I
II
III
IV
Blood loss (ml)
Up to 750
750–1,500
1,500–2,000
>2,000
Blood loss (% blood volume)
Up to 15
15–30
30–40
>40
Pulse rate (per minute)
<100
100–120
120–140
>140
Blood pressure
Normal
Normal
Decreased
Decreased
Pulse pressure (mmHg)
Normal or increased
Decreased
Decreased
Decreased
Respiratory rate (per minute)
14–20
20–30
30–40
>35
Urine output (ml/h)
>30
20–30
5–15
Negligible
Central nervous system/mental status
Slightly anxious
Mildly anxious
Anxious, confused
Confused, lethargic
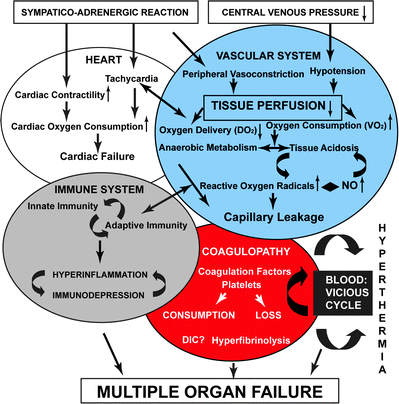
Fig. 1.1
Pathophysiology of hemorrhagic shock. Reprinted from Angele MK, Schneider CP, Chaudry IH. Bench to bedside review: latest results in hemorrhagic shock. Crit Care. 2008;12(4):218. Epub 2008 Jul 10. with permission of BioMed Central
Hemorrhage is commonly categorized by volume and percent blood loss with specific findings at defined losses [2]. Interestingly these categories are largely based on opinion rather than objective clinical data. Clinical parameters are not markedly different from baseline in phases one and two of shock, contributing to the difficulty in recognizing shock in its early stages. In providing care to the critically injured patient, it is of utmost importance to have the ability to diagnose impending or early hemorrhagic shock. It is rather easy to diagnose severe hemorrhagic shock; however, the affected patients have already undergone cardiovascular collapse and are near death. The astute clinician will prefer to intervene earlier, when the diagnosis is more obscure. Once recognized, directed treatment of imminent shock or ongoing hemorrhage begins.
During field resuscitation, patients receive treatments necessary to control bleeding. Several centers tout an integrated database or registry to incorporate pre-hospital data to analyze outcomes (Table 1.2) [3–5]. Such pre-hospital interventions to consider in the management of hemorrhage are infusions of crystalloid and placement of tourniquets. Ultimately all patients in hemorrhagic shock need definitive control of the bleeding before there will be any chance of salvage.
Table 1.2
Current indications and contraindications for EDT
Indications Salvageable post-injury cardiac arrest: Patients sustaining witnessed penetrating trauma with <15 min of pre-hospital CPR Patients sustaining witnessed blunt trauma with <5 min of pre-hospital CPR Persistent severe post-injury hypotension (SBP ≤60 mmHg) due to: Cardiac tamponade Hemorrhage—intrathoracic, intra-abdominal, extremity, cervical Air embolism |
Contraindications Penetrating trauma: CPR >15 min and no signs of life (pupillary response, respiratory effort, or motor activity) Blunt trauma: CPR >5 min and no signs of life or asystole |
Crystalloid
Choice of crystalloid as a resuscitation fluid in the face of known hemorrhagic shock remains one of the most highly debated topics in the trauma literature at this time. It seems intuitive that if a person is hemorrhaging, correction of that shock will be contingent on the repletion of blood, and that his or her coagulopathy will respond to transfusion of plasma and platelets. However, replacement of volume by crystalloid represents classical teaching and guidelines for correction of the initial phase of hemorrhagic shock [6]. Advanced Trauma Life Support (ATLS) [2] discusses placing two large-bore IVs and bolusing 2 liter of crystalloid for any patient assumed to be in hemorrhagic shock or any patient with significant blood loss. However, recent data suggest that as little as 1.5 l of fluid has negative clinical implications and numerous sources are refuting the benefit of large-volume crystalloid resuscitation in hemorrhagic shock [7].
While many clinicians consider lactated Ringer’s and normal saline interchangeable, they are not. Multiple studies in the swine model compare the use of various crystalloid solutions, focusing on lactated Ringer’s solution and normal saline. The swine model demonstrates that if shock is induced and maintained for 30 min, followed by resuscitation with either normal saline or lactated Ringer’s solution, the animals resuscitated with Ringer’s lactate have better improvement in markers of shock, pH, and extracellular lung water [8]. In this study neutrophil activation contributes to cellular damage. Other studies support the neutrophil activation phenomenon; dextran is the biggest activator, followed by normal saline and then lactated Ringer’s [9]. Colloid, plasma, and blood have also been implicated as morbid contributors to effects on neutrophil activation, mainly in the pulmonary system [10–12].
Lactated Ringer’s, as a resuscitation fluid, yields less acidosis and less coagulopathy than seen with similar volumes of normal saline [13]. Normal saline causes a well-recognized metabolic hyperchloremic acidosis; patients resuscitated with lactated Ringer’s do not achieve such levels of acidosis. Furthermore, normal saline-resuscitated patients demonstrate more blood loss than those resuscitated with lactated Ringer’s [8, 14]. This has been demonstrated also in the vascular literature. In a study of aortic repairs it was shown that there was more perioperative bleeding and acidosis when normal saline was used as opposed to lactated Ringer’s [15]. There was no statistically significant difference in outcome however. Even despite the better physiologic results with lactated Ringer’s resuscitation as compared to normal saline, lactated Ringer’s still would not be the first choice for resuscitation in a patient with hemorrhagic shock as excessive bleeding is not well controlled with replacement of volume by crystalloid [16].
While several centers still practice crystalloid-based resuscitation, many trauma centers are moving toward the practice implemented during the ongoing war in Iraq and Afghanistan where the standard of care is to transfuse blood and blood products immediately and limit the crystalloid volume during the initial resuscitation [17, 18]. Excessive crystalloid has been implicated in increased mortality and morbidity rates when used in large volume in the trauma patient [19–21].
Permissive hypotension purposefully maintains mean arterial pressure as low as possible to ensure adequate organ perfusion. If the minimum mean arterial pressure is not exceeded with over-resuscitation, the delicate new clot formation should not be disrupted prior to operative intervention [22, 23]. These authors show that by purposefully maintaining mean arterial pressure no greater than 50 mmHg, the patients in these groups are not afflicted with coagulopathy to the same degree as controls that are resuscitated to a mean arterial pressure of greater than 65 mmHg. Earlier data from animal models show no difference in ultimate outcome when hypotension is maintained; end organ perfusion and prevention of metabolic perturbations that can occur when tissue oxygenation is inadequate are the goals of permissive hypotension [24]. That is to say that when metabolic acidosis is controlled and the mean arterial pressure is minimized on purpose, patients do not show any long-term adverse effects compared to patients whose resuscitation targets a higher mean arterial pressure. It is unclear how long patients can remain hypotensive without deleterious effects. The original descriptions of this concept date to World Wars 1 and 2. The original civilian studies on this topic show less intraoperative bleeding and overall fluid requirement and hence less postoperative morbidity when this strategy is applied [25]. Survival is improved by limiting crystalloid infusion. Furthermore overaggressive resuscitation to a physiologically normal blood pressure may contribute to ineffective hemostasis, termed “popping the clot,” shown in an animal study where raising the blood pressure caused re-bleeding and increased mortality [26]. This cycle of repeated resuscitation and bleeding is ultimately detrimental to clot stability and to overall survival [27].
The use of tourniquets in the field has turned some of the most life-threatening injuries into ones where life and limb can be salvaged. The resurgent use of tourniquets has been overwhelmingly supported in the recent military data from the Iraq and Afghanistan experience, where it is shown that there are virtually no adverse effects of the tourniquet itself [28]. Even in inexperienced hands, tourniquets have been shown to prevent life-threatening exsanguination and should be applied in any situation in which extremity hemorrhage exists and prior to the onset of exsanguination [28, 29]. Mangled extremities are not more likely to require amputation when a tourniquet is applied. There are several commercial devices available and their purpose is to exert enough circumferential pressure to prevent blood from flowing into the extremity in question [30]. Contrary to older teaching, use of a tourniquet does not cause increased amputation rates [31].
Hospital Arrival
Once in the emergency department, indices of vital signs and laboratory values may assist the surgeon in separating the critically ill trauma patient from one who appears “stable.” Lactate, serum bicarbonate, base deficit, hemoglobin, or tissue oxygenation are some of the most crucial lab values in determining metabolic acidosis, which occurs with poor tissue oxygen extraction and indicates shock at the cellular level [32–38]. Lactate, in the pre-hospital setting, may be more predictive of prognosis than are vital signs, which can be fairly stable until hemodynamic collapse ensues [39]. Lactate increases in under-perfused tissues and can be an early predictor of impending shock, and helps differentiate the stable patient from the one in a compensated shock state.
Base deficit is a reflection of metabolic acidosis secondary to unmeasured anions, which is typically assumed to be lactate in the trauma patient [40]. Base deficit, lactate, anion gap, and bicarbonate levels all correspond to metabolic acidosis and have all been shown to predict morbidity and mortality [41–44]. However, bicarbonate is only a single marker of acid–base status, whereas anion gap, base deficit, and lactate all have some dependence on electrolytes, pH, and buffer capacity of blood [45]. There does not seem to exist great consensus in the literature regarding which is the best predictor of mortality [46].
Up to a third of patients in the ICU show discordance between their base deficit and lactate, and in these situations it has been shown that lactate is more predictive of overall outcome, when it differs significantly from base deficit [47]. Authors from this source imply that base deficit on its own does not have the predictive capacity for mortality that lactate has. On the other hand, while lactate is the most helpful in the initial phase of resuscitation, it is not as accurate in determining the ongoing causes of metabolic acidosis in critical situations outside of trauma where lactate may not elevate, such as respiratory alkalosis and diabetic ketoacidosis.
Serum bicarbonate will correlate with base deficit only when the pH is constant, which has clinical implications in the patient whose standard chemistry is drawn from a venous line at a different time than the arterial blood gas is collected [48]. The fluctuating pH may affect the accuracy of either measurement when compared to the other. There may be a significant difference in base deficit when comparing arterial to venous samples. Venous samples may be more sensitive to changes in pH, pCO2, and pO2 resulting in earlier changes in base deficit [49].
Acidosis, coagulopathy, and hypothermia portend the downward spiral into fulminant hemorrhagic shock. The key to understanding hemorrhagic shock is to understand the interactions of the lethal triad and the human body’s capacity to self-correct versus what must be medically and surgically repaired. Acidosis is a product of poor tissue perfusion and death at the cellular level [50]. Lactic acidosis is a finding associated with cellular anoxia. Free radical release during tissue hypoxia also contributes to overall organ dysfunction and further perpetuates the cascade [51]. The coagulopathy is secondary to dilution, platelet dysfunction, cellular damage, decreased hepatic synthesis of factors, and shunting of proteins away from creating coagulation factors and toward production of acute-phase reactants [51–55]. Hypothermia occurs secondary to decreased metabolism. It is also associated with infusion of cold or chilled blood products and crystalloid, and hypothermia itself contributes to continued perpetuation of coagulopathy [56]. Furthermore it is the mismatch between oxygen delivery and consumption with resultant organ dysfunction that defines the shock state [57]. All three elements of the lethal triad contribute and potentiate the death spiral after substantial bleeding. Interruption of this process is paramount to survival.
Evaluation of Volume Status
Distinguishing compensated shock from impending complete cardiovascular collapse can be difficult. Understanding physiology and volume status on a global scale seems straightforward—it is the clinical application of these principles to the individual patient that creates a conundrum for identifying the degree of shock. Given the application of focused assessment with sonography in trauma (FAST) exam, there has been some interest in examination of the inferior vena cava (IVC) volume during the initial assessment. This is a noninvasive, accurate, and rapid way to assess the patient’s overall volume status and is easy to repeat. The technique has been described as placing the patient in the supine position and angling the probe toward the right shoulder from a subcostal view. The IVC can be measured at the entrance of the hepatic veins. Measuring in expiration appears to yield the most accurate measurement. Several small studies demonstrate that measurements of IVC diameter are incredibly fast, noninvasive, accurate measures to determine if shock is present [58–61]. Of note there can be error in measuring the IVC diameter; when accounting for volume variability, the anterior–posterior measurement has been found to be less precise than measurements taken on the oblique axis [61]. In this manuscript the minor axis was defined as the shorter axis when the IVC was viewed as an ellipse shape in horizontal orientation. Trauma patients were included in the study if they were noted to be hypovolemic on the initial ultrasound (minor axis measurement less than 15 mm, consistently measured one cm below the renal vessels) and if they received a computed tomography (CT) scan of their abdomen to further confirm results within 1 h of their diagnosis of hypovolemia. Expected expansion after fluid resuscitation was approximately 7 mm in the minor axis. It remains to be seen if this technique can be widely applied and reliably instituted as a means to identify patients who are volume depleted or dependent.
The Role of CPR During Resuscitation
One of the great follies occurring during the treatment of hemorrhagic shock is to perform advanced cardiac life support (ACLS) or cardiopulmonary resuscitation (CPR) with the notion that there will be any chance of survival. ACLS/CPR does not appear in the resuscitation algorithm of ATLS simply because it has no purpose there; it has no role in the definitive treatment of hemorrhagic shock [2]. Until the source of the hemorrhage is controlled and intravascular volume restored after hypovolemic arrest, there is no other effective treatment option.
In the pediatric literature, several studies have looked at long-term survival data on children who received ACLS in the field prior to arrival at the treating facility [62]. The majority of non-survivors in this study of blunt trauma mechanisms all had either a head injury or a spinal cord injury, and the causes of death in all cases were secondary to devastating neurologic demise or neurogenic shock with cardiopulmonary collapse. Only two deaths had identifiable sources of hemorrhage, which were unable to be adequately controlled. Of the two who survived out of the total 25 who received CPR either in the field or in the emergency department, both had detectable vital signs during transport, lost vitals in the emergency department, and had protracted periods of CPR. Neither survivor had a head injury. The final conclusions from this data set were that children who received field CPR had poor prognosis and that traumatic hemorrhage, brain injury, and spinal cord injury were likely not treatable in any manner by way of CPR. These results are consistent with previous data showing abysmal outcomes for survivability in children receiving post-traumatic CPR [63, 64].
Emergency Room Thoracotomy
Although residents often consider it a rite of passage to perform the emergency room thoracotomy (ERT) [65], the mature surgeon realizes that the ERT has its place in very few clinical circumstances (Fig. 1.2). With only a 2% overall survival rate in blunt trauma, and a 35% survival rate for patients with a single penetrating, quickly controllable injury and no or brief loss of vitals, a selective approach to deciding which patient qualifies for such an invasive maneuver is mandatory.
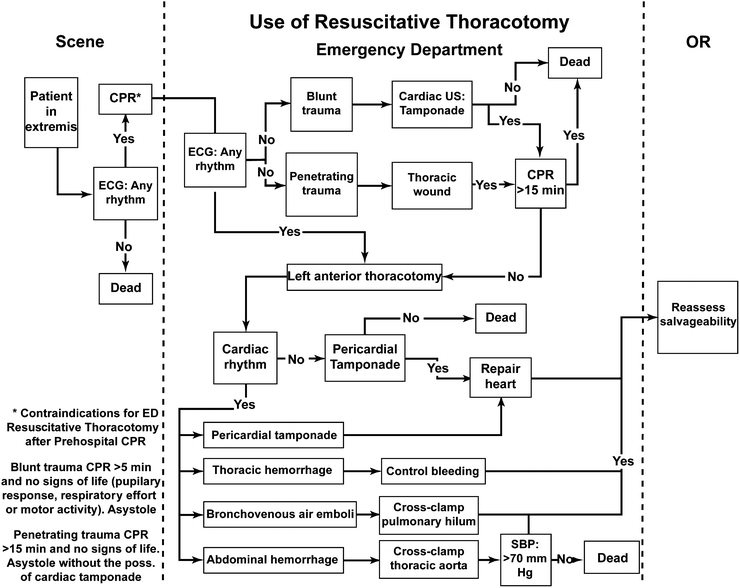
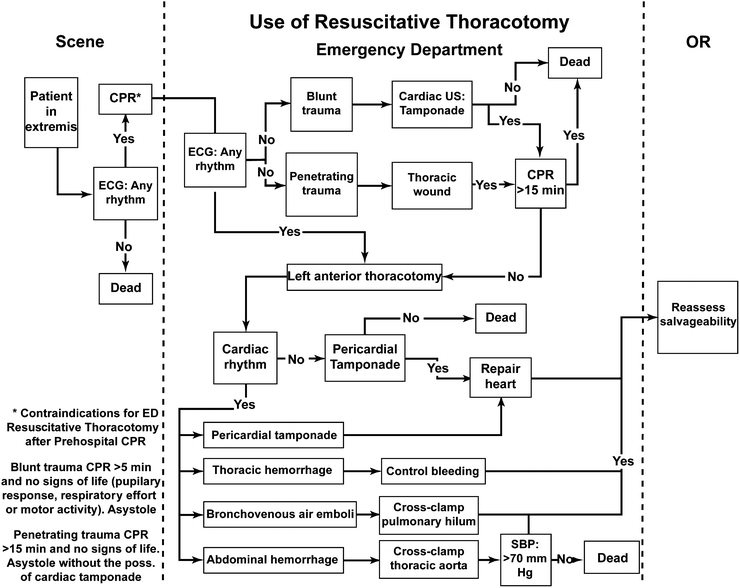
Fig. 1.2
Algorithm showing when a resuscitative thoracotomy should and should not be performed. Reprinted with permission from Mears G, Glickman SW, Moore F, Cairns CB. Data based integration of critical illness and injury patient care from EMS to emergency department to intensive care unit. Curr Opin Crit Care. 2009 Aug;15(4):284–9
In 1993 a small study of 23 retrospectively reviewed cases from UC Davis-Sacramento’s children’s hospital showed that the parameters for ERT and survival rate paralleled those of the adult population with mortality of greater than 96% and more than 85% based on blunt or penetrating mechanism [66]. A more selective approach to the pediatric population is now standard of care.
Once the decision has been made to commit to the ERT, several key maneuvers remain instrumental. The chest should be opened swiftly and efficiently. The skin, subcutaneous tissue, and muscle should be quickly divided at the level of the fourth intercostal space and the superior edge of the rib exposed and then followed to gain appropriate level access to the thoracic cavity. The rib space is held open using a retractor and the inferior pulmonary ligament is divided sharply with scissors up to the inferior pulmonary vein. The lung is then retracted superiorly and anteriorly with the left hand while the right hand traces the posterior ribs to the aorta. The pleural tissue about the aorta is divided sharply or bluntly dissected with a finger and the aortic cross clamp is placed. Care is taken not to injure any intercostal arteries. During this time any blood in the chest is evacuated swiftly. The pericardium can be opened anterior to the phrenic nerve with a plan of how cardiac injury will be controlled during consequent transport to the operating room. The patient should then, upon return of vital signs, be transported to the operating room where definitive surgical management can occur.
Massive Transfusion
Since no factors, other than severe head injury, have ever been identified to correlate with non-survivability, massive transfusion protocols should not be held for an assumption of impending mortality [67]. According to this article, no lab value, no injury severity score (ISS), no demographic data, and no vital sign, singly or grouped, determine a mortality score. A second manuscript from the same group of authors discusses a potential model for predicting mortality at 30 days; however, still there are cautions against using such a model to withhold much-needed blood products during resuscitation [68]. Factors most predictive of 24-h mortality are pH, base deficit, and amount of blood transfused within the initial 6 h. Factors at 30 days that are of significance include age and ISS on admission.
At Memorial Hermann Hospital in Houston, Texas, the massive transfusion protocol is activated for any patient who is suspected to require substantial transfusion, based on any one of the following: heart rate on arrival of more than 120 beats per minute, systolic blood pressure on arrival of less than 90, a positive FAST exam, penetrating or blunt trauma mechanism, or having a requirement for un-crossmatched blood in the emergency room on arrival. These recommendations come from retrospective data comparing predictive scores for massive transfusion. Using these parameters a score of two or greater was found to be 75% sensitive and 86% specific, correlating relatively well without statistical significance to other published scoring systems [69]. The goal of this guideline is to make a continuous supply of six units of packed red blood cells (PRBC), six units of plasma (FFP), and one dose of a six-pack of platelets readily available. After 12 units of PRBC it is advised to check a fibrinogen level and if less than 100 mg/dl to administer ten units of cryoprecipitate. Serial labs are also drawn during the massive transfusion and include lactate, arterial blood gas, rapid thromboelastogram (TEG), coagulation panel, and complete blood count (CBC) with differential and platelet count. It should be noted that a TEG is available within minutes (five for a rapid TEG), whereas the coagulation panel and CBC take more than 45 min to process [70]. Additionally all code three trauma activations, which are the highest acuity patients at Memorial Hermann Hospital, are typed and crossed on arrival so that type-specific blood may be given when available.
Although directed transfusion with specific ratio has never been definitively proven to have advantage [71, 72], a 1:1:1 ratio of FFP:platelets:PRBC is maintained for trauma patients at Memorial Hermann Hospital. Data supporting the 1:1:1 FFP:platelet:PRBC ratio initially came from military literature dating from 2007, which shows an improvement in mortality for patients receiving such ratios [73]. This was later extrapolated in several studies to the civilian population and further propagated in several trauma centers as a new standard of care [74]. A review article from 2010 looked at nine additional observational studies that were published after the 2007 article [75]. While these authors agree that a 1:1:1 ratio seems to be well supported by the retrospective data thus far, they quickly focus attention to the lack of randomized controlled trials on the subject and to the inherent difficulty of maintaining a perfect ratio during a true massive resuscitation. Interestingly, there are no large randomized studies supporting the conventional method of transfusion. Additionally, they identify the prospect of using lab-guided and goal-directed transfusion recommendations, which have not at this point been prospectively studied.
A goal-directed transfusion protocol is a seemingly attractive approach for trauma resuscitation. Originally, massive transfusion protocols were designed to rapidly and reliably provide products to patients who had clinical evidence of substantial hemorrhage. Products and blood were given without a specific ratio until patients either expired or improved clinically. After introduction of the 1:1:1 ratio, which targets the coagulopathy that accompanies massive transfusion, surgeons began to question if transfusion should be automatic or rather if it should be guided by objective data and lab values. One of several manuscripts on goal-directed resuscitation [76] expresses the idea that resuscitation may be more functional and cost effective if lab values, such as TEG, are used to guide decision making during the resuscitation. This concept relies on laboratory reports being ordered, drawn, sent to, and returned from the laboratory in a clinically relevant time frame. Current published data call into question the rapidity with which laboratory values can be utilized in the rapidly bleeding patient. Which reproducible ratio (if any) that a goal-directed resuscitation returns will be interesting to see. There is, at this time however, no consensus on either the 1:1:1 ratio or the use of goal-directed transfusion for resuscitation. These outstanding questions await level 1 data.
Thromboelastogram
The trauma service at the University of Texas at Houston has been using r-TEG consistently in all higher level activations for more than 18 months. The TEG is used to guide decisions on non-massively hemorrhaging patients or patients at risk of hemorrhage. TEG is a plotted graph of the effectiveness of clot formation and breakdown, and is considered more accurate to identify causes of coagulopathy in the trauma patient than is a coagulation panel [77, 78]. A recent Cochrane Review failed to show any mortality difference in patients who are resuscitated using TEG guidance versus those who follow a standardized massive transfusion protocol; however, the authors note that only five of the included nine studies evaluated mortality data as an endpoint [79]. They also note that TEG can potentially reduce the amount of transfusions if interpreted and applied during hemorrhagic shock, but that the data on this point is not definitive [79].
The TEG curves can provide information about all aspects of the clotting system, possibly even the interactions with the endothelium, which is currently an ongoing area of research [80]. The initial part of the TEG, which comprises the R time, or the activated clotting time (ACT), illustrates the amount of time to begin forming a clot (Fig. 1.3). The K time shows how long it takes to reach clot strength and quantitates the clot kinetics [81], whereas the alpha angle and the maximal amplitude (MA) show the rate of clot formation and the absolute clot strength indicating a relationship between fibrinogen and platelets, respectively. A low angle reflects a low fibrinogen concentration; a low MA means that the platelet count or function is reduced and the patient would benefit from platelet transfusion or desmopressin (DDAVP). The LY30 indicates the stability of the clot and the degree of fibrinolysis. The G value shows clot strength or firmness [82].
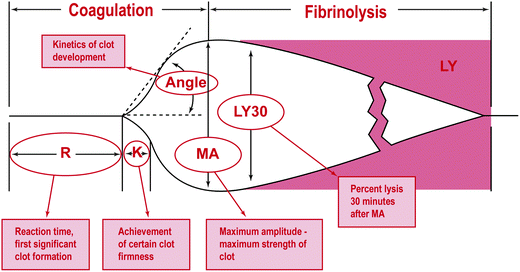
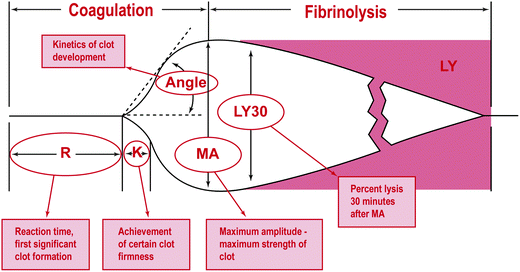
Fig. 1.3
Analytical software graphical representation of a TEG tracing. R: initial time; K: time it takes to reach clot strength; MA: maximal amplitude; LY: lysis. Reprinted with permission from Mark H. Ereth, MD. Uncontrolled bleeding after thoracic aortic aneurysm repair: a case report and interactive discussion. http://www.bloodcmecenter.org
Normal ACT, R time, and K time indicate that clotting factors are intact and functional. Delays in any of these mean that the patient would most benefit from the administration of FFP or factor; additionally it can reflect a patient on heparin or other medication that impairs clotting. The angle and MA reflect platelet function and an increase in either suggests hypercoagulable state, whereas a decrease in either means that the platelets may not be aggregating properly. In patients with an elevated MA there is argument for administration of a daily aspirin or placement of an IVC filter (publication pending) [83]. It has been shown that an MA greater than 68 correlates with an increase in coagulability, predisposing patients to thromboembolism [84]. LY30 greater than 3% has significant consequences of increased mortality and should be treated with amicar or tranexamic acid [85–88].
Damage control resuscitation is a term coined in the military [89, 90]. It is a reproducible strategy with reproducible results and it is automatic and continuous until a physician decides that the shock state has resolved and that hemostasis has been achieved. It describes a resuscitation that uses replacement blood product, rather than crystalloid, for hemorrhagic shock. By limiting the crystalloid infused in the initial resuscitation, patients appear to have less complications and morbidity [91, 92]. There are fewer reports of compartment syndromes, a higher number of abdomens that can be closed after a damage control laparotomy, less acidosis, and less electrolyte disturbances.
Many centers now utilize a strategy of blood product resuscitation and limitation of crystalloid allocation [93]. For example Cotton and colleagues investigated the success of the trauma laparotomy when damage-control resuscitation in a 1:1:1 ratio and limited crystalloid were implemented [94]. This strategy of damage control resuscitation was found to be useful in the field. Patients in the damage control resuscitation group received approximately 10 l less of crystalloid in the first 24 h, had better short- and long-term survival, and showed signs of being less acidodic, less coagulopathic, and less hypothermic on arrival to the ICU than patients who received a traditional resuscitation. The study was a retrospective cohort that examined two similar groups of patients, finding improved morbidity and mortality rates in the group receiving better ratios and colloid. Secondary analyses showed statistically significant differences in multi-organ failure, acute lung and kidney injury, and their effects.
The length of time it takes to get access to FFP plays a role in the success of a massive transfusion protocol [92]. Several studies have examined time factors in receiving product as a way to analyze the effectiveness of a massive transfusion protocol [95–97]. At Memorial Hermann Hospital in Houston the trauma team improved availability of the initial unit of FFP by simply changing the physical location of the thawed FFP from the blood bank to the emergency department (unpublished data). This data shows an improvement in infusion time interval from 56 min to less than 5 min, which is associated with improved outcomes.
Hypertonic Saline
Crystalloid evaluation would not be complete without consideration of hypertonic saline. Hypertonic saline use is pervasive throughout the literature. Prior to the recent explosion of blood product-based resuscitation, crystalloid resuscitation was the standard of care. Hypertonic saline shows some improvement in blood pressure and arguable survival difference for patients who receive it in the pre-hospital setting [98]. There are other studies showing decreased pre-hospital fluid requirements in patients who receive hypertonic during transport [99]. Immunomodulatory effects are enhanced with single administration of 250 ml of hypertonic saline in the initial phase of resuscitation of hemorrhagic shock [100], and this could have additional effects on patients with later discovered head injury [101]. A large study of hypertonic saline showed statistical difference in outcome in pediatric head-injured patients when compared with isotonic fluid administration [102]. Hypertonic saline decreases interstitial pressure and consequently decreases bowel edema, which may be a potential benefit of using it on the patient whose abdomen is still open, as will be discussed later [103, 104]. Animal studies in the 1990s showed that there was no protective effect or difference in outcome for the patient in hemorrhagic shock with a head injury [105]. Since that time several studies examining hypertonic saline as a resuscitative fluid have been terminated secondary to futility and concerns for patient safety [106, 107]. It is still debatable that hypertonic has a physiologic or survival advantage when compared to other crystalloid formulations when used as a primary resuscitation fluid [108].
Complications of Resuscitation
Data from the days when trauma patients were resuscitated with multiple liters of saline prior to receiving their first blood product shows complications more related to the overwhelming volume of crystalloid infused than to the blood and product resuscitation [109–111]. These types of complications include compartment syndromes, high number of abdomens that cannot be closed, and grossly edematous bowel, all secondary to large volume resuscitation [112]. Complications of transfusion-related acute lung injury (TRALI) and transfusion-associated circulatory overload (TACO) are not seen frequently now because the base resuscitative fluid is colloid at lower volume not large volumes of crystalloid [113–115]. Ileus, heart failure, and difficulty with wound healing have all additionally been attributed to over-resuscitation with crystalloid.
All trauma patients who receive a massive resuscitation are at risk of abdominal compartment syndrome. One study claims that there will be an epidemic if crystalloid resuscitations are continued with such fervor and that patients are threatened by secondary compartment syndrome that occurs solely as the result of excessive crystalloid resuscitation during hemorrhagic shock [116]. Abdominal hypertension is defined as any pressure greater than 12 mmHg without evidence of multi-organ failure. Abdominal compartment syndrome is defined as any one of the following: pressure greater than 20 mmHg; progressive, identifiable organ dysfunction; and improvement following decompression. The trauma population is susceptible, even those who lack abdominal injuries and develop elevated pressures simply due to the amount of fluid they receive [112]. In Houston during the late 1990s the resuscitations during the first 24 h for a group of 128 patients requiring decompression for organ dysfunction averaged the following volumes: (26 ± 2 units PRBC, 38 ± 3 l crystalloid). Seven of these cases required urgent non-abdominal operations, where they likely received several additional units of crystalloid or colloid [117].
It is recommended to check bladder pressures and peak inspiratory pressures routinely and aggressively in patients where massive transfusion has taken place [118]. This practice of serially checking bladder pressures, based on observational data, seems to help in the early identification of abdominal hypertension, perhaps staving off the evolution to abdominal compartment syndrome [119]. Decompression can be done with placement of a temporary dressing and later planned closure with evidence of better results and earlier closure [120, 121].
Keeping the abdomen open after a damage control laparotomy also has its disadvantages. It has been shown that ileus and bowel edema prevent advancement of feeds and definitive closure, and that these phenomena are likely related to an ongoing inflammatory response that occurs as a result of the sustained acute resuscitative phase [122–124]. It is additionally unclear whether ileus is a cause or an effect of bowel edema and vice versa [125, 126]. Three percent hypertonic saline running at 30 ml/h during the time that the abdomen is open decreases bowel edema [103]. The mechanism is thought to be due to hydrostatic gut edema induced by overaggressive resuscitation with crystalloid. The hypertonic saline gives a smaller volume of more concentrated solution, and pulls extra edematous fluid from the bowel wall. Success has been shown in the rat and subsequently in the human model.
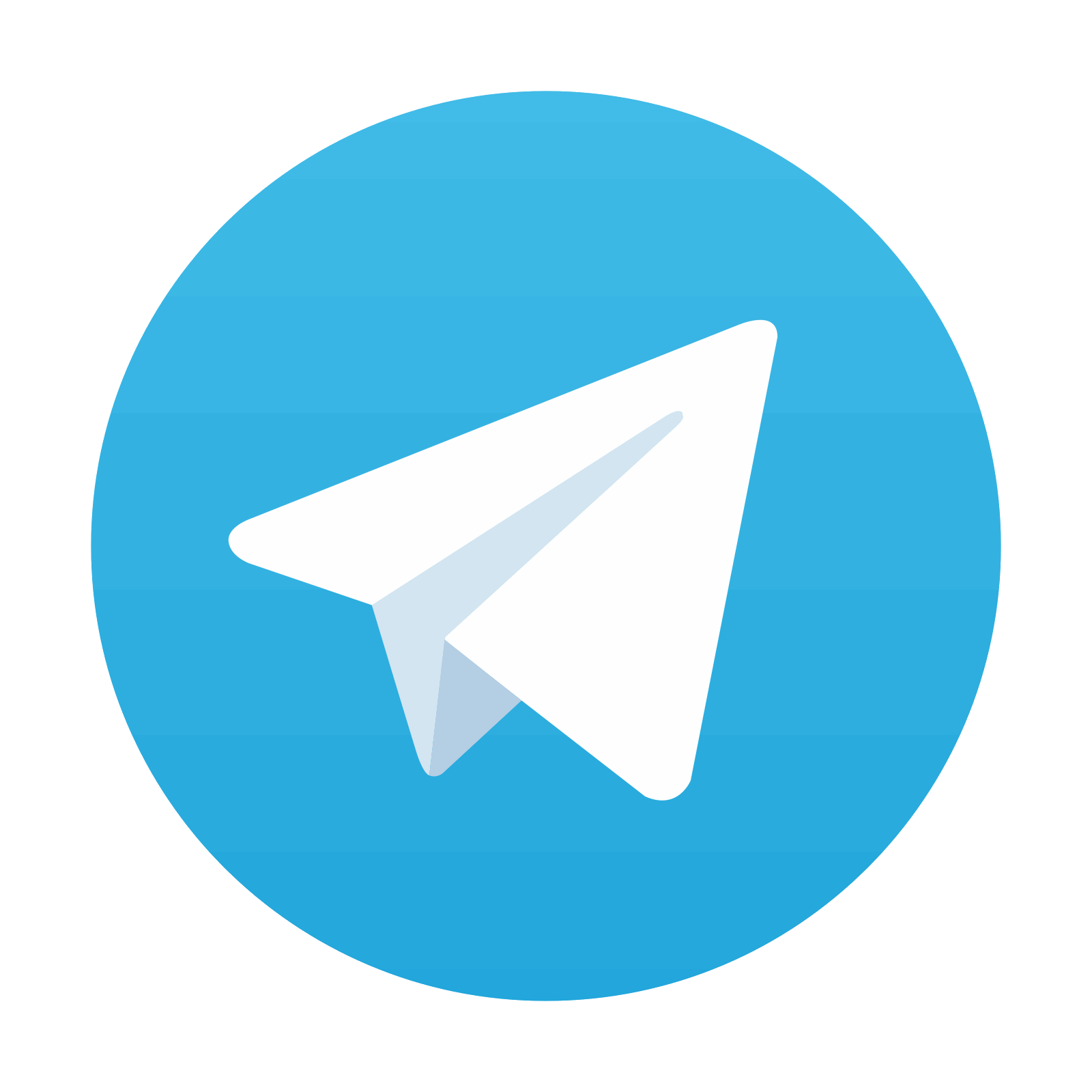
Stay updated, free articles. Join our Telegram channel

Full access? Get Clinical Tree
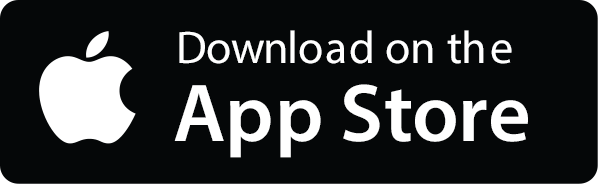
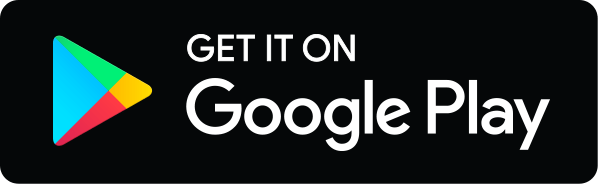