Fig. 18.1
Oscillating blood pressure in a real patient with a frequency of about 1 cycle every 6 min. Highly concentrated norepinephrine and epinephrine solutions (200 μg/ml) were being infused by syringe driving pumps, while a volumetric pump provided a carrier infusion. Oscillations in the carrier fluid flow rate caused oscillations in vasopressor administration
For the delivery of intravenous anaesthetic drugs, predominantly syringe pumps are used as over a large range of infusion rates the accuracy is considered to be adequate. But syringe-driven infusion pumps also have their disadvantages. Several conditions can cause an infusion device not to deliver the expected infusion rate and volume.
The source of these deviations can be the pump, the syringe and infusion setup or the user and his interaction with the infusion device.
The Syringe Pump
Basic components of an infusion pumps are the electrical motor, one or more displacement sensors and a pressure sensor. A sensor will measure the movement of the plunger of the installed syringe. Speed of the motor is controlled by electronic circuitry that uses this sensor output for feedback. In the past, analogue circuits were used. Today most controlling circuits will contain microprocessors which highly increase the accuracy. Feedback mechanisms need time to optimise the control and hence will create fluctuations around the set point. The lower the infusion rate and the shorter the time of measurement or observation, the higher the deviation of set infusion rate will be. In order to assess the final error in the infusion rate, trumpet curves are constructed [Fig. 18.2] that show the percentage error related to the time of observation of measurement. When the observation window enlarges, the effect of the fluctuation is damped out and the bias relative to the set rate of the pump becomes apparent. The trumpet curve is in essence a statistical analysis of the error of the pump over time and belongs to one particular flow rate. To get a good impression of the infusion pump, trumpet curves at different flow rates should be judged, dependent on the clinical use of the infusion pump. Modern infusion pumps with microprocessor control can be so accurate that the trumpet curves almost become irrelevant. Trumpet curves do not reflect the startup behaviour of the device and possible under or overshoot.
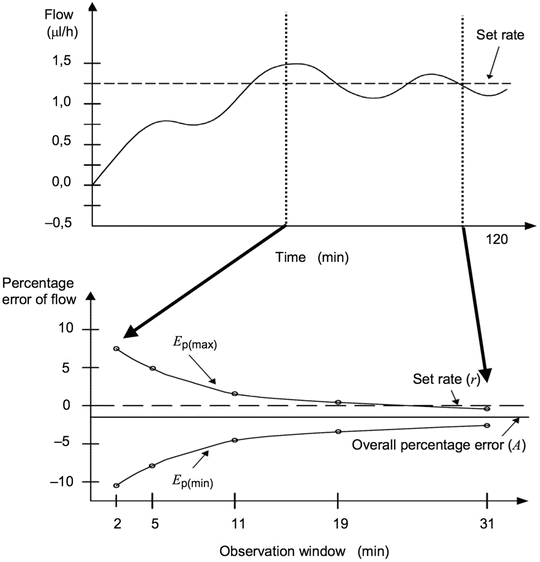
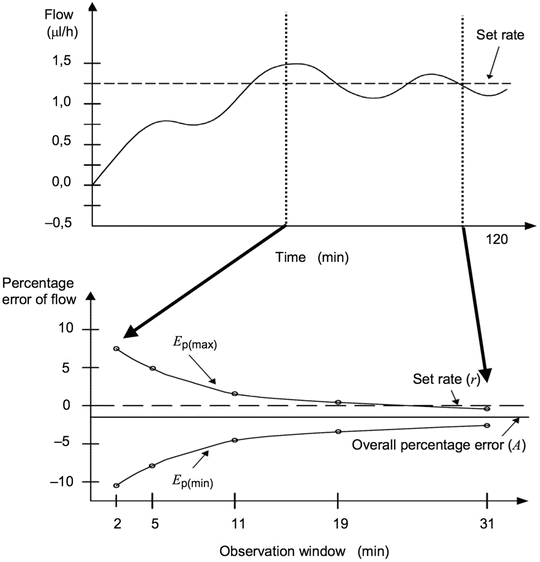
Fig. 18.2
Typical results from testing conventional rate-controllable syringe driver pumps. Fluctuations around the mean infusion rate are caused by positive and negative feedback of the control mechanism, giving the typical funnel shape curve after several repeated tests. Usually the observation window starts when the output is stable and therefore does not give information on the startup performance
For intravenous anaesthesia, flow rates from 1 to 1000 ml/h are necessary, but requirements on a neonatology department will differ from an adult department and may require accurate flow rates less than 1 ml/h. Not only mechanical parts like gear boxes but also electronic components like capacitors will wear out over time. This may influence the accuracy of the infusion pump, and it is a good practice to check infusion pumps on a scheduled time base.
Pumps used in Europe must comply with CE (Conformitée Européene) marking. For CE-marked pumps, the measurements of flow rate accuracy conform to standards developed by the International Electrotechnical Commission and are described in standard IEC 60601-2-24. There are more standards issued by the IEC that concern the electrical safety and reliability of infusion pumps that are both adopted by the CE marking and the federal agency for Food and Drug Administration (FDA).
For target-controlled infusion, no specific standards exist yet. The aforementioned trumpet curves are not sufficient to determine the accuracy of the target-controlled infusion pumps as the impact of rate deviations on the calculated concentrations is difficult to predict and are dependent on the characteristics of the applied pharmacokinetic model. Furthermore, adequate startup performance is important for target-controlled infusion and effect-controlled infusion as this influences the loading of the central volume of the pharmacokinetic model. Trumpet curves do not describe the startup performance.
During the first commercialisation of target-controlled infusion pumps, Astra Zeneca sold a separate microprocessor module (Diprifusor® ) that could be implemented in existing infusion pumps for the target-controlled infusion of propofol [2]. Accuracy and precision of the combination of module and pump was guarded by standards and testing requirements, issued internally by the manufacturer of the module (personal communication). The performance of the module-pump combination was confirmed in laboratory settings [3].
The coming of the so-called open TCI devices has opened the possibility of implementing more drugs and models but also opened the door for other errors.
The pharmacokinetic calculations may be erroneous or based on inappropriate parameters, but also the interplay between the computational software layer that handles the pharmacokinetic calculations and the layer that handles the flow rate control can influence the performance of the TCI system [4, 5].
There are a multitude of pharmacokinetic models in use these days. For some drugs, the users of target-controlled infusion pumps even have more than one model to choose from. Even pharmacokinetic data coming from the same publication can have a different implementation of the pharmacokinetic model due to different interpretation of the data by the pump manufacturer (see target-controlled infusion).
Compartmental models have an inherent problem of being unable to correctly predict the early phase in fast changing concentrations because the model assumes immediate mixing of the drug in the compartments [6]. The discussion of the most appropriate model for propofol still continues. It is however important to emphasise that from a clinical point of view, only the predictability of the drug effect, which is the result of the correctly chosen dose and not the drug’s theoretical blood concentration, is important [7].
The electronic circuitry in modern infusion pumps mostly consist of an embedded microprocessor. Together with the improved sensor technology, this allows for a much better accuracy of the mechanical performance of the syringe-based infusion pump. Unless not appropriately programmed, the biggest deviation from the set infusion rate, when controlled manually, is not to be expected from the mechanical and electronic controlling part of the device but from external equipment like the syringe and infusion lines.
The Syringe
The syringe can be an important source of deviation from the intended amount of fluid or drug delivery.
First of all, syringes are not standardised in sizes, even if the volume indication of different brands is the same. Syringe infusion pumps, unlike volumetric pumps, do not really measure the flow of the fluid but rely on the measurement of the plunger displacement to calculate the flow rate. Usually, but not always, all syringes in a hospital are of the same brand, and the settings in the infusion pumps have to be adjusted to this specific brand. The automatic recognition of different syringe sizes by the infusion pump is not always reliable especially when differences in diameter are small like in the case of 20 and 30 ml syringes. When the procurement department of a hospital changes syringe supplier, all pumps have to be reprogrammed. It is not unlikely that in such a case not all pumps are updated because infusion pumps may travel a lot between departments.
An even more frequent source of error is when a patient is transferred from one hospital to another where another brand of syringes is used. Not only the syringe may be taken over, occasionally also the infusion device itself with wrong syringe settings is ‘assimilated’ in the bulk of available infusion devices if the make and type is similar. Usually the medication is checked when a patient enters a hospital. Making a note of the (infusion) devices connected to the patient and the syringes in use should also be a part of the standard check procedure when a patient is transferred, thereby increasing the awareness for problems that may arise from exchanging syringes and equipment.
Differences in syringe brands may not be limited to size. Compliance of syringe and plunger will depend on the manufacturer and also on issues like lubrification of the inside of the syringe plunger to prevent ‘sticky’ syringe, which will play a role in the performance of syringe-pump combination [8].
Fitting of the Syringe
Because infusion pumps will have to accommodate different syringes with different sizes, the fitting of the flange of the syringe and the plunger is often somewhat loose. This is especially true for pumps that have no automatic syringe loading. An easy way of assessing the amount of fluid involved in the play in the syringe flange fitting is to put the infusion pump in pressure display mode and press some fluid retrogradely into the syringe via a stopcock at the end of the syringe. The amount measured is also dependent on the compliance of the syringe. Deformation of the rubber plunger is for a large part responsible for the initial low stiffness of the syringe, allowing for about 1–2 ml of extra fluid to enter a syringe of 50 ml without substantial increase in pressure. With higher pressures, the syringe may move a little bit if the part where the flange of the syringe is fitted is flexible. Using this simple test, the consequence of air in the syringe can also be demonstrated.
Air in the Syringe
Air in the syringe can have great consequences for the reliability of the infusion and alarm functioning [9]. Air is compressible, as opposed to fluids. This will have an impact when the pressure in the syringe changes due to obstruction or change of height of pump position. Usually occlusion alarms are set around 100 kPa (1 Bar). This is about twice the atmospheric pressure, so according to Boyle’s law the volume of the air bubble will be halved at the time the pressure inside the syringe will be at the level that triggers the alarm. For an air bubble of 1 ml, and an infusion rate of 1 ml/h, it will take 30 min before an occlusion is noticed. At 10 ml/h, the delay is still 3 min [Fig. 18.3]. To be added to this is the volume extension that occurs because of the compliance of the whole infusion system and pump which can be in the range of 1–2 ml. It is important always deair a syringe. This has to be done just before connecting the syringe and infusion line to the patient. If there are multiple small air bubbles, the initial visual inspection may not reveal much air but after light tapping these bubbles will merge into one large space of air. Not only for intravenous anaesthetic drugs but for all highly concentrated drugs in syringe-driven infusion pumps, uninterrupted flow is essential.
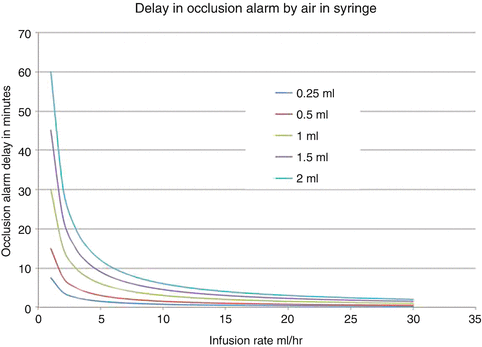
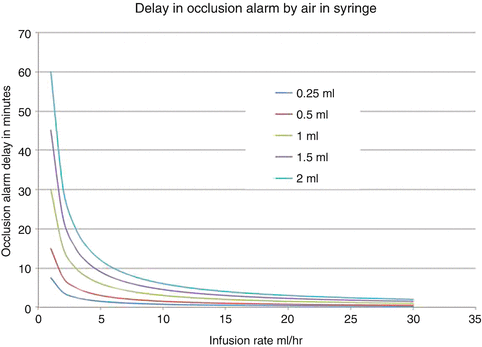
Fig. 18.3
Effect of air in the syringe on delay in activation of occlusion alarm set at 1 bar. Usually at least 1 ml must be added for the compliance in plunger and play of the syringe fitting in the case of a 50 ml syringe
With some exceptions where the pressure inside the syringe is directly measured in the infusion line, most syringe-driven pumps derive the pressure from the force on the piston or the plunger of the syringe. The relation between this force and the actual pressure inside the syringe is dependent on the size of the surface of the plunger. A 50 ml syringe has a radius of about 30 mm, whereas a 20 ml syringe has a radius of about 20 mm. The surfaces of these plungers are 28.3 cm2 and 12.5 cm2 (πr2), respectively. To obtain the same pressure in the syringes, the force has to be reduced for the 20 ml syringe to 0.44 of the force of the 50 ml syringe. Or in other words, if not adjusted, the level of the pressure alarm would be more than doubled in the 20 ml syringe.
Syringe pumps reduce the force that will activate the high pressure alarm, usually according to this relationship of the plunger areas. The problem is that the force is not only used for building the pressure inside the syringe but also to overcome the resistance of the friction of the plunger inside the syringe. This friction is related the circumference of the plunger which is 9.4 cm and 6.3 cm, respectively (2πr), and hence the part of the force responsible for overcoming the friction should be only reduced to 0.67 of the value for a 50 ml syringe. As the friction is unknown and cannot be taken into the adjustment, smaller syringes tend to alarm on overpressure much earlier than bigger syringes when the pressure alarm limits are set sensitive and may even tend to give false alarms on line occlusion. This should however not influence the decision to select the appropriate syringe for clinical circumstances such as paediatric intravenous anaesthesia, as compliance of the smaller syringes is better and amount of undissolved air in the syringe is less.
Positioning of the Pump
It is clear that pressure changes inside the syringe will cause fluctuations in the flow of fluid towards the patient. As explained above, the easy compressibility of the rubber part of the plunger together with residual air is mainly responsible. Pressure changes can result from obstruction of the flow but also may be caused by a change in the height of the infusion pump in respect to the level of the patient [10]. Accurate measurements show that lowering and lifting of the pump even over relative small distances will influence the constant flow rate of the infusion. When the position of the syringe pump is 80 cm below the patient, a pressure of 80 cm H2O will exist inside the syringe. When the position is changed to 80 cm above the patient, the pressure will change to negative: −80 cm water. It is inevitable that some fluid will spontaneously flow into the patient with an amount that is dependent on all the above-mentioned factors: play in the mechanics of the pump, fitting of the syringe, compliance of syringe and plunger and the existence of air. If the plunger is not retained by the pump, the negative pressure will force the syringe to empty itself. If that happens, then once the initial resistance of the plunger has been overcome, a 50 ml syringe will empty itself in a view minutes. This process is called syphoning or free flow and can be prevented by putting an anti-syphoning valve in the infusion line [11]. This valve will only open at a certain overpressure, thereby preventing this free flow. Syphoning is a dangerous situation that can easily occur in the hectic environment of a surgical procedure or in the emergency room. This can happen if, for example, someone takes the syringe out of an infusion pump and puts it on top of a monitor or if the syringe is not properly placed in the pump because the contents of the syringe are temporarily not required and pump and syringe are put in an elevated position relative to the patient.
When there is a possibility of air entering the syringe, for example, by cracks or a not appropriately closed stopcock, the level of the pump only needs to be a few centimetres above the venous pressure to allow drug to free flow into the patient as no friction of the plunger will play a role in such a situation. Although these possible deleterious situations must have occurred numerous times in emergency rooms, intensive care units, operation theatres and other places in the hospital where patients are transported while being connected to a syringe infusion pumps, very little is known on the real incidence, and reports are incidental [12]. Closing the stopcock when an infusion is temporarily not required should be a habit of all care providers.
The opposite of syphoning is back flow into the syringe. This can happen when the pump is positioned lower than the patient or when more pumps are connected to a manifold. When an infusion line is occluded downstream from connected infusion pumps, then contents from the infusion system with the lowest compliance will flow into the infusion system with the highest compliance until the pressure rise is large enough to trigger the occlusion alarm in one of the connected devices. This can be prevented by one-way valves in the infusion line [13]. One-way valves should definitively be used when a gravity infusion is part of a multi-infusion setup. Not doing so will effectively remove the occlusion alarm from the infusion pumps. If no one-way valves are used in the individual infusion lines, then one must realise that in case of a distal occlusion, the delay in the occurrence of the pressure alarm will be related to the sum of all the volumes of the high compliance parts and air in the individual syringes.
Dead Space
Dead space in infusion systems is by far the most unpredictable cause of flow change when infusion pumps and infusion lines are connected to one single entry point into the patient [14]. Dependent on the bore and length of an infusion line, dead spaces of 5 ml or more can easily be created if extension lines are placed between the point where infusion lines come together and the venous cannula. In particular, when a gravity infusion is used as a carrier, such a dead space of 5 ml may create dangerous fluctuations in drug concentrations and will disturb the objective of a targeted-controlled infusion system when used. Especially in the early phase of an anaesthetic procedure, for most drugs unintentional interruption of drug administration will cause a much faster decrement in blood concentration than when the drug has been administered for hours (context-sensitive decrement time).
Another complicating factor at the start of an anaesthetic procedure is the fact that individual pharmacokinetic and pharmacodynamic properties of the patient are initially unknown while there is a great change required in the patients’ conscious and anaesthetic state to cope with another unknown factor: the surgical stimulation of the patient. Based on monitored patient data, the anaesthesiologist will try to get an idea of the sensitivity of the patient for the administered drugs in relation to the applied stimulus. Unnoticed changes in the drug flow caused by dead space and the other above-mentioned phenomena will disturb this feedback process and may lead to over or under drug dosing not only immediately but also later on.
Drug infusion is not only about the physics of flow, compliance and dead space [15]. Pharmacodynamic interactions may exaggerate the influence of the above-mentioned phenomena especially when high drug concentrations are used. Therefore, even intentional changes in drug administration like the change of a target in TCI may lead to unintended changes in the anaesthetic state when these high drug concentrations are used in the presence of dead space [Fig. 18.4].
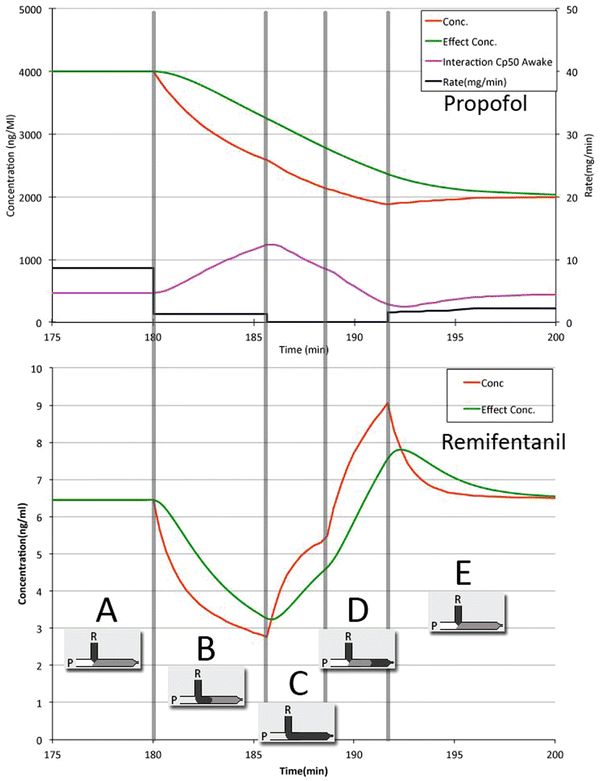
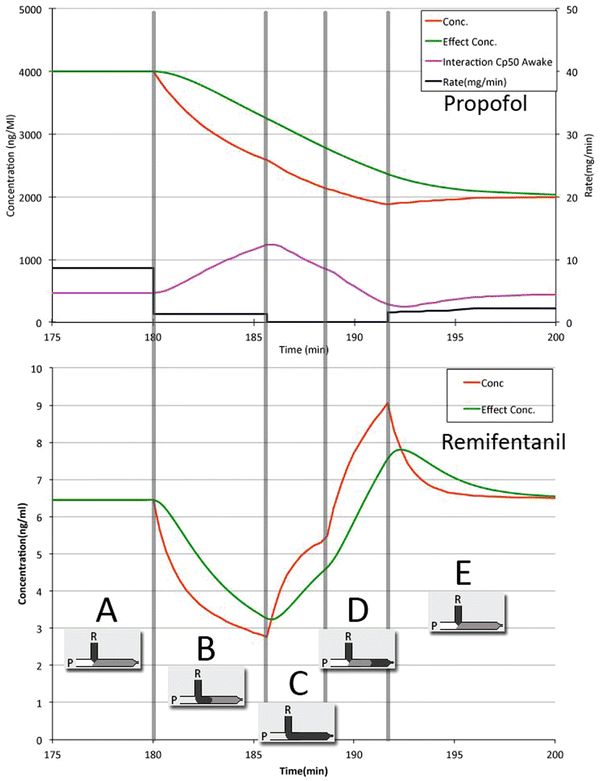
Fig. 18.4
Effect of dead space and high drug concentration [33]. Propofol (P) TCI 10 mg/ml target 4 μg/ml after 3 h changed to 2 μg/ml. Remifentanil (R) 100 μg/ml fixed rate at 0.25 μg/kg/min. Dead space (DP) volume: 1 ml. No mixing inside DP assumed [14]. A. Mixture of propofol remifentanil runs into the patient—at 180 min P target is changed from 4 μg/ ml to 2 μg/ml: TCI pump stops. B. Flow in DP equals flow R. Existing mixture is pushed out slowly; R and P concentrations drop. Probability of wakening up rises. A small amount of propofol is still delivered. C. Mixture does not contain P anymore. Concentration R rises. D. P reaches new target of 2 μg/ml. TCI pump starts again. No propofol delivered because of DP. R is flushed by P, causing concentration to overshoot. E. DP filled by mixture again. R concentration stabilises to initial concentration
Practical Advice
For intravenous anaesthesia, use dedicated infusion systems with low dead space and one-way valves in each individual line to avoid possible problems with dead space and occlusion alarm delays.
Use the lowest concentrations of IV drugs that are clinically acceptable.
Contamination
The success and increasing use over time of intravenous anaesthesia and TCI is for a large part attributable to propofol . Propofol has an advantageous pharmacokinetic and pharmacodynamic profile, but it also has its disadvantages. With respect to the equipment and handling, probably the biggest disadvantage is the ease of contamination. For this reason, some manufacturers offer propofol in pre-filled syringes, and/or preservatives have been added. Originally, ethylenediaminetetraacetic acid (EDTA ) was used, but other manufacturers used sulphite for marketing reasons. The latter has been withdrawn because of predictable bronchospasm problems that sulphite caused in patients that had a history of heavy smoking [16].
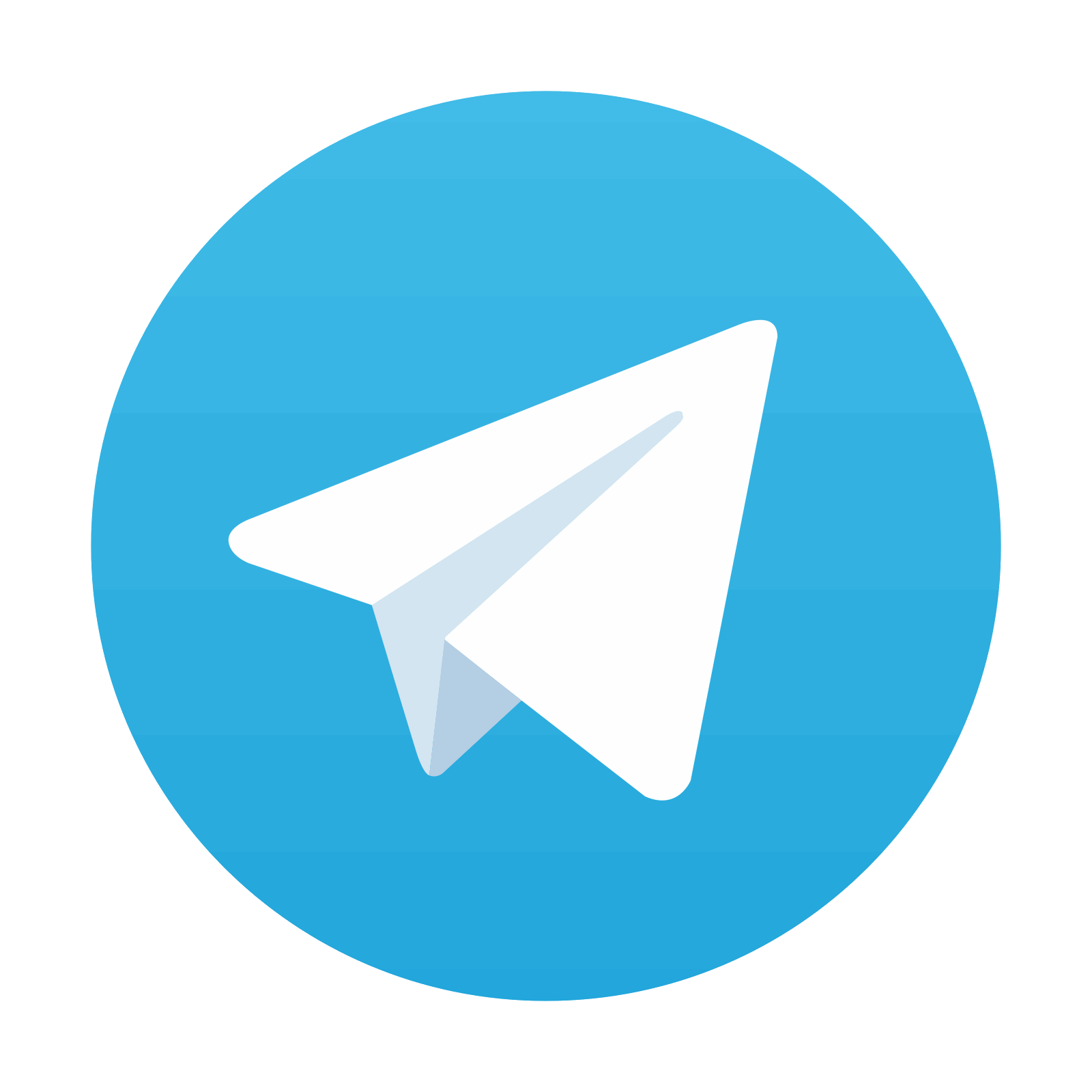
Stay updated, free articles. Join our Telegram channel

Full access? Get Clinical Tree
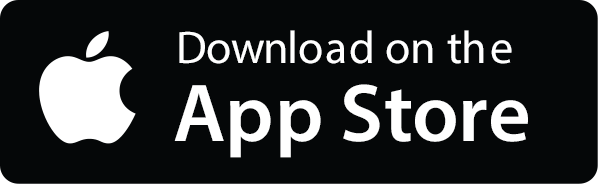
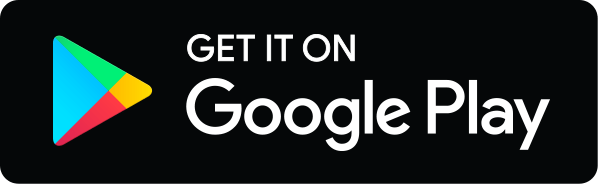