Chapter 80 Thrombosis in Pediatric Intensive Care
This chapter describes key issues related to thrombosis in the pediatric intensive care unit. Developmental hemostasis is a crucial concept both to the understanding of the etiology of thrombosis in children, and to the application of diagnostic and therapeutic strategies. The etiology, epidemiology, clinical features, diagnosis, and management of the major types of thrombosis encountered in children in the intensive care unit (ICU) will be discussed. However, thrombosis of the central nervous system (CNS), including arterial ischemic stroke and cerebral sinovenous thrombosis are beyond the scope of this chapter (see Chapter 63). As in many areas of pediatrics, high-level evidence is often lacking; however, best-available evidence will be drawn upon where possible. Extrapolation from adult studies resulting in suboptimal treatment outcomes in children highlights the need for pediatric-specific trials and guidelines.
Developmental Hemostasis
The hemostatic system is a dynamic, evolving entity that not only likely affects the frequency and natural history of thromboembolic disease in children, but also the response to therapeutic agents.1–5 The concept of developmental hemostasis was first coined in the late 1980s and is now uniformly accepted. Not only are the plasma levels of many individual coagulation proteins different in pediatric patients from adults, but the global functioning of the coagulation system appears to be quite different. In additional to quantitative differences, there is evidence (mostly from animal models) of qualitative differences in many coagulation proteins, especially in neonates.6,7 Finally, again from animal models, data would support significant differences in the antithrombotic properties of the blood vessel wall, with altered concentrations of active glycosaminoglycans.8,9 Although ongoing research in this area is desperately needed, current knowledge regarding the differences between adults and children in plasma proteins most likely to impact on anticoagulation therapy is as follows.
Plasma concentrations of antithrombin (AT) are physiologically low at birth (~0.50 U/mL) and do not increase to adult values until 3 months of age. Sick premature neonates frequently have plasma levels of AT of less than 0.30 U/mL. Fetal reference ranges are now available and show that AT levels range from 0.20 U/mL to 0.37 U/mL at gestational ages of 19 to 38 weeks. This likely has a profound effect on the action of heparin, whose antithrombotic activity is dependent on catalysis of AT to inactivate specific coagulation enzymes, in particular thrombin. Some studies suggest children in pediatric intensive care units have markedly reduced AT levels compared to age-matched controls, potentially further enhancing this effect (Figure 80-1). The capacity of plasmas from neonates to generate thrombin is both delayed and decreased compared to adults, and is similar to plasma from adults receiving therapeutic amounts of heparin.10 Following infancy, the capacity of plasmas to generate thrombin increases but remains approximately 25% less than for adults throughout childhood.11 Both an increased sensitivity and an increased resistance to unfractionated heparin’s anticoagulant activities have been reported in vitro in plasma from neonates. Increased sensitivity to unfractionated heparin is observed in systems based on assays dependent on thrombin generation (e.g., activated partial thromboplastin time [APTT]). The in vitro effects of unfractionated heparin (0.25 U/mL), on neonates, children, and adults were compared recently, and thrombin generation was delayed and reduced in children compared to adults, and virtually absent in neonates.12 Resistance to unfractionated heparin is observed in systems based on assays that measure the inhibition of exogenously added Factor Xa or thrombin and that are dependent on plasma concentrations of AT.
In vitro, thrombin generation is similar in adults and children at the same concentration of low-molecular-weight heparin (LMWH). However, at 0.25 U/mL LMWH, thrombin generation was delayed and reduced by approximately half in newborns compared to adults. These differences were matched by reductions in rates of prothrombin consumption.12
The vitamin K–dependent clotting factors are the most extensively studied group of factors in infants. Physiologically low levels of clotting factors (II, VII, IX, and X) were measured in infants who received vitamin K prophylaxis at birth. The levels of the vitamin K–dependent factors and the contact factors (Factors XI and XII, prekallikrein, and high-molecular-weight kininogen) gradually increase to values approaching adult levels by 6 months of life. For children receiving vitamin K antagonists, the capacity of their plasmas to generate thrombin is delayed and decreased by 25% compared to plasmas from adults with similar INRs.13
Whether the overall activity of the protein C/protein S system varies with age is unknown. However, at birth, plasma concentrations of protein C are very low, and they remain decreased during the first 6 months of life. Although total amounts of protein S are decreased at birth, functional activity is similar to that in the adult because protein S is completely present in the free, active form because of the absence of C4-binding protein.14,15 Furthermore, the interaction of protein S with activated protein C in newborn plasma may be regulated by the increased levels of α2-macroglobulin. Plasma concentrations of thrombomodulin are increased in early childhood and decrease to adult values by late teenage years. However, the influence of age on endothelial cell expression of thrombomodulin has not been determined.16
Total tissue factor pathway inhibitor (TFPI) levels in newborns are reported as being similar to levels in older children or adults. Free TFPI is reported as being significantly lower in newborns.17
Etiology and Epidemiology
While there has been much published recently on a variety of factors that may contribute to the etiology of thrombosis, perhaps the most useful concept for the clinician to understand remains Virchow’s triad18. Virchow’s triad recognizes that three factors are involved in the development of thrombosis, those being the blood vessel wall, the blood constituents, and blood flow19 (Figure 80-2). Patients in the pediatric ICU (PICU) often demonstrate abnormalities in one, two, or all of these factors, and in the consideration of each patient, it is important to determine which of these abnormalities may be predominant, as this can be a useful guide to therapy. For example, in a patient with a cardiac lesion, either primary or postsurgical, where there is extremely poor blood flow in one part of the cardiovascular system, the optimal management is to improve the blood flow. While anticoagulation may well have an important role, progressively increasing the intensity of anticoagulation will significantly increase the risk of bleeding and yet may not substantially further reduce the risk of thrombosis, which is being driven primarily by flow. Alternatively, patients with disseminated intravascular coagulation (DIC) have a marked perturbation of function of the vascular endothelium (blood vessel wall) and anticoagulation alone is unlikely to prevent thrombotic complications, which will only be avoided by treatment of the primary illness and subsequent resolution of the DIC.
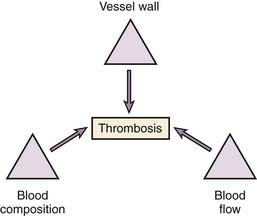
Figure 80–2 Virchow’s triad, highlighting the importance of each factor in contributing to thrombosis formation.
Table 80-1 summarizes the epidemiology and known risk factors for most etiologies of common non-CNS thrombosis seen in critically ill children. The most important risk factors for thrombosis in the pediatric intensive care unit remain the presence of vascular access devices and recent cardiac surgery. Two additional risk factors worth discussing, although they are not often particularly relevant in children are thrombophilias and heparin-induced thrombocytopenia.
Table 80–1 Summary of the Epidemiology, Clinical Features, Diagnosis, and Complications of the Most Common Types of Non-CNS Thromboembolism in Children
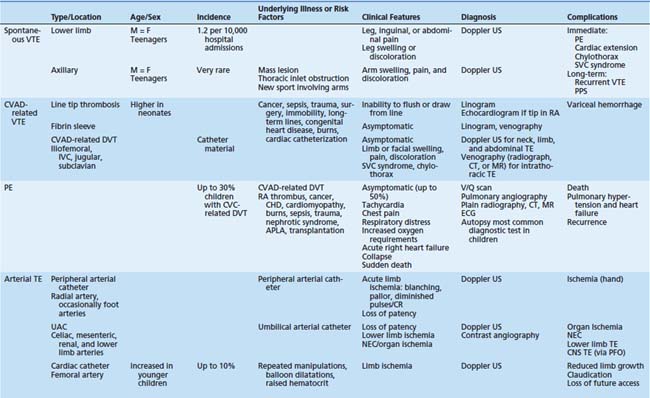
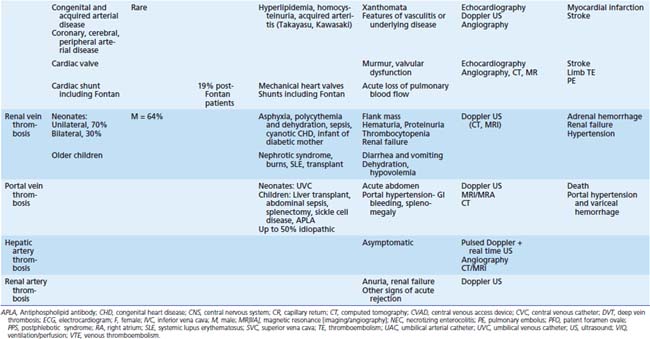
Central Venous Access Devices
The most common risk factor for venous thrombosis in children appears to be the presence of a central venous catheter (CVC) although most children have several concomitant risk factors.20
Over 50% of venous thromboembolisms in children occur in the upper venous system secondary to the use of central venous catheters (CVCs). Three types of CVC-related thrombosis are described in the literature: clots at the tips of CVCs that impair infusion or withdrawal of blood; fibrin sleeves that are not adherent to vessel walls but may occlude CVCs; and CVC-related thrombi that adhere to vessel walls, with partial or complete obstruction of vessels where the CVC is located. This discussion refers to this third type of thrombosis only; that is, obstructive CVC-related thrombosis. There are a number of mechanisms that may be contributory to the development of CVC-related thrombosis, including damage to the vessel wall by the CVC or by substances infused through the CVC (total parenteral nutrition [TPN], chemotherapy), disrupted blood flow due to the presence of the CVC, and thrombogenic catheter materials. Use of CVCs occurs most commonly in children who require short-term intensive care, hemodialysis, or long-term supportive care (TPN or chemotherapy).21
The incidence of CVC-related thrombosis reported in the literature varies, reflecting differences in underlying conditions, diagnostic tests, and index of suspicion. For example, the incidence of CVC-related thrombosis in children receiving long-term TPN varies from 1% based on clinical diagnosis,22 to 35% based on ventilation-perfusion scans or echocardiography, to 75% based on venography.23 In a prospective cohort, 18% of children in an intensive care setting with CVCs in place for at least 48 hours developed CVC-related thrombosis.24 The recently completed Prophylactic Antithrombin Replacement Therapy in Kids with ALL Treated with L-Asparaginase (PAARKA) study reported a venographically proven CVC-related thrombosis incidence of 37% among asymptomatic children with acute lymphocytic leukemia receiving L-asparaginase therapy,25 In many patient populations, the incidence is not accurately known.
Arterial Access
Adverse events occurring as a result of femoral artery puncture include arterial spasm and arterial thrombosis. Clinically, vascular spasm and thrombosis are indistinguishable in the initial phases of presentation with the following symptoms: decreased or absent pulses, pale or mottled limb, and decreased capillary refill. Arterial spasm usually resolves within a few hours in the absence of therapy while arterial thrombosis usually requires therapy. The incidence of femoral artery thrombosis following cardiac catheterization without thromboprophylaxis is approximately 40%. The incidence is inversely proportional to patient age and weight, with infants at highest risk.26 The frequency of femoral artery thrombosis in PICUs is unclear. Similarly with peripheral arterial catherization, the mechanism of injury is endothelial damage and blood vessel occlusion. The exact frequency of peripheral arterial occlusion in the PICU remains unknown.
Cardiac Surgery
Children with congenital heart disease constitute a major proportion of children seen in tertiary hospitals with thrombosis. Recent data shows that almost 50% of infants less than 6 months old and 30% of older children who suffer venous thromboembolic disease have underlying cardiac disorders. Similarly, almost 70% of infants (less than 6 months) and 30% of children who suffer arterial thrombosis have underlying cardiac defects. In addition, the majority of children receiving primary anticoagulant prophylaxis are being treated for complex congenital heart disease or severe acquired cardiac illness.26 Presumably, the mechanisms underlying this increased risk of thrombosis are alterations to blood flow (for example, following Fontan surgery, where venous return is driving the pulmonary blood flow), and disturbances of the vascular endothelium related to intravascular sutures or vascular manipulation. Whether a postsurgical inflammatory state induces changes in the blood constituents that predispose to thrombosis is also unknown. Two classic examples of cardiac surgery-related thrombosis are the Blalock Taussig (BT) shunt, and the Fontan procedure.
The natural history of BT shunts has been assessed using angiography. Godart et al.27 assessed BT shunt growth and development of stenosis and distortion in 78 patients at a mean follow-up time of 51 months. They found that growth of the pulmonary arteries occurred but did not exceed the normal growth of the pulmonary arterial tree. However, a shunt procedure could cause distortion and stenosis of the pulmonary artery that might have important implications for future corrective surgical intervention. Risk factors for patency and stenosis include the age of the patient and graft size.28,29
The incidence of thrombotic occlusion of BT shunts in the literature ranges from 1% to 17%.28–42 This risk might be increased in children having first-stage Norwood surgery, as these children are small and unstable and often have labile blood pressures as well.
There have been a number of recent reviews regarding anticoagulation after Fontan surgery.43,44 The Fontan procedure, or a modified version, is the definitive palliative surgical treatment for most congenital univentricular heart lesions. Thrombosis remains a major cause of early and late morbidity and mortality. Reported incidences of venous thrombosis and stroke ranged from 3% to 16% and 3% to 19%, respectively, in retrospective cohort studies where thrombosis was the primary outcome, and from 1% to 7% in retrospective studies assessing multiple outcomes. Thrombosis may occur anytime following Fontan procedures, but often presents months to years later. No predisposing factors have been identified with certainty, although this may be due to inadequate power and the retrospective nature of the studies.
Thrombophilia
Congenital thrombophilia is usually defined as having the following features: (1) positive family history, (2) early age of onset of thromboembolism, (3) recurrent disease, and (4) multiple or unusual thrombosis locations. Clinically, the most significant inherited prothrombotic conditions are deficiencies of antithrombin (AT), protein C (PC), and protein S (PS), because of the large increase in relative risk these deficiencies confer. Activated protein C resistance/factor V Leiden (FV-R506Q) and prothrombin G20210A (IIG20210A) polymorphism, while having less impact on individual risk, are significant because of their frequencies in certain populations. A large number of other candidate genes have been proposed as risk factors for congenital thrombophilia.45 However, most of these candidates have not undergone careful segregation or population studies to define their pathogenic role. In fact, some of the seemingly obvious candidates such as abnormalities in fibrinolysis do not appear to confer thrombotic risk.46 These latter studies are, however, hampered by the low prevalence of most of these inherited abnormalities in the general population.
Recent reports demonstrate an increased risk for thrombosis in families with a second genetic abnormality.47 Most reports have described a combination of FV-R506Q with abnormalities of PC, PS, or AT. These findings begin to shed light on the marked variability in clinical expression of these syndromes. The effect of more severe deficiencies has long been evident from the severely affected neonates with homozygous PC and PS deficiency. Apart from the well-defined homozygous cases, the risk and severity of thrombosis appear to vary with the type and number of underlying genetic abnormalities.47–50
The role of these congenital prothrombotic states in childhood thrombosis remains controversial. Despite recent meta-analysis suggesting that thrombophilia is a significant risk factor in childhood thrombosis, there are serious limitations to the published literature on which these meta-analyses are based. 51
The reported incidence of congenital prothrombotic disorders in children with venous thrombosis varies from less than 1% to over 60%.48–50,52–84 If one considers the deficiencies of AT, PC, and PS in addition to the Factor V Leiden and prothrombin gene mutations, large family studies found negligible rates of thrombosis in children less than 15 years.85 A number of cohort studies have failed to identify AT deficiency in children with both arterial and venous thrombosis.70,71,75,76 Those studies that reported higher frequencies of AT deficiency did not distinguish between acquired and inherited deficiency.57 In children with cancer and venous thrombosis, the reported incidences of thrombophilias are 3%.75,86 The variability in incidence reported in all these studies reflects small sample sizes, variability in study design, differing definitions of prothrombotic disorders, and different patient selection criteria.87 A prospective study of an unselected cohort of children with venous thrombosis found that with the exception of teenagers with spontaneous thrombosis, inherited thrombophilic markers did not contribute significantly to the pathogenesis of venous thrombosis in children.88
Screening for congenital prothrombotic disorders in children with venous thrombosis is of unproven benefit, regardless of the presence or absence of acquired risk factors. At this time, uniform screening of children with major illnesses, and/or those who require CVCs, for congenital prothrombotic disorders in order to provide prophylactic therapy cannot be recommended. The contribution of congenital prothrombotic disorders to venous thrombosis in pediatric patients remains to be clarified. Few children develop thrombosis due to a heterozygote congenital prothrombotic condition, without also having an acquired risk factor.45,68,69,89–96
Heparin-Induced Thrombocytopenia
Heparin-induced thrombocytopenia (HIT) occurs in approximately 3% to 5% of adults exposed to unfractionated heparin (UFH) and is typically associated with a reduced platelet count, occurrence 5 to 10 days after heparin exposure, and an increased risk of thrombosis despite the thrombocytopenia. HIT is the result of a complex antigen-antibody interaction, and the most important therapeutic intervention once HIT is diagnosed is the immediate withdrawal of all heparinoid anticoagulants, and substitution with non-heparinoid drugs until the risk of thrombosis is ameliorated.97
A number of case reports of pediatric HIT have described patients ranging in age from 3 months to 15 years.98–102 UFH exposure in these cases ranged from low-dose exposure during heparin flushes used in maintaining patency of venous access devices, to supratherapeutic doses given during cardiopulmonary bypass and hemodialysis. Studies specifically examining the frequency of HIT in children have varied in their reported results, likely related to differences in patient inclusion and laboratory techniques.103–108 Reported rates vary from almost nonexistent in unselected heparinized children106 up to 2.3% in children in the PICU.107 However, HIT appears to occur far less frequently in children than in adults and the rationale for this is unclear.106 Many patients in the neonatal intensive care unit/PICU who are exposed to UFH have multiple potential reasons for thrombocytopenia and/or thrombosis, and recent papers confirm that many positive HIT tests are in fact false positives.109 Danaparoid, hirudin, and argatroban are alternatives to UFH in children with HIT;100–102,110–113 however, these drugs have significant risks in children.114 Until such time as the true clinical incidence of HIT in children is understood, the diagnostic tests have higher sensitivity and specificity, and there are safe and reliable alternatives to heparin therapy in acutely sick children, the diagnosis of HIT in children should be made with caution. Careful attention to the diagnostic criteria, exclusion of other causes, and rationale use of test results are all required.
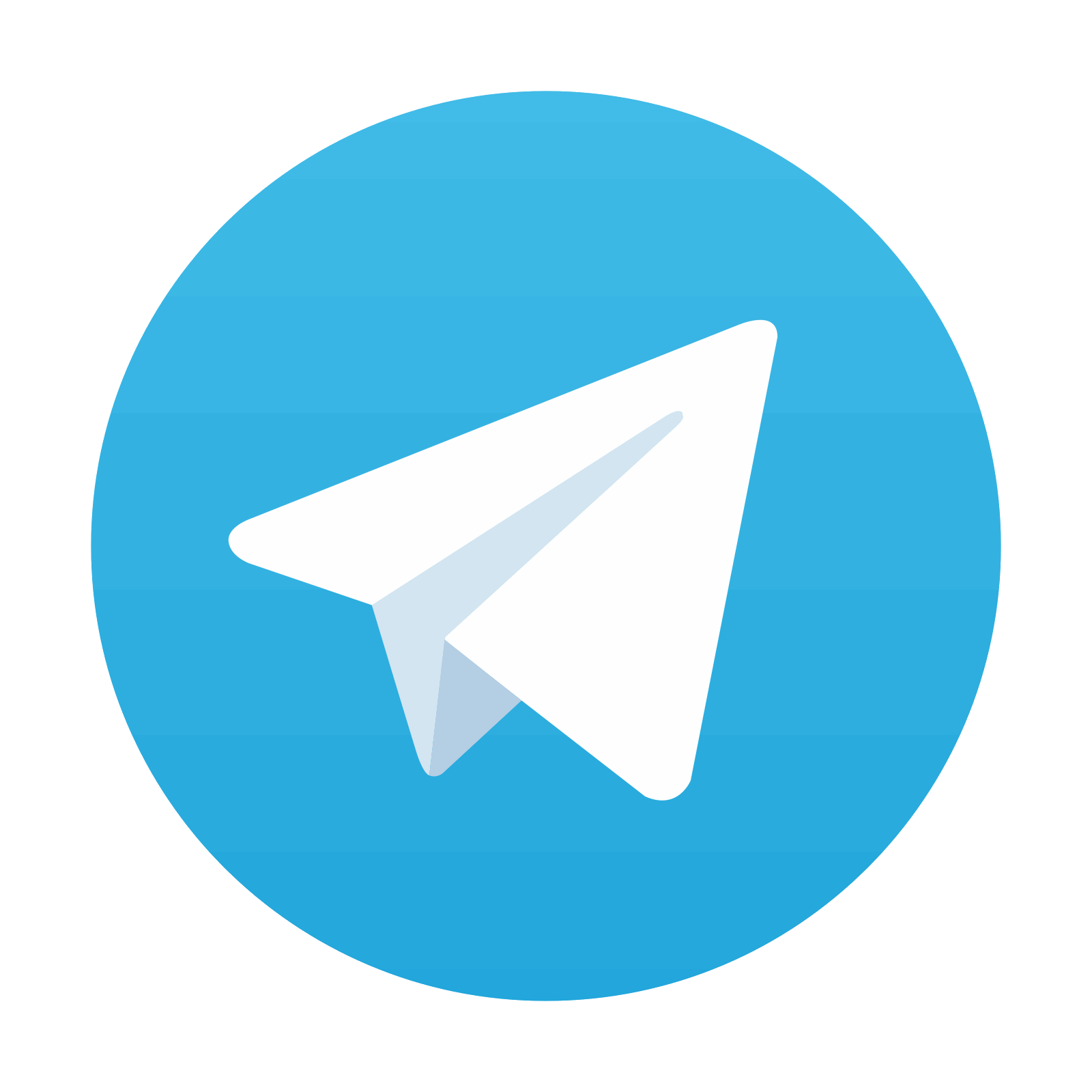
Stay updated, free articles. Join our Telegram channel

Full access? Get Clinical Tree
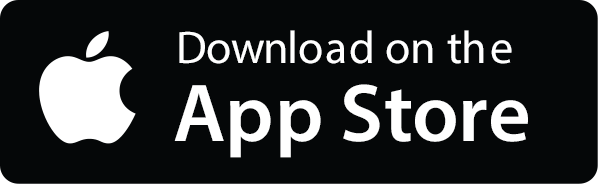
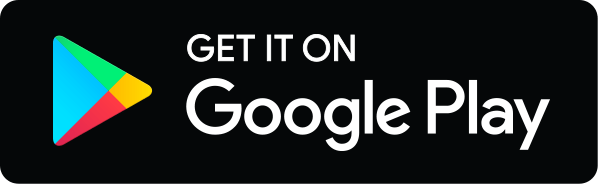