similar study to the previous one the replacement of propofol with ketamine to induce anesthesia allowed for better induction quality with more difficult intubation and very similar cardiopulmonary parameters. Following 4 h of anesthesia, the mean recovery time was of 31.1 min with a quality scored of good to excellent [149].
The addition of diazepam (0.02 mg/kg, IV) to the previous protocol in 50 client owned horses undergoing different surgeries, provided excellent induction and endotracheal intubation conditions [28]. With a similar medetomidine CRI, but a propofol variable rate infusion (VRI) (0.098–0.108 mg/kg/min) CV parameters were well maintained (no vasopressor agents required) but still 23 horses needed IPPV. While the anesthesia time varied between 46 and 225 min, the recoveries were reported as been uneventful with a mean recovery time of 42.2 min.
When adding GGE (100 mg/kg) to a similar protocol (medetomidine 5 μg/kg and propofol 3 mg/kg, IV) to induce anesthesia in seven horses, Oku et al. recorded better quality of anesthesia and considered 3 μg/kg/min of medetomidine and 1 mg/kg/min of propofol as the minimum infusion rate (MIR) required to maintain anesthesia in horses undergoing castration [148]. Spontaneous breathing was maintained but one horse was apneic at 10 and 15 min and two others at 35 min after receiving a bolus of propofol . Cardiovascular parameters were well maintained and recoveries were of fair (five horses) good (one horse) and excellent (one horse) quality. The number of attempts at standing varied from one to five, with a mean recovery time of about 44 min.
Similarly to other species (dogs and cats), co-infusion of ketamine (20–50 μg/kg/min) allows for a decrease of propofol infusion rate while maintaining good cardiopulmonary parameters [31, 32, 150].
In Flaherty’s study , the protocol consisted of detomidine (20 μg/kg IV) premedication followed by induction with ketamine 2.2 mg/kg IV and maintenance of anesthesia with ketamine 40 μg/kg/min and propofol 0.12 mg/kg/min (preceded with a 0.5 mg/kg, IV) in four ponies [31]. Following a propofol infusion lasting 65 min (ketamine 60 min) the recovery was of good quality and lasted about 27.2 min.
Ohta’s et al., premedicated horses with 1 mg/kg of xylazine, but used a propofol based IV induction (3 mg/kg) with midazolam (0.05 mg/kg) which resulted in unacceptable induction conditions with some horses paddling [32]. Thereafter, they maintained anesthesia with a ketamine and propofol infusion rate of 50 μg/kg/min and 0.16 mg/kg min, respectively. Ketamine was stopped 20 min before the estimated end of the surgery. While propofol was stopped 112–140 min after the start of the infusion, recoveries were of good quality and lasted about 70 (±23) minutes.
Ketamine (2 mg/kg) and diazepam (0.03 mg/kg) IV were used to induce acepromazine (0.03 mg/kg, IV) and detomidine (0.015 mg/kg, IV) premedicated horses [150]. Induction of anesthesia was then followed by a propofol bolus of 0.3 mg/kg and anesthesia was maintained with infusions of propofol (0.16 mg/kg/min) and ketamine (20–40 μg/kg/min). The ketamine infusion was started at a rate of 40 μg/kg/min and was decreased by 10 μg/kg/min every 20 min. It was terminated 30 min before the estimated end of anesthesia. After a mean anesthesia time of 59 ± 17 min, recovery time was 34 ± 7 min and of good quality.
The addition of medetomidine infusion to ketamine/propofol anesthesia was studied by Umar et al. [37, 144, 151]. The basic protocol was the same between the three studies: premedication with medetomidine 5 μg/kg, IV followed by induction of anesthesia with midazolam 0.04 mg/kg and ketamine 2.5 mg/kg IV. To maintain anesthesia, ketamine at 1 mg/kg/h and medetomidine 1.25 μg/kg/h was added to a propofol VRI of 0.14 mg/kg/min (2006 and 2007 studies) and 0.17–0.13 mg/kg/min (2015 study). In all the studies the CV parameters were very well maintained. In the first study (2006), apnea was observed in all horses (six) after the propofol loading dose and therefore IPPV was initiated in this study as well as the following two studies. Once IPPV was started, no respiratory issues were observed in any of the studies. Duration of anesthesia was 115 ± 17 min, 4 h and 175 ± 14 min in the 2006, 2007 and 2015 study, respectively. Recovery quality was good in all the studies, with a time to standing of 62 ± 10, 98 ± 21 and 74 ± 28 min, respectively.
Ruminants
Very little information is available on the use of propofol based TIVA in cattle. In 2015, Deschk et al. reported the use of propofol in unsedated calves (6–12 months old) for induction (5 mg/kg IV) and maintenance (0.6 and 0.8 mg/kg/min for 60 min) of anesthesia [38]. The calves were allowed to breathe room air. Recoveries were uneventful. No apnea was noticed at any time while some muscle tremors were observed in three out of eight calves at a propofol infusion rate of 0.6 mg/kg/min but in only one out of eight at a propofol infusion rate of 0.8 mg/kg/min. The arterial pressures decreased in both groups but to a greater extent in the 0.8 mg/kg/min group which also had a bigger decrease in SVRI (systemic vascular resistance index). Cardiac index (CI) increased in the 0.8 mg/kg/min group while staying constant in the 0.6 mg/kg/min group.
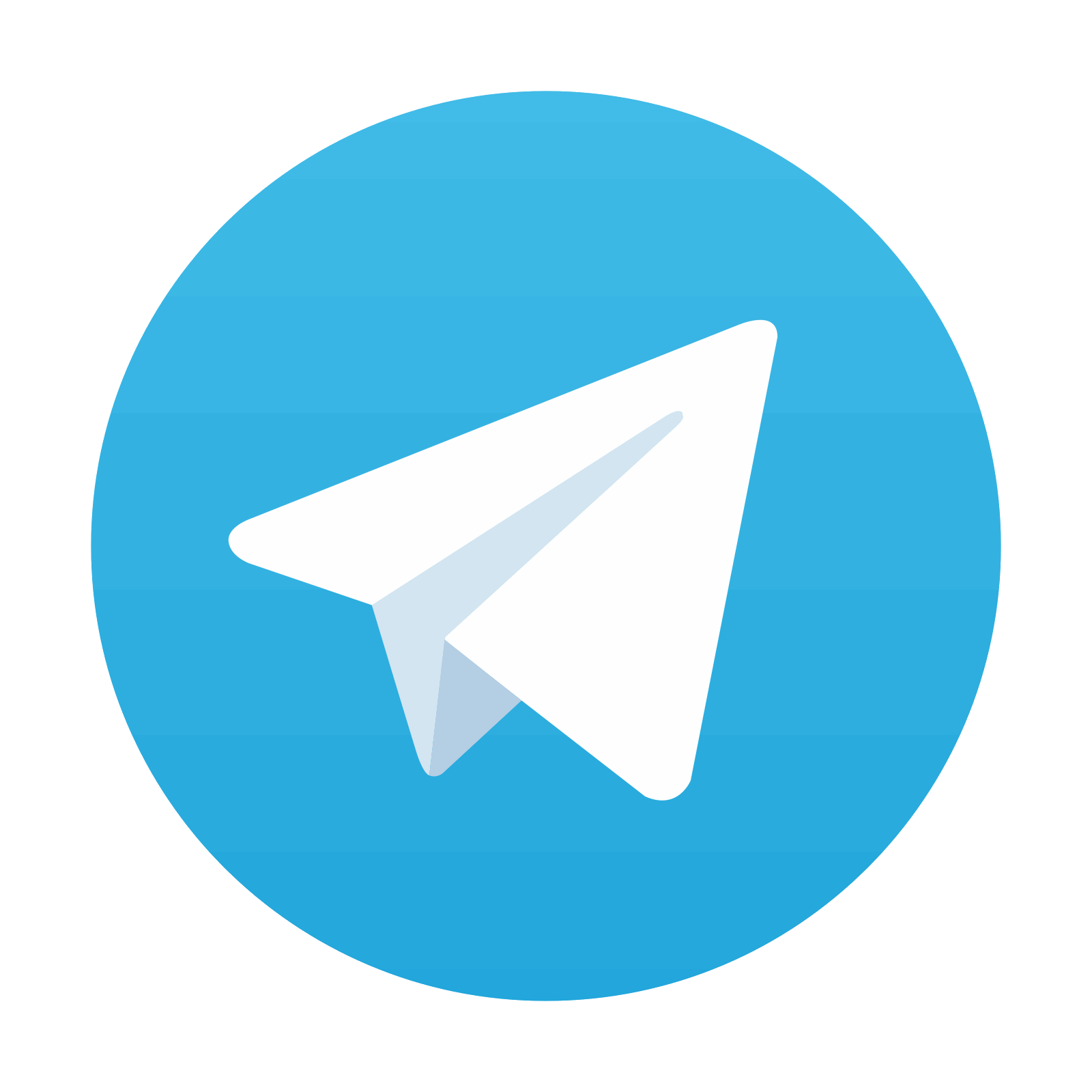
Stay updated, free articles. Join our Telegram channel

Full access? Get Clinical Tree
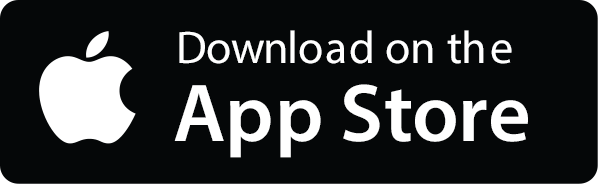
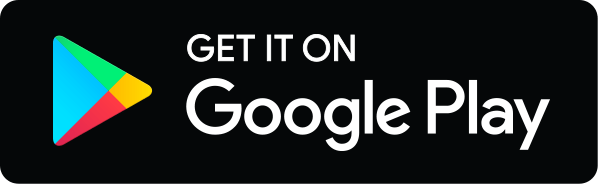
