Despite exposure to wide fluctuations of environmental temperatures, human body temperature is maintained within a narrow range.16,120 Elevation or depression of body temperature occurs when (1) thermoregulatory mechanisms are overwhelmed by exposure to extremes of environmental heat or cold; (2) endogenous heat production is either inadequate, resulting in hypothermia, or exceeds the physiologic capacity for dissipation, resulting in hyperthermia; or (3) disease processes or xenobiotic effects interfere with normal thermoregulatory responses to heat or cold exposure.
Heat is transferred to or away from the body through radiation, conduction, convection, and evaporation. Radiation involves the transfer of heat from an object to the environment and from warm objects in the environment to another object, for example, from the sun to a body. Conduction involves the transfer of heat to solid or liquid media in direct contact with the body. Water immersion conducts significant amounts of heat away from the body. This effect facilitates cooling in a swimming pool on a hot summer day and hypothermia despite moderate ambient temperatures when an individual is wet on a rainy day. The amount of heat lost through conduction and radiation depends on the temperature gradient between the skin and its surroundings; cutaneous blood flow; and insulation such as subcutaneous fat, hair, clothing.134 In the respiratory tract, heat is lost by conduction to water vapor or gas. In animals unable to sweat, this represents the primary method of heat loss. The amount of heat lost through the respiratory tract depends on the temperature gradient between inspired air and the environment and the rate and depth of breathing.134 Convection is the transfer of heat to the air surrounding the body. Wind velocity and ambient air temperature are the major determinants of convective heat loss. Evaporation is the process of vaporization of water, such as that in sweat. Large amounts of heat are dissipated from the skin during this process, resulting in cooling. Ambient temperature, rate of sweating, air velocity, and relative humidity are important factors in determining how much heat is lost through evaporation. On a very hot and humid day, sweat pours off an exercising person, rather than evaporating and initiating heat loss. In very warm environments, thermal gradients are reversed, leading to transfer of heat to the body by radiation, conduction, or convection.143
In a human not affected by xenobiotics or disease, stimulation of peripheral and hypothalamic temperature-sensitive neurons results in autonomic, somatic, and behavioral responses that lead to the dissipation or conservation of heat. Thermoregulation is the complex physiologic process that serves to maintain hypothalamic temperature within a narrow range of 98.6 ± 0.8°F (37 ± 0.4°C), known as the set point.120 This hypothalamic set point is influenced by factors such as diurnal variation, the menstrual cycle, and others. Maintaining, raising, or lowering the set point results in many outwardly visible physiologic manifestations of thermoregulation such as sweating, shivering, flushing, or panting. In the central nervous system (CNS), thermosensitive neurons are located predominantly in the preoptic area of the anterior hypothalamus, but to a lesser extent in the posterior hypothalamus. These neurons are divided into those that are hot, cold sensitive, or temperature insensitive. Approximately 30% of preoptic neurons are warm sensitive. These increase their firing rate during warming and decrease their firing rate during cooling.28 Warming of the hypothalamus in conscious animals results in vasodilation, hyperventilation, salivation, and increases in evaporative water loss, as well as a reduction of cold-induced shivering and vasoconstriction.120 Cooling of the hypothalamus in conscious animals causes shivering, vasoconstriction, and increased metabolic rate even if the environment is hot.113 How these temperature-sensitive neurons of the hypothalamus detect temperature changes and affect neuronal transmission is unclear. Altered action potential initiation and propagation caused by temperature-dependent membrane potential changes are associated with the ratios of Na+ to Ca2+ that alter neuronal excitability and neurotransmitter release, or effect on the Na+,K+-ATPase (adenosine triphosphatase) pump.134 Xenobiotics that increase intracellular cyclic adenosine monophosphate (cAMP) concentrations increase the thermosensitivity of warm-sensitive neurons.28 In the brain stem, warm- and cold-sensitive neurons are located in the medullary reticular formation, where information from cutaneous receptors, spinal cord, and preoptic area of the anterior hypothalamus is integrated.125
The spinal cord is thermosensitive. Heat- and cold-sensitive ascending spinal impulses are conducted in the spinothalamic tract. As in the hypothalamus, local heating or cooling of the spinal cord results in thermoregulatory responses.120 In addition to the hypothalamus, brain stem, and spinal cord, there is evidence of thermosensitivity in the deep abdominal viscera.110,120,209 Intraabdominal heating or cooling results in thermoregulatory responses. Cold- and warm-sensitive afferent impulses are recorded from the splanchnic nerves in animals.110,211 Finally, the skin also contains heat and cold thermosensitive neurons. Whereas cold receptors are free nerve endings that protrude into the basal epidermis, warm-sensitive receptors protrude into the dermis.119,121 Cutaneous thermoreceptor output is affected by the absolute temperature of the skin, rate of temperature change, and area of stimulation.120 Cutaneous cold receptors are A-δ and C nociceptor afferent fibers. A-δ fibers are small-diameter, thinly myelinated fibers that conduct at 5 to 30 m/sec, and C fibers are small-diameter, unmyelinated fibers that conduct at 0.5 to 2 m/sec.115 Afferents from heat receptors are primarily C fibers. Cutaneous thermoreceptive neurons respond to external temperature change as well as rate of temperature change, sending early warning to the CNS via afferent impulses, allowing rapid and transient thermoregulatory responses before brain temperature changes (Fig. 29–1). It is important to emphasize that thermoregulatory responses as described above are affected by xenobiotics and disease processes.
Vasomotor responses to thermoregulatory input differ according to location. The normal thermoregulatory response to heat stress is mediated primarily by heat-sensitive neurons in the hypothalamus. In healthy individuals without impairment of vasodilatory mechanisms by xenobiotics, active vasodilation in the extremities occurs under noradrenergic control; increasing sympathetic stimulation results in vasoconstriction, and decreasing sympathetic stimulation results in vasodilation. Vasodilation in the head, trunk, and proximal limbs is not a result of decreased sympathetic tone; instead, it is a result of an active process involving the central nervous system and the peripheral cholinergic sudomotor nerves and the local effects of temperature on venomotor tone. Sweat glands release local transmitters, such as vasoactive intestinal polypeptide (VIP) or bradykinins, and vasodilation results. Areas of the body such as the forehead, where sweating is most prominent during heat stress, correspond to areas where active vasodilation is greatest. The neurotransmitters involved in the regulation of relationships between vasodilation and sweating as a response to heat stress are not fully elucidated in humans, but animal evidence suggests the presence of specific vasodilator nerves.120
Sweat glands are controlled by postganglionic nerve fibers, which are cholinergic, and large amounts of acetylcholinesterase as well as other peptides modulate neural transmission.125,126
The neurotransmitters involved in thermoregulation include serotonin, norepinephrine, acetylcholine, dopamine, prostaglandins, β-endorphins, and intrinsic hypothalamic peptides such as arginine vasopressin, adrenocorticotropic hormone (ACTH) thyrotropin-releasing hormone (TRH), and melanocyte-stimulating hormone (MSH).50,199 Studies on the effects of individual neurotransmitters in thermoregulation yield contradictory results, depending on the animal species and the route of administration of the exogenous neurotransmitter. However, epidemiologic studies demonstrate that patients taking xenobiotics affecting these neurotransmitters are at greater risk for heat illness. In the laboratory, refinements in techniques of microinjection of neurotransmitters into the hypothalamus of animals, rather than intraventricular instillation, have elucidated microanatomic sites where neurotransmitters are active. More research is needed, however, because interspecies variations and theoretical differences in response to exogenous versus endogenous peptides make this area of study complex. There is ample clinical and epidemiologic evidence that xenobiotics that affect the neurotransmitters listed above influence the ability to thermoregulate.
Apomorphine is a mixed dopamine agonist that causes hypothermia in animals; studies using selective D1– and D2-receptor agonists and antagonists demonstrate the hypothermic effect of apomorphine as a result of its effects on D2 receptors, with some modulation by D1 receptors in the hypothalamus.179 Stimulation of D2 receptors mediate the hypothermia induced by the peptide sauvagine.30 Stimulation of D3 by specific agonists caused hypothermia in an animal model.180,181 The link between dopamine D2 receptors and norepinephrine receptors in the hypothalamus leads to vasodilation and hypothermia. The effect of clozapine in producing hypothermia in rats is caused by D1 and D3 stimulation.180,220 Lesser-known peptides are also involved in thermoregulation. For example, neuropeptide Y is an amino acid neurotransmitter that occurs in high concentrations in the preoptic area of the anterior hypothalamus. Administration of neuropeptide Y caused a reduction in core temperature when administered with adrenergic receptor antagonists such as prazosin, an α1-adrenergic antagonist; propranolol, a β-adrenergic antagonist; and clonidine, a central α2-adrenergic agonist.84,218 Temperature regulation is complicated as both central and peripheral mechanisms are involved. The results of studies of temperature changes induced in animal models by the administration of xenobiotics frequently vary depending on the ambient temperature at which the experiment is performed, and on the species of animal. The α2-adrenergic agonist clonidine induced a dose-dependent significant increase in temperature of rats kept at high ambient temperature. This effect was mediated by the α2-adrenergic postsynaptic receptors. The administration of an α1-adrenergic antagonist and a central α2-adrenergic agonist reduced the rise in temperature. The β2-adrenergic agonist clenbuterol-induced hyperthermia is mediated by β-adrenergic receptors. However, the hyperthermic effect of clenbuterol may result from secondary α2-adrenergic agonist stimulation centrally, perhaps from the norepinephrine release that results from β-adrenergic stimulation. In support of this is that the α2-adrenergic antagonists block the increase in temperature caused by clenbuterol.184
Studies on muscarinic receptors demonstrate involvement of muscarinic M2 and M3 receptors in the production of hypothermia when agonists to these receptors are administered centrally.221 Blockers of ATP-sensitive K+ channels can reverse the effect of cholinomimetic drugs in producing hypothermia.206
Many xenobiotics have pharmacologic effects that interfere with thermoregulatory responses (Tables 29–1 and 29–2).168,170,252 α-Adrenergic agonists prevent vasodilation in response to heat stress. Increased endogenous heat production in the setting of increased motor activity occurs during exercise and also occurs in patients manifesting cocaine or amphetamine toxicity. Life-threatening hyperthermia is associated with the use of these xenobiotics. Whereas adrenergic antagonists and calcium channel blockers diminish the cardiac reserve available to compensate for heat-induced vasodilation, diuretics decrease cardiac reserve through their effects on intravascular volume.63 β-Adrenergic antagonists also interfere with the capacity to maintain normothermia under conditions of cold stress, possibly related to their interference with the mobilization of substrates required for thermogenesis.170 Opioids and diverse sedative–hypnotics depress hypothalamic function and predispose to hypothermia.85 Carbon monoxide poisoning must also be considered in hypothermic patients. Organic phosphorus compounds and other xenobiotics that cause cholinergic stimulation cause hypothermia by stimulation of inappropriate sweating and possibly through depression of the endogenous use of calorigenic substrates.170 Xenobiotics with anticholinergic effects decrease sweating and predispose to hyperthermia, which is of particular concern during environmental heat exposure or exercise. Phenothiazines appear to interfere with normal response to both heat and cold. Severe hyperthermia associated with the absence of sweating is frequently described in patients using phenothiazines and is a consequence of their anticholinergic effects.222,264 In addition, phenothiazines have central effects interfering with the hypothalamus, commonly although incompletely attributed to the antidopamine effect. Effects on cold tolerance are attributed to their α-adrenergic antagonist effects, which prevent vasoconstriction in response to cold stress.169 In addition, hyperthermia associated with severe extrapyramidal rigidity occurs in patients taking antipsychotics.161 This rigidity is attributed to the dopamine-blocking effects of this class of drugs.
I. Impaired cutaneous heat loss |
A. Vasoconstriction through α-adrenergic stimulation |
Amphetamines |
Cocaine |
Ephedrine |
Phenylpropanolamine |
Pseudoephedrine |
B. Sweat gland dysfunction |
Antihistamines |
Antimuscarinics |
Cyclic antidepressants |
Topiramate |
Zonisamide |
II. Myocardial depression |
A. Decreased cardiac output |
Antidysrhythmics |
β-Adrenergic antagonists |
Calcium channel blockers |
B. Reduced cardiac filling by salt and water depletion |
Diuretics |
Ethanol |
III. Hypothalamic depression |
Antipsychotics |
IV. Impaired behavioral response |
Cocaine |
Ethanol |
Opioids |
Phencyclidine |
Sedative–hypnotics |
V. Uncoupling of oxidative phosphorylation |
Dinitrophenol |
Pentachlorophenol |
Salicylates |
VI. Increased muscle activity through agitation, seizures, or rigidity |
Amphetamines |
Caffeine |
Cocaine |
Isoniazid |
Lithium |
Monoamine oxidase inhibitors |
Phencyclidine |
Serotonin toxicity |
Strychnine |
Thyroid hormone excess |
VII. Dystonia |
Antipsychotics |
Neuroleptic Malignant Syndrome (NMS) |
VIII. Withdrawal |
Dopamine agonists |
Ethanol |
Sedative–hypnotics |
Impaired nonshivering thermogenesis |
β-Adrenergic antagonists |
Cholinergics |
Hypoglycemics |
Impaired perception of cold |
Carbon monoxide |
Ethanol |
Hypoglycemics |
Opioids |
Sedative–hypnotics |
Impaired shivering by hypothalamic depression |
Antipsychotics |
Carbon monoxide |
Ethanol |
General anesthetics |
Opioids |
Sedative–hypnotics |
Impaired vasoconstriction |
α-Adrenergic antagonists |
Antipsychotics |
Ethanol |
Ethanol is the xenobiotic most commonly related to the occurrence of hypothermia by virtue of its effects on CNS depression, vasodilation, and blunting of behavioral responses to cold. However, thermoregulatory dysfunction associated with ethanol intoxication is undoubtedly more complex.
In animal models, ethanol leads to hypothermia, the extent of which is partly dependent on ambient temperature.194,212,213 In mice, as the dose of ethanol increased, body temperature decreased, and the rate of this decline in body temperature was faster at higher ethanol doses.190 The decline in body temperature could be reversed by increasing ambient temperature; increasing ambient temperature to 96.8°F (36°C) caused an immediate rise in the body temperature.190 The poikilothermic effect of ethanol was not a result of hypoglycemia. Poikilothermia is the variation in body temperature greater than ±3.6°F (±2°C) on exposure to environmental temperature changes. Rats treated with equipotent amounts of pentobarbital showed the same effects on body temperature as rats treated with ethanol, suggesting a similar central mechanism of CNS depression resulting in altered thermoregulation.190
Numerous mechanisms are involved in the ethanol-induced depression of CNS function.215 Genetic factors influence the role of ethanol in the production of hypothermia. Mice are selectively bred for genetic sensitivity or insensitivity to acute ethanol-induced hypothermia, and the differences appear to be mediated by the serotonergic systems.86,100,185,190 Cyclo His-Pro DKP, another neurotransmitter that is found in many animal species, acts at the preoptic-anterior hypothalamus to modulate body temperature. Exogenous administration of this neuropeptide produced a dose-dependent decrease in ethanol-induced hypothermia. Attenuation of hypothermia resulted from passive immunization with Cyclo His-Pro DKP antibody.37,130 Ethanol effects appears to be mediated through modulation of endogenous opioid peptides because high-dose (10 mg/kg) naloxone reverses ethanol-induced hypothermia in animals.202
Pharmacokinetic characteristics of ethanol metabolism change in the presence of hypothermia. Hypothermic piglets infused with ethanol showed slower ethanol metabolism and a smaller volume of distribution (Vd) and, as a result, higher ethanol concentrations than normothermic control piglets. Ethanol elimination and metabolism decreased as temperature fell.148
Tolerance develops to the effect of ethanol-induced hypothermia in all species.88,194 The degree of tolerance is proportional to the dose and duration of treatment with ethanol and is not explained by the increased rate of metabolism with chronic exposure.134 Age is a factor in the development of tolerance; older animals do not display the same degree of tolerance to the hypothermic effects of chronic ethanol administration as do younger animals.187,202,262 The development of tolerance to ethanol-induced hypothermia is affected by genetic factors. Experimentally, tolerance to ethanol-induced hypothermia increases the incorporation of certain amino acids into proteins in the rat brain. The formation of new proteins in ethanol-tolerant rats suggests stimulation of gene expression associated with ethanol tolerance.134,255 Upregulation of the in N-methyl-D-aspartate (NMDA) receptors are also implicated in the development of ethanol tolerance. In addition, altered nicotinamide adenine dinucleotide (NADH) oxidation to NAD+, diminishes blood flow to the liver, or slows metabolism through the microsomal enzyme system.215
Hypothermia alters the breath–ethanol partition in the alveolus, and the temperature of expired breath alters breath–ethanol analysis results. In patients with mild hypothermia, breath–ethanol analysis results in lower values by 7.3% per degree centigrade (or 1.8°F) decrease in body temperature.91 Whether breath–ethanol analysis is also affected by hyperthermia remains to be studied.91
Many disease processes interfere with normal thermoregulation, limiting an individual’s capacity to prevent hypothermia or hyperthermia. Extensive dermatologic disease or cutaneous burns impair sweating and vasomotor responses to heat stress.34 Patients with autonomic disturbances secondary to diabetes mellitus or peripheral vascular disease also have altered vasomotor responses that impair vasodilation and sweating.236 Extensive surgical dressings preclude the evaporation of sweat in an otherwise normal patient. Heat-stressed persons with poor cardiac reserve and lower cardiac output are not able to sustain skin blood flow rates high enough to maintain normothermia.77,240 Intense motor activity leads to excessive heat production in all patients, particularly those with Parkinson disease or hyperthyroidism. Patients with agitated delirium or seizures also have significantly elevated rates of endogenous heat production. Hypothalamic injury caused by cerebrovascular accidents, trauma, or infection disturb thermoregulation.76,165 Hypothalamic dysfunction leads to high, unremitting fevers and insufficient stimulation of heat loss mechanisms such as sweating. Hypothalamic damage predisposes to hypothermia by interference with centrally mediated heat conservation.76,165,223,224 Fever, the normal response to stimulation of the hypothalamus by pyrogens, results in an elevated physiologic temperature set point and is a disadvantage in the heat-stressed individual120 (Table 29–3).
Advanced age | Immobilization |
Decreased ability to shiver | Central nervous system dysfunction illness |
Decreased metabolic rate | Spinal cord injury |
Decreased temperature discrimination | Trauma |
Reduced peripheral blood flow | Medical Diseases |
Central nervous system depression | Hepatic failure |
Cerebrovascular accident | Sepsis |
Ethanol | Uremia |
Hypothalamic dysfunction | Nutritional |
Infection | Glycogen depletion |
Xenobiotics, diverse | Hypoglycemia |
Endocrine | Starvation |
Adrenal insufficiency | Thiamine deficiency |
Diabetic ketoacidosis | Social |
Hyperosmolar coma | Failure to use indoor heating |
Hypopituitarism | Homelessness |
Hypothyroidism | Inadequate indoor heating |
Environmental | Poverty |
Homelessness | Social isolation |
Unintentional exposure to …. |
Hypothermia is defined as a lowering of the core body temperature to below 95°F (<35°C). Between 2003 and 2013, there were 13,419 hypothermia-related deaths for the United States overall. The Centers for Disease Control and Prevention defines these deaths as those with an underlying or contributing cause of death from exposure to excessive natural cold45 (Table 29–3).
Alaska, Montana, Wyoming, and New Mexico had the greatest overall death rates from hypothermia. States with milder climates and rapid fluctuations in temperature, such as North and South Carolina, and western states, such as Arizona, with high elevations and cold nighttime temperatures also report hypothermia-related deaths.44
The normal physiologic response to cold is initiated by stimulation of cold-sensitive neurons in the skin, so that the onset of the body response to cold occurs before cooling of central blood. Cold-sensitive neurons in the skin send afferent impulses to the hypothalamus, resulting in shivering and piloerection. Shivering is the main thermoregulatory response to cold in humans, except in neonates, in whom nonshivering thermogenesis prevails. Shivering is initiated in the posterior hypothalamus when impulses from cold-sensitive thermoreceptors are integrated in the anterior hypothalamus and communicated to the posterior hypothalamus or when cold-sensitive neurons in the posterior hypothalamus are activated directly. Efferent stimuli from the posterior hypothalamus travel through the midbrain tegmentum, pons, and lateral medullary reticular formation to the motor pathways of the tectospinal and rubrospinal tracts, resulting in shivering.19 A mechanism of stimulation of shivering that usually occurs later when core temperature drops is the local cooling of the spinal cord, which leads to shivering by increasing excitability of motor neurons.
Heat produced without muscle contraction is known as nonshivering thermogenesis.32,120 Nonshivering thermogenesis is mediated by the sympathetic nervous system.54 Catecholamines activate adenyl cyclase, increasing cAMP, resulting in mobilization of fat and glucose stores (β-adrenergic receptors).171,217 Nonshivering thermogenesis is blocked by α-adrenergic receptor antagonism and increased by administration of norepinephrine. Brown adipose tissue is the most important site of nonshivering thermogenesis. In humans, brown fat is found primarily in neonates, although in cold-acclimatized people, small amounts are found on autopsy.32 Brown adipose tissue functions as a thermoregulatory effector organ, producing heat by the oxidation of fatty acids when the tissue is stimulated by norepinephrine.36
In addition to shivering and nonshivering thermogenesis, efferent sympathetic fibers from the hypothalamus stimulate peripheral vasoconstriction (α-adrenergic receptors). Piloerection and vasoconstriction result in decreased heat loss from the body. Intense vasoconstriction shunts blood away from the periphery to the core and antidiuretic hormone antagonism results in increased urine output and hemoconcentration.
Several disease processes commonly result in an inability to maintain a normal body temperature in a cool environment (Table 29–3).65,163
Evaluations to determine the presence of underlying diseases are often difficult in a patient with hypothermia.67,89,163 The mental status is not usually significantly abnormal until the temperature falls below 90°F (32.2°C). If normal mental status is not regained when the temperature reaches 90°F (32.2°C) during rewarming, underlying CNS structural, toxic, or metabolic problems are probable.67,89,163,207 Failure of the patient to rewarm quickly suggests the presence of underlying disease67 (Fig. 29–2). In one study, whereas hypothermic patients without underlying disease are reported to rewarm at a rate of 1.0°F/h to 3.7°F/h (0.6°C/h–2.1°C/h) (average, 2.1°F/h; 1.2°C/h), patients with significant underlying disease (sepsis, gastrointestinal hemorrhage, diabetic ketoacidosis, pulmonary embolus, myocardial infarction) warmed at a rate of 0.25°F/h to 1.8°F/h (0.1°C/h–1.0°C/h) (average, 1°F/h; 0.6°C/h).67,258
The pharmacology of certain xenobiotics is altered in the setting of hypothermia. In hypothermic piglets, the Vd and the clearance of fentanyl are decreased.149 Similarly, in piglets given gentamicin, the Vd and clearance rate decreased in direct proportion to the decrease in cardiac output and glomerular filtration rate (GFR).147 Hypothermic puppies given intravenous (IV) lidocaine showed slower rates of disappearance of the drug than when normothermic.186 Humans and animals given propranolol showed a reduced Vd and decreased total body clearance, resulting in higher than expected propranolol concentrations.175,176,192 Decreased hepatic metabolism of propranolol during hypothermia is demonstrated in vitro.176 Hypothermia prolongs neuromuscular blockade with d-tubocurarine111 and increases neuromuscular blockade with suxamethonium.262 Phenobarbital metabolism and Vd decreased with hypothermia in children.133 The lethal dose of digoxin was doubled in hypothermic dogs.17 Digoxinlike substances are present during hypothermia.124
Reasons for altered metabolism in hypothermia include delayed distribution of the drug and altered enzyme function with temperature and pH changes. Cardiac output decreases, leading to decreased liver perfusion and decreased delivery of drug to hepatic microsomal enzymes.111,136-138,200 Plasma volume decreases as free water moves intracellularly, causing hemoconcentration and further decreasing organ perfusion.112 Biliary excretion of atropine, procaine, and sulfanilamide decreases in vitro.136-138 In vitro, the activity of metabolic pathways, including acetylation and hydrolysis, decrease with cooling.136,137
These same metabolic changes occur in therapeutic hypothermia. Hypothermia is induced for its neuroprotective effects but requires attention to alterations in pharmacokinetics and pharmacodynamics of xenobiotics administered during hypothermia. Physiological changes during induced hypothermia are similar to those of unintentional hypothermia and include slowed enzymatic reactions, vasoconstriction, decreased-cardiac output, and hemoconcentration. Studies support a decrease in the Vd of pancuronium, midazolam, morphine, and gentamicin.64 Clearance of xenobiotics is affected by organ perfusion, enzyme activity, and xenobiotic characteristics such as the degree of protein binding and the pKa. Hepatic and renal clearance is affected by regional blood flow, which is decreased during hypothermia. In general, the decrease in the Vd and the decrease in clearance result in increased serum xenobiotic concentrations.64 Experimental studies in animals demonstrate a 25% increase in fentanyl concentrations at a core temperature of 32°C.92 Concurrent-supportive modalities during hypothermia such as cardiopulmonary bypass or extracorporeal membrane oxygenation (ECMO) further alter the kinetics of xenobiotics during hypothermia.260 In neonates, asphyxia treated with induced hypothermia presents the additional complication of rapidly changing neonatal physiology and the effects of supportive measures such as ECMO.64
The clinical effects of hypothermia are related to the membrane depressant effects of cold, which result in ionic and electrical conduction disturbances in the brain, heart, peripheral nerves, and other major organs123 (Table 29–4). Cold tissues are protected by decreases in tissue oxygen requirements. As body temperature decreases, metabolic activity declines at a rate of approximately 7% per 1.8°F (1°C).263 This effect provides significant protection to vital organs despite the potentially deleterious effects of membrane suppression.
Cardiovascular |
Normal, decreased, or increased cardiac output |
Normal heart rate or tachycardia 20 to shivering, then bradycardia as severity of hypothermia increases |
Vasoconstriction and central shunting of blood |
Electrocardiogram |
Prolongation of intervals (PR, QRS and QT) |
Atrial fibrillation |
Increased ventricular irritability |
J-point elevation (“Osborn waves”) |
Central nervous system |
Mild: 90°F–95°F (32°C–35°C) |
Normal mental status or slightly slowed |
Moderate: 80°F–90°F (27°C–32°C) |
Lethargic but verbally responsive |
Severe: 68°F–80°F (20°C–27°C) |
Unlikely to respond verbally or purposefully to noxious stimuli |
Profound: <68°F (<20°C) |
Unresponsive; appears dead |
Gastrointestinal tract |
Decreased motility |
Depressed hepatic metabolism |
Hematologic |
Hemoconcentration |
Left shift of oxyhemoglobin dissociation curve |
Kidneys |
Cold-induced diuresis (antidiuretic hormone antagonism) |
Lungs |
Hyperventilation to hypoventilation with increasing hypothermia |
Bronchorrhea |
Metabolic |
Metabolic acidosis |
Increased glycogenolysis |
Increased serum free fatty acids |
Normal thyroid and adrenal function |
Effects on the CNS are temperature dependent. However, predicting the temperature of the patient based on the clinical presentation is unreliable.31,33,71 The patient with mild hypothermia (90°F–95°F; 32.2°C–35°C) may have a relatively normal clinical appearance. Ataxia, clumsiness, slowed response to stimuli, and dysarthria are common.89 As cooling continues, the mental status slowly deteriorates. In moderate hypothermia (80°F–90°F; 27°C–32.2°C), the patient is usually lethargic but still likely to respond verbally. In severe hypothermia (68°F–80°F; 20°C–26.6°C), the patient is unlikely to respond verbally but will react purposefully to noxious stimuli.89,116 With profound hypothermia (<68°F; <20°C), the patient is unresponsive to stimuli. The patient “appears dead”.116 However, standard criteria for brain death do not apply to hypothermic patients. The hypothermia itself protects against cerebral hypoxic damage.123 Temperature drop inhibits the release of the excitatory neurotransmitter glutamate and attenuates the release of dopamine in animal models of brain ischemia, suggesting a protective effect of hypothermia in brain injury.39 Cerebrospinal fluid (CSF) glutamate concentrations were lower in patients showing benefit from mild induced hypothermia after brain injury compared with brain-injured patients kept normothermic.173
Patients have survived with body temperatures as low as 48.2°F (9°C).93 Vigorous resuscitation is required for these patients. This approach leads to hours of cardiopulmonary resuscitation (CPR) of hypothermic patients with ventricular fibrillation, ventricular tachycardia, or asystole but is sometimes successful in resuscitating patients initially presumed to be dead.238
For field rescue work, the Swiss distinguish between 5 stages of hypothermia and provide guidelines for rescue in dangerous environmental conditions. These stages are based on the degree of consciousness, the presence or absence of shivering, cardiac activity, and core temperature.33,75 The adage that a patient cannot be considered dead until the patient is warm and dead is further refined by the Swiss guidelines for field rescue attempts under avalanche conditions.
The cardiac and hemodynamic effects of cold correlate closely with body temperature. As cooling begins, there is a transient increase in cardiac output. Tachycardia develops secondary to shivering and sympathetic stimulation. At about 81°F (27.2°C), shivering ceases. Bradycardia develops with maintenance of a normal cardiac stroke volume.35,67 This bradycardia is responsible for the decreased myocardial oxygen demand, which is protective in the setting of hypothermia.35 In profound hypothermia, bradycardia may progress to asystole and death.
Unlike cerebral circulation, where autoregulation is preserved during cooling, coronary autoregulation is disturbed during hypothermia, and the myocardium is at risk of compromise.142 Attempts to maximize myocardial oxygenation through administration of oxygen and volume replacement to increase diastolic filling pressures are recommended.
The initial response to cold is hyperventilation; however, as temperature continues to decrease, hypoventilation develops, which infrequently progresses to apnea and death. In animal models, this is attributed to cold-induced failure of phrenic nerve conduction.142
The most common electrocardiographic (ECG) abnormality in hypothermia is generalized, progressive depression of myocardial conduction. The PR, QRS, and QT intervals all prolong, and increasingly profound hypothermia leads to gradual progression to asystole.74,251 Ventricular fibrillation occurs in an irritable myocardium most commonly at temperatures less than 86°F (30°C), resulting in a high O2 consumption dysrhythmia. Atrial fibrillation is the most common dysrhythmia occurring in the presence of hypothermia.90,201,253 Shivering is not always clinically evident, but the characteristic fine muscular tremor frequently produces a mechanical artifact in the baseline of the ECG.78 A deflection occurring at the junction of the QRS and ST segment is invariably present in patients with temperatures <86°F (<30°C) (Fig. 29–3). First described in a single patient in 1938, the J-point deflection is commonly known as the Osborn wave.79,203,247 The J-point deflection, thought to be a “current of injury” associated with CO2 retention under hypothermic conditions, was believed to be a poor prognostic sign.203 Subsequent study does not support any prognostic significance because the J-point deflection is invariably found in hypothermic patients when multiple ECG leads are obtained.78,79,201,249,253 The size of the J-point deflection increases as body temperature decreases.219,282 Atrial dysrhythmias that occur in the absence of underlying heart disease invariably disappear solely with rewarming.
After blood specimens are drawn, the hypothermic patient in whom hypoglycemia is present should be given 0.5 to 1.0 g of dextrose/kg of body weight as D50W (50% dextrose in water in adults or D20W or D10W as appropriate for children) and at least 100 mg of IV thiamine. If hypoglycemia is the cause of the hypothermia, the response to dextrose is usually dramatic, heralded by the onset of shivering and rapid return to normal body temperature. Wernicke encephalopathy is uncommon but is associated with mild hypothermia; thermoregulation and normal ocular motion typically returns after the initiation of thiamine therapy.57
Hypothermia shifts the oxygen dissociation curve to the left (Chap. 28), resulting in decreased oxygen unloading to tissues; therefore, oxygen administration is reasonable.66 If clinically indicated, endotracheal intubation should be performed for airway protection or inadequate ventilation or oxygenation.60 However, there are rare case reports of ventricular fibrillation occurring during endotracheal intubation.14,99,116,204 Every effort should be made to limit patient activity and stimulation during the acute rewarming period because activity increases myocardial oxygen demand or alters myocardial temperature gradients, increasing the risk of iatrogenic ventricular fibrillation. Although pulmonary artery catheters and central venous lines are typically inserted in clinically unstable patients they should be avoided unless absolutely essential so as not to precipitate ventricular dysrhythmias.114,157,248 If a central venous catheter is considered necessary, it should not be allowed to touch the endocardium.250 Patients who develop ventricular fibrillation or asystole are difficult to manage because of the many supportive therapies required at once and the often lengthy resuscitation efforts. In these instances, CPR should be initiated and the patient intubated and ventilated to maintain a pH of 7.40, uncorrected for temperature.66 Active internal rewarming (see later discussion) should be instituted in the patient in cardiac arrest because standard therapy for ventricular fibrillation or asystole are often unsuccessful until rewarming is achieved. There is a risk of toxicity if multiple doses of drugs are administered in the hypothermic patient in whom drug metabolism is altered and circulation is arrested. However, based on current research discussed later, it is reasonable to incorporate pharmacotherapy into the management of hypothermic cardiac arrest. A vasopressor, either epinephrine or vasopressin, is reasonable to increase the coronary perfusion pressure. Amiodarone or lidocaine should be administered if ventricular fibrillation is present. Defibrillation in humans is often not successful until the temperature exceeds 86°F (30°C); however, defibrillation can be successfully accomplished in animals and patients with temperatures of less than 86°F (30°C).6,14,61,261 A reasonable approach is that if defibrillation is unsuccessful below 86°F (30°C), defibrillation should not be attempted again until the patient is warmed several degrees centigrade. Pneumatically powered devices capable of mechanical chest compression and cardiopulmonary bypass are appropriate and safe during prolonged hypothermic cardiopulmonary arrests.14,53,239,248
Assessment of the adequacy of ventilation and oxygenation in the hypothermic patient often poses a dilemma to clinicians because the chemical effects of cold on arterial pH and blood gases lead to confusion in the interpretation of arterial blood gas values. Cold inhibits the dissociation of water molecules, causing pH to increase as cooling occurs. In vitro, the pH change of blood as it is cooled increases parallel to the pH change of neutral water. The partial pressures of CO2 and O2 decrease as cooling occurs even as the blood content of those gases remains unchanged. Blood in a syringe taken from a patient whose body temperature is 98.6°F (37°C) yields a pH of 7.40 and a PCO2 of 40 mm Hg in the blood gas machine at 98.6°F (37°C) but yields a pH of 7.72 and a PCO2 of 14 mm Hg if the blood is cooled to 61°F (16°C) and the values are measured at that temperature. Specially calibrated laboratory equipment, not routinely available, is required to measure blood gas values directly at other than normal body temperature. A patient whose body temperature is 61°F (16°C) and whose actual in vivo blood gas values are a pH of 7.72 and PCO2 of 14 mm Hg will have a pH of 7.40 and PCO2 of 40 mm Hg when the blood is warmed to 98.6°F (37°C) and measured in the standard laboratory blood gas machine. Because the machine measures pH and blood gas pressures only in blood warmed to 98.6°F (37°C) (the uncorrected values), the actual in vivo values in hypothermic patients can be approximated using mathematically derived corrected values. Because the pH of neutrality has also increased, it is unclear what clinical meanings these corrected values have. The uncorrected values indicate what the pH and PCO2 would be if the patient were normothermic. At first glance, the clinician might be content to learn that a hypothermic patient at 61°F (16°C) has a corrected pH of 7.47 and PCO2 of 40 mm Hg. However, the uncorrected values in the blood gas machine at 98.6°F (37°C) of pH of 7.18 and PCO2 of 111 mm Hg indicate that the patient has a significant respiratory acidosis. Attempts to maintain a corrected pH of 7.40 will lead to hypoventilation and risk alveolar collapse and impairment of oxygenation. The preponderance of evidence in the anesthesia and cardiovascular surgery literature suggests that maintenance of ventilation is associated with a decreased incidence of myocardial injury and a decreased incidence of ventricular fibrillation.66 Thus, the pH and PCO2 blood gas values should be left uncorrected after the blood sample is warmed in the blood gas machine and interpreted in the same way as in a normothermic patient.66
When hypotension occurs in a patient with hypothermia, it is possibly a result of the presence of bradycardia and volume depletion. However, hypotension is a predictor of infection, particularly when associated with a slow rewarming rate.67 In hypothermia, salt and water depletion occurs as a result of a variety of mechanisms, including central shunting of blood by vasoconstriction and cold-induced diuresis. Cold diuresis occurs when increases in central blood volume result in inhibition of the release of antidiuretic hormone. Impairment of renal enzyme activity and decreased renal tubular reabsorption contribute to the large quantities of dilute urine known as cold diuresis.112,116,157,263 An infusion of 0.9% NaCl should be given to expand intravascular volume. Urine output is an important indicator of organ perfusion and the adequacy of intravascular volume in hypothermic patients, although the initial cold diuresis leads to underestimation of fluid needs.263
The best means to effect resuscitation of a hypothermic victim in ventricular fibrillation is controversial. The most recent recommendations of the American Heart Association (AHA) for the treatment of cardiac arrest when the core body temperature is below 86°F (<30°C) states that the administration of a vasopressor or antidysrhythmic according to standard Advanced Cardiac Life Support algorithm concurrent with rewarming is reasonable.7,156 A meta-analysis of animal studies of antidysrhythmic and vasopressor therapy for ventricular fibrillation in the setting of hypothermia found that there was significant improvement in the return of spontaneous circulation (ROSC) in animals that received epinephrine or vasopressin.261 In this report, the authors could not identify a benefit to the use of amiodarone administered without a vasopressor. They postulated that this is most likely due to the improvement in coronary perfusion pressure caused by vasopressors during cardiac arrest.261 The benefit of amiodarone in profound hypothermia is probably limited but is reasonable and as there are no other options indicated.
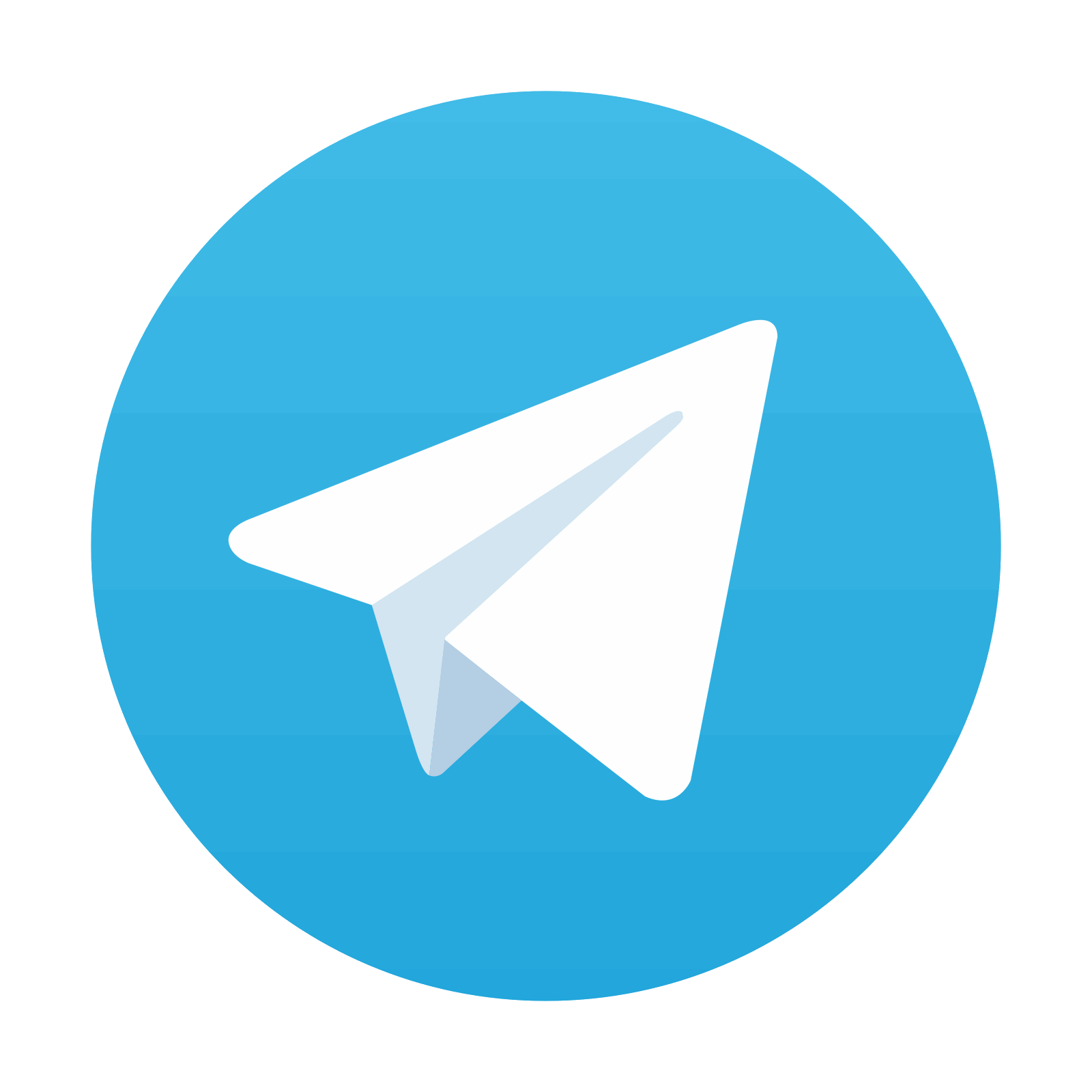
Stay updated, free articles. Join our Telegram channel

Full access? Get Clinical Tree
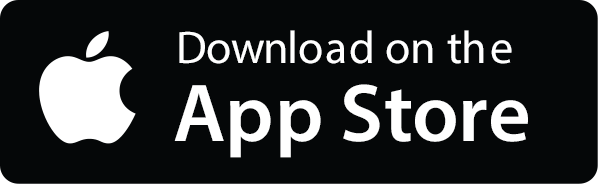
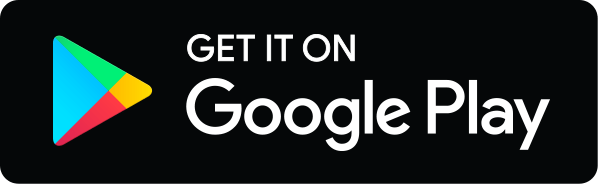