The Allergic Response
Jerrold H. Levy
Key Points










Related Matter
Type 3 Immune Complex Reaction
Anaphylaxis
Introduction

The allergic response represents just one aspect of the pathologic response that the immune system evolved to recognize foreign substances. As part of normal host surveillance mechanisms, a series of cellular and humoral elements oversees foreign surfaces of cell surfaces and molecular structures called antigens to provide host defense. These foreign substances (antigens) consist of molecular arrangements found on cells, bacteria, viruses, proteins, or complex macromolecules.2,3 Immunologic mechanisms (1) involve antigen interaction with antibodies or specific effector cells; (2) are reproducible; and (3) are specific and adaptive, distinguishing foreign substances and amplifying reactivity through a series of inflammatory cells and proteins. The immune system serves to protect the body against external microorganisms and toxins, as well as internal threats from neoplastic cells; however, it can respond inappropriately to cause hypersensitive (allergic) reactions. Life-threatening allergic reactions to drugs and other foreign substances observed perioperatively may represent different expressions of the immune response.2,3
Basic Immunologic Principles
The immune system protects the body from invasion by organisms by recognizing and removing foreign substances called antigens that are molecular structures, usually proteins and/or carbohydrates. However, the body also has mechanisms to tolerate similar molecular configurations of the host (self-tolerance); however, problems arise when the immune system is dysfunctional as in cases of autoimmunity that can give rise to serious diseases including rheumatoid arthritis and lupus. The immune response includes both cell-mediated immunity and humoral immunity. Cell-mediated immunity involves immune cell directed at elimination or destruction of pathogens or cells. Humoral immunity comprises different antibodies and proteins such as complement that can directly or in concert with cellular immunity orchestrate cell injury and destruction. The purpose is to provide host defense mechanisms.
As part of humoral immune responses, protein mediators called cytokines and chemokines are released initially by inflammatory responses to bring other immune cells to the site of the injury or infection, and cause further inflammatory responses and fever, and to increase capillary permeability to allow other immune cells to migrate and translocate to the site of injury. This inflammatory response also causes hemostatic activation and produces pain, erythema, and edema locally and potentially systemically depending on the extent of the injury. Cytokines have an extensive spectrum of inflammatory effects, an issue studied more extensively in sepsis.4 The immune response can be variable in onset from immediate in anaphylaxis, to days, and can remember antigens for many years, especially following immunization.
Table 12-1. Agents Administered During Anesthesia that Act as Antigens | ||||||||||||||
---|---|---|---|---|---|---|---|---|---|---|---|---|---|---|
|
Although this is a simplified review of the immune system, it is important to consider individual aspects of the immune response and their importance below.
Antigens
As mentioned, molecules stimulating an immune response (antibody production or lymphocyte stimulation) are called antigens.5,6 Only a few drugs used by anesthesiologists, such as polypeptides (protamine) and other large macromolecules (dextrans), are complete antigens (Table 12-1). Most commonly used drugs are simple organic compounds of low molecular weight (around 1,000 Da). For such a small molecule to become immunogenic, it must form a stable bond with circulating proteins or tissue micromolecules to result in an antigen (hapten-macromolecular complex). Small molecular weight substances such as drugs or drug metabolites that bind to host proteins or cell membranes to sensitize patients are called haptens. Haptens are not antigenic by themselves. Often, a reactive drug metabolite (i.e., penicilloyl derivative of penicillin) is believed to bind with macromolecules to become antigens, but for most drugs this has not been proved. Some molecular structures in bacteria or fungi are immediately recognized as foreign.
Thymus-derived (T-cell) and Bursa-derived (B-cell) Lymphocytes
The thymus of the fetus differentiates immature lymphocytes into thymus-derived cells (T cells). T cells have receptors that are activated by binding with foreign antigens and secrete mediators that regulate the immune response. The subpopulations of T cells that exist in humans include helper, suppressor, cytotoxic, and killer cells.5,6 The two types of regulatory T cells are helper cells (OKT4) and suppressor cells (OKT8). Helper cells are important for key effector cell responses, whereas suppressor cells inhibit immune function. Infection of helper T cells with a retrovirus, the human immunodeficiency virus, produces a specific increase in the number of suppressor cells. Cytotoxic T cells destroy mycobacteria, fungi, and viruses. Other lymphocytes, called natural killer cells, do not need specific antigen stimulation to set up their role. Both the cytotoxic T cells and natural killer cells take part in defense against tumor cells and in transplant rejection. T cells produce mediators that influence the response
of other cell types involved in the recognition and destruction of foreign substances.5,6
of other cell types involved in the recognition and destruction of foreign substances.5,6
B cells represent a specific lymphocyte cell line that can differentiate into specific plasma cells that synthesize antibodies, a step controlled by both helper and suppressor T-cell lymphocytes. B cells are also called bursa-derived cells because in birds, the bursa of Fabricius is important in producing cells responsible for antibody synthesis.
Antibodies

Table 12-2. Biologic Characteristics of Immunoglobulins | ||||||||||||||||||||||||||||||||||||||||||||||||||||||||||||||||||||||||||||||||||||
---|---|---|---|---|---|---|---|---|---|---|---|---|---|---|---|---|---|---|---|---|---|---|---|---|---|---|---|---|---|---|---|---|---|---|---|---|---|---|---|---|---|---|---|---|---|---|---|---|---|---|---|---|---|---|---|---|---|---|---|---|---|---|---|---|---|---|---|---|---|---|---|---|---|---|---|---|---|---|---|---|---|---|---|---|
|
Five major classes of antibodies occur in humans: IgG, IgA, IgM, IgD, and IgE. The heavy chain determines the structure and the function of each molecule. The basic properties of each antibody are listed in Table 12-2.
Effector Cells and Proteins of the Immune Response
Cells
Monocytes, neutrophils (polymorphonuclear leukocytes [PMNs]), and eosinophils represent important effector cells that migrate into areas of inflammation in response to specific chemotactic factors, including lymphokines, cytokines, and complement-derived mediators. The deposition of antibody or complement fragments on the surface of foreign cells is called opsonization, a process that promotes killing foreign cells by effector cells. In addition, lymphokines and cytokines produce chemotaxis of other inflammatory cells in a manner described in the following sections.5,6 Activation of these cellular process is orchestrated by multiple mechanisms, as best recently reviewed.7
Monocytes and Macrophages
Macrophages regulate immune responses by processing and presenting antigens to effect inflammatory, tumoricidal, and microbicidal functions. Macrophages arise from circulating monocytes or may be confined to specific organs such as the lung. They are recruited and activated in response to microorganisms or tissue injury. Macrophages ingest antigens before they interact with receptors on the lymphocyte surface to regulate their action.
Macrophages synthesize mediators to facilitate both B-lymphocyte and T-lymphocyte responses.
Macrophages synthesize mediators to facilitate both B-lymphocyte and T-lymphocyte responses.
Polymorphonuclear Leukocytes (Neutrophils)
The first cells to appear in acute inflammatory reaction are neutrophils that contain acid hydrolases, neutral proteases, and lysosomes. Once activated, they produce hydroxyl radicals, superoxide, and hydrogen peroxide, which aid in microbial killing.
Eosinophils
The exact function of the eosinophil in host defense is unclear; however, inflammatory cells recruit eosinophils to collect at sites of parasitic infections, tumors, and allergic reactions.
Basophils
Basophils comprise 0.5% to 1% of circulating granulocytes in the blood. On the surface of basophils are IgE receptors, which function similarly to those on mast cells.
Mast Cells
Mast cells are important cells for immediate hypersensitivity responses. They are tissue fixed and located in the perivascular spaces of the skin, lung, and intestine. On the surface of mast cells are IgE receptors, which bind to specific antigens. Once activated, these cells release physiologically active mediators important to immediate hypersensitivity responses (see IgE-mediated Pathophysiology section under Anaphylactic Reactions). Mast cells can be activated by a series of both immune and nonimmune stimuli.
Proteins
Cytokines/Interleukins

Complement
The primary humoral response to antigen and antibody binding is activation of the complement system.9,10 The complement system consists of around 20 different proteins that bind to activated antibodies, other complement proteins, and cell membranes. The complement system is an important effector system of inflammation. Complement activation can be initiated by IgG or IgM binding to antigen, by plasmin through the classic pathway, by endotoxin, or by drugs through the alternate (properdin) pathway (Fig. 12-2). Specific fragments released during complement activation include C3a, C4a, and C5a, which have important humoral and chemotactic properties (see Non-IgE–mediated Reactions section). The major function of the complement system is to recognize bacteria both directly and indirectly by attracting phagocytes (chemotaxis), as well as the increased adhesion of phagocytes to antigens (opsonization), and cell lysis by activation of the complete cascade.9,10
A series of inhibitors regulates activation to ensure regulation of the complement system. Hereditary (autosomal dominant) or acquired (associated with lymphoma, lymphosarcoma, chronic lymphatic leukemia, and macroglobulinemia) angioneurotic edema is an example of a deficiency in an inhibitor of the C1 complement system (C1 esterase deficiency). This syndrome is characterized by recurrent increased vascular permeability of specific subcutaneous and serosal tissues (angioedema), which produces laryngeal obstruction and respiratory and cardiovascular abnormalities after tissue trauma and surgery, or even without any obvious precipitating factor.11 One of the important pathologic manifestations of complement activation is acute pulmonary vasoconstriction associated with protamine administration.1
Effects of Anesthesia on Immune Function

Hypersensitivity Responses (Allergy)
Gell and Coombs first described a scheme for classifying immune responses to understand specific diseases mediated by immunologic processes. The immune pathway functions as a protective mechanism, but can also react inappropriately to produce a hypersensitivity or allergic response. They defined four basic types of hypersensitivity, types I to IV. It is useful first to review all four mechanisms to understand the different immune reactions that occur in humans.
Type I Reactions
Type I reactions are anaphylactic or immediate-type hypersensitivity reactions (Fig. 12-3). Physiologically active mediators are released from mast cells and basophils after antigen binding to IgE antibodies on the membranes of these cells. Type I hypersensitivity reactions include anaphylaxis, extrinsic asthma, and allergic rhinitis.
Type II Reactions
Type II reactions are also known as antibody-dependent cell-mediated cytotoxic hypersensitivity or cytotoxic reactions (antibody-dependent cell-mediated cytotoxic) (Fig. 12-4). These reactions are mediated by either IgG or IgM antibodies directed against antigens on the surface of foreign cells. These antigens may be either integral cell membrane components (A or B blood group antigens in ABO incompatibility reactions) or haptens that absorb to the surface of a cell, stimulating the production of antihapten antibodies (autoimmune hemolytic anemia). The cell damage in type II reactions is produced by (1) direct cell lysis after complete complement cascade activation, (2) increased phagocytosis by macrophages, or (3) killer T-cell lymphocytes producing antibody-dependent cell-mediated cytotoxic effects. Examples of type II reactions in humans are ABO-incompatible transfusion reactions, drug-induced immune hemolytic anemia, and heparin-induced thrombocytopenia.
Type III Reactions (Immune Complex Reactions)

Type IV Reactions (Delayed Hypersensitivity Reactions)
Type IV reactions result from the interactions of sensitized lymphocytes with specific antigens (Fig. 12-6). Delayed hypersensitivity reactions are mainly mononuclear, manifest in 18 to 24 hours, peak at 40 to 80 hours, and disappear in 72 to 96 hours. Antigen–lymphocyte binding produces lymphokine synthesis, lymphocyte proliferation, and generation of cytotoxic T cells, and attracting macrophages and other inflammatory cells. Cytotoxic T cells are produced specifically to kill target cells that bear antigens identical with those that triggered the reaction. This form of immunity is important in tissue rejection, graft-versus-host reactions, contact dermatitis (e.g., poison ivy), and tuberculin immunity.
Table 12-3. Recognition of Anaphylaxis During Regional and General Anesthesia | ||||||||||||||||||||||||||||||||||||||||||||||||||||||||||||||||||
---|---|---|---|---|---|---|---|---|---|---|---|---|---|---|---|---|---|---|---|---|---|---|---|---|---|---|---|---|---|---|---|---|---|---|---|---|---|---|---|---|---|---|---|---|---|---|---|---|---|---|---|---|---|---|---|---|---|---|---|---|---|---|---|---|---|---|
|
Intraoperative Allergic Reactions

Anaphylactic Reactions
IgE-mediated Pathophysiology

may be unknown to the patient. On reexposure, binding of the antigen to bridge two immunospecific IgE antibodies found on the surfaces of mast cells and basophils releases stored mediators, including histamine, tryptase, and chemotactic factors.13,16,17 Arachidonic acid metabolites (leukotrienes and prostaglandins), kinins, and cytokines are subsequently synthesized and released in response to cellular activation.18 The released mediators produce a symptom complex of bronchospasm and upper airway edema in the respiratory system, vasodilation and increased capillary permeability in the cardiovascular system, and urticaria in the cutaneous system. Different mediators are released from mast cells and basophils after activation.
Chemical Mediators of Anaphylaxis
Histamine stimulates H1, H2, and H3 receptors. H1 receptor activation releases endothelium-derived relaxing factor (nitric oxide) from vascular endothelium, increases capillary permeability, and contracts airway and vascular smooth muscle.16,19 H2 receptor activation causes gastric secretion, inhibits mast cell activation, and contributes to vasodilation. When injected into skin, histamine produces the classic wheal (increased capillary permeability producing tissue edema) and flare (cutaneous vasodilation) response in humans.20,21 Histamine undergoes rapid metabolism in humans by the enzymes histamine N-methyltransferase and diamine oxidase found in endothelial cells.
Peptide Mediators of Anaphylaxis
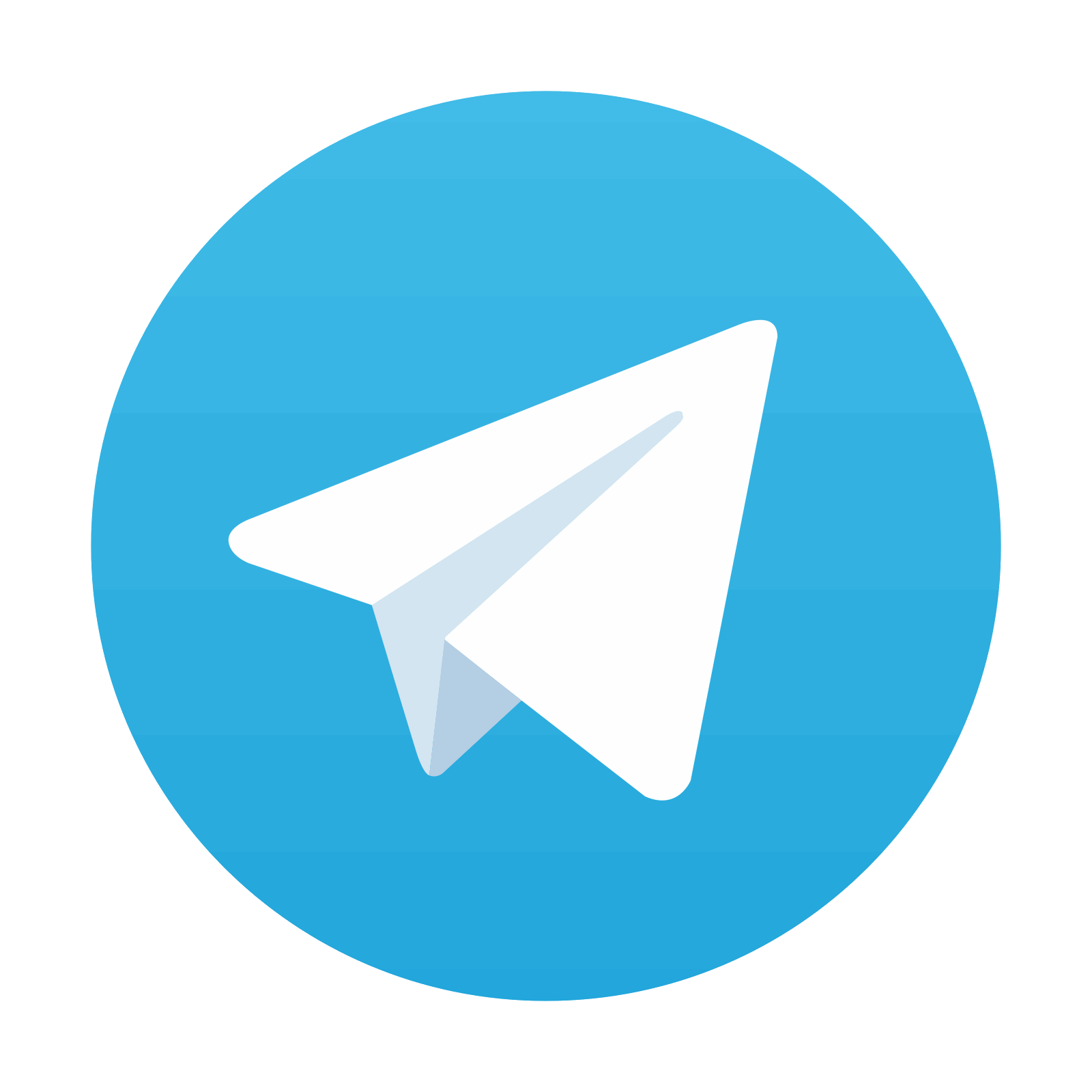
Stay updated, free articles. Join our Telegram channel

Full access? Get Clinical Tree
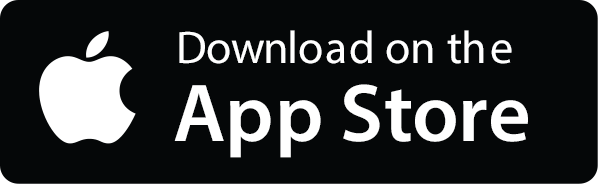
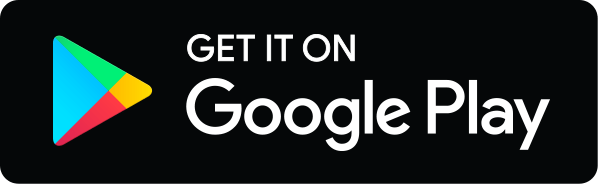
