Chapter 69 Tests of Kidney Function in Children
Renal dysfunction and injury are not unusual in the critically ill child and may develop as a consequence of the underlying disease process (e.g., hypoperfusion secondary to sepsis, progressive glomerulonephritis) or its therapy (e.g., aminoglycoside toxicity). The ability to accurately, precisely, and rapidly assess renal function is essential for the early detection of acute kidney injury (AKI), for dose adjustments of medications excreted by the kidney, in risk assessment for imaging studies involving intravenous contrast agents or gadolinium, and for monitoring for medication-related nephrotoxicity. Until recently, a consensus for defining acute kidney injury (AKI) did not exist. AKI is now recognized to encompass a spectrum of disease ranging from mild, reversible, renal dysfunction to severe, potentially irreversible organ failure with need for dialysis support. In 2004, the Acute Dialysis Quality Initiative proposed the RIFLE (Risk, Injury, Failure, Loss, and End Stage Renal Disease) classification scheme for defining AKI in adults based on changes in serum creatinine or urine output.1 This has since been modified for use in children (pRIFLE), in whom the severity of AKI is stratified based on changes in estimated GFR or urine output (Table 69-1).2 The true incidence of AKI in the pediatric intensive care unit (PICU) is not well established due to the absence of a consensus definition, but recent studies suggest it is increasing.3 Despite advances in pediatric critical care and dialysis, morbidity and mortality rates associated with AKI remain high.4 Therapeutic intervention trials for AKI have had disappointing results, which can be attributed in part to delays in diagnosis as well as to the heterogeneity of patients enrolled.5,6 Earlier recognition and intervention for AKI may provide a greater potential for recovery and/or stabilization of renal function. However, the early detection of impending AKI is severely limited by the lack of sensitive and specific tools currently available to assess renal function.
Table 69–1 Pediatric Modified RIFLE Criteria
Estimated CCl | Urine output | |
---|---|---|
Risk | eCCl decrease by 25% | <0.5 mL/kg/h for 8 h |
Injury | eCCl decrease by 59% | <0.5 mL/kg/h for 16 h |
Failure | eCCl decrease by 75% or eCCl <35 mL/min/1.73 m2 | <0.5 mL/kg/h for 24 h or anuric for 12 h |
Loss | Persistent failure >4 wk | |
End stage | End-stage renal disease (persistent failure >3 mo) |
eCCl, Estimated creatinine clearance.
Modified from Akcan-Arikan A, Zappitelli M, Loftis LL, et al: Modified RIFLE criteria in critically ill children with acute kidney injury, Kidney Int 71(10):1034, 2007.
Assessment of Glomerular Function and Injury
Glomerular filtration rate (GFR) is considered the best overall indicator of kidney function but remains challenging to accurately and efficiently measure in clinical practice. Functionally, the total kidney GFR is determined by the cumulative number of nephrons and the GFR within each nephron (single nephron glomerular filtration rate, or SNGFR). Conceptually, it represents the volume of plasma that could be completely cleared of a substance per unit of time. Kidney function may therefore decline due to hypoperfusion, resulting in a decrease in SNGFR, or due to nephron injury, resulting in fewer functioning nephrons. The kidney has a certain degree of reserve and attempts to adapt to nephron loss by increasing the SNGFR of the remaining nephrons, thus maintaining total kidney GFR initially and masking early renal injury. Indeed, by the time the GFR actually falls, significant injury has already occurred. Loss of this renal reserve is one of the earliest manifestations of renal injury but is even more challenging to measure than GFR.7–9
Glomerular filtration is a dynamic variable that can fluctuate in a given individual by as much as 7% to 8% from day to day based on differences in hydration status and protein consumption.10,11 GFR is also influenced by age, gender, and body size and therefore varies between individuals as well. Therefore, to facilitate comparison of GFR amongst children and adults of considerably different size, absolute GFR is normalized to body surface area (BSA), which correlates well with kidney weight, the most direct standard of reference.12 Appreciation of the maturational increase in GFR that occurs during infancy is also necessary for proper assessment of kidney function in children. At birth, the mean GFR is quite low (20.3 mL/min/1.73 m2 in term infants), due to renal immaturity, but yet sufficient to meet the metabolic demands of a healthy infant. The GFR doubles within the first 2 weeks of life and then continues to gradually increase to reach a mean of 77 mL/min/1.73 m2 between 1 and 6 months of age and adult levels of 120 to 130 mL/min/1.73 m2 by approximately 2 years of age (Table 69-2).13
Table 69–2 Normal GFR Values for Children
Age | GFR (mL/min/1.73 m2) | Range (mL/min/1.73 m2) |
---|---|---|
Preterm (<34 wk) | ||
2–8 days | 11 | 11–15 |
4–28 days | 20 | 15–28 |
30–90 days | 50 | 40–65 |
Term (>34 wk) | ||
2–8 days | 39 | 17–60 |
4–28 days | 47 | 26–68 |
30–90 days | 58 | 30–86 |
1–6 mo | 77 | 39–114 |
6–12 mo | 103 | 49–157 |
12–19 mo | 127 | 62–191 |
2–12 yr | 127 | 89–165 |
Modified from Heilbron DC, Holliday MA, al-Dahwi A, et al: Expressing glomerular filtration rate in children, Pediatr Nephrol 5(1):5-11, 1991.
Renal Clearance Techniques
Inulin
The renal clearance of inulin remains the gold standard for measuring GFR. Inulin is an uncharged 5.2 kDa polymer of fructose that possesses many characteristics of an ideal filtration marker. It is not protein-bound and is freely filtered at the glomerulus without being reabsorbed, secreted, or metabolized by the kidney. Further, it is eliminated exclusively by the kidney.14 The filtered load of inulin (GFR × Pin) is therefore equal to its urinary excretion (Uin × V) where Pin and Uin are the plasma and urine concentrations of inulin (mg/dL), respectively, and V is the urine flow rate (mL/min). The renal clearance of inulin (Cin) can be calculated:
When used for children, however, this must be scaled for BSA:
Iothalamate
Iothalamate (MW 614 Da) is also freely filtered by the glomerulus and has been studied extensively as an alternative exogenous marker for GFR measurements. Unlike inulin, however, iothalamate has some protein binding (<8%) and proximal tubular secretion (10%) raising concern for overestimation of GFR. Reported correlations between iothalamate and inulin renal clearances in the literature have been variable, with some studies demonstrating good correlation, whereas most suggest that iothalamate overestimates inulin clearance.15–17
Creatinine Clearance
Clearance studies that make use of endogenous markers such as serum creatinine obviate the need for a constant infusion and make the study more amenable to clinical practice. However, the problems associated with timed urine collections persist and accordingly limit the usefulness of this technique, particularly in children. Further, creatinine is a flawed filtration marker. Although it is predominantly eliminated by glomerular filtration, a small but variable amount (~10%) is eliminated by tubular secretion. As renal function deteriorates, the proportion of secreted to filtered creatinine progressively increases, leading to an overestimation of GFR and making creatinine clearance a more unreliable measure of GFR.11 Administration of oral cimetidine beginning 2 days prior to the study can at least partially circumvent this issue by inhibiting the tubular secretion of creatinine.18 Although timed urine collections are still necessary, they are typically obtained over a 2-hour period facilitating performance in a monitored clinic setting.
Guidelines from the National Kidney Foundation no longer recommend routine performance of creatinine clearance studies to estimate GFR, as prediction formulas are now felt to be more accurate.19 Nonetheless, creatinine clearance-based studies can still be helpful when assessing renal function in individuals with atypical body composition (e.g., anorexia, malnutrition) or dietary intake (e.g., vegetarian diet).20
Plasma Disappearance Techniques
Plasma disappearance techniques further simplify the measurement of GFR and avoid the need for urine collections. They are most commonly performed using a single intravenous injection technique, although constant infusion and subcutaneous protocols are also available.15,21,22 Serial blood samples collected at specified times over a several-hour period following injection of the filtration marker are used to generate a plasma disappearance curve. This curve can be well approximated by a double exponential curve that is characterized by an initial “fast” curve and a later “slow” curve as illustrated in Figure 69-1. The initial “fast” curve represents the distribution phase and reflects renal elimination as well as diffusion of the marker into the extravascular space, whereas the late “slow” curve reflects only the renal elimination phase. Renal clearance can be calculated by dividing the delivered dose by the entire area under the plasma disappearance curve.23 Modification from a two-compartment model to a one-compartment model focusing on the renal elimination phase simplifies the procedure, reducing the number of required blood specimens to two. GFR can then be derived from the slope of the slow curve but requires incorporation of a correction factor to compensate for overestimation of the GFR, which results from exclusion of the area under the fast curve.23–26 Appropriate timing of the specimens is critical to ensure an accurate estimation of GFR. The first sample must be obtained after the marker has equilibrated, typically 2 hours after injection. The second specimen is obtained approximately 5 hours after injection but, in subjects with more advanced chronic kidney disease, this may need to be delayed further to minimize overestimation of the GFR, which can result from an inaccurate depiction of the lower slope of the plasma disappearance curve.25 The validity of the single-compartment model may also be compromised in edematous states where the volume of distribution is increased, or with extravasation of the tracer at the injection site, as this decreases the actual dose delivered.24 Unfortunately, when performed correctly, plasma disappearance studies are time-consuming and perhaps too complex for routine clinical care.
Radioisotopes
Plasma clearance studies are most commonly performed with radioisotopes that are more readily available and easier to assay than inulin. Their major disadvantage is, of course, radiation exposure, which limits their use, particularly in children.27 51Cr-EDTA, 99mTc-DTPA, and 125I-Iothalamate have been extensively studied and compared to inulin renal clearance.15,28 All are low-molecular-weight compounds that are freely filtered by the glomerulus but which differ slightly in protein binding and renal handling. 51Cr-EDTA (292 Da), appears to have little protein binding, and its plasma clearance correlates well with inulin clearances. It is commonly used in Europe but is unavailable in the United States.25 99mTc-DTPA (393 Da) is most frequently used in the United States but demonstrates variable accuracy in GFR measurements based on product source, differences in protein binding, and potential dissociation of the chelate (t1/2 = 6 hours) during the study.29 125I-Iothalamate (614 Da) is a high-osmolar anionic contrast agent with some protein binding that also undergoes active secretion by the proximal tubule, contributing to overestimation of plasma clearance compared to inulin or EDTA.17 Nuclear GFR studies can also be performed using a gamma camera, which measures the renal uptake of the tracer 2 to 3 minutes after injection and which can provide information regarding differential kidney function; however, the estimated GFR obtained this way is not as accurate as with the plasma-sampling technique.25 To reduce radiation exposure, plasma clearance studies can also be performed using nonradiolabeled iothalamate which can be measured by high-performance liquid chromatography (HPLC).30, 31
Iohexol
Iohexol is emerging as an excellent alternative to inulin and radioisotopes for clearance studies. Iohexol (Omnipaque) is a low-molecular-weight (821 Da), nonionic, low-osmolar intravenous contrast agent used routinely in the United States for radiologic studies at doses appreciably higher than that required for GFR studies.32,33 It is almost entirely eliminated through glomerular filtration and is not reabsorbed, secreted, or metabolized by the kidney, thus fulfilling many of the desired traits of an ideal filtration marker. Further, it has minimal protein binding and negligible extrarenal elimination even in advanced chronic kidney failure.32,34 Though it can easily be measured by x-ray fluorescence or high-performance liquid chromatography, the latter is more sensitive and, therefore, requires a significantly lower dose of iohexol for the study.33,35 Iohexol has been safely used for clearance studies for many years in Scandinavia.32,36,37 Its excellent safety profile has recently been confirmed in the United States in over 1000 GFR estimations performed as part of the Chronic Kidney Disease in Children (CKiD) study, a multicenter, prospective, observational, cohort study of the progression of chronic renal failure.26,38 (G.J.S., unpublished observations). Iohexol plasma clearance results appear to be comparable to renal inulin clearance and plasma EDTA disappearance studies across a broad range of GFR.33,39,40
Plasma Markers
Creatinine
Serum creatinine is the most commonly used laboratory study to assess renal function in clinical practice. It is simple, convenient, and practical—requiring a single blood sample—and therefore well suited for serial examination. However, the relationship between serum creatinine and GFR is quite complex, being influenced by several factors other than GFR. Therefore, at best, creatinine provides a crude estimate of the GFR. As illustrated in Figure 69-2, serum creatinine bears an inverse, nonlinear relationship with GFR and lacks sensitivity to acute and small changes in GFR. Notably, at low levels of serum creatinine corresponding to normal renal function, a substantial decrease in GFR may occur before being reflected by even a small increase in serum creatinine. In contrast, at higher levels of serum creatinine associated with renal failure, the same absolute rise in creatinine reflects a much smaller loss of remaining renal function. To a first approximation, every doubling of the serum creatinine represents a 50% decline in remaining GFR.
Ideally, an endogenous marker such as creatinine can serve as a useful surrogate for GFR if it is produced at a constant rate and eliminated only via the kidney at a rate equivalent to its production rate such that a steady state exists. Figure 69-3 illustrates the relationship between the plasma level of an endogenous filtration marker, its generation (from cells and diet), and its elimination (renal and extrarenal). Ideally, elimination of the marker must occur exclusively by glomerular filtration. The serum level of the marker would then be expected to rise with renal impairment. Serum creatinine, however, does not strictly fulfill these criteria. Although creatinine production is relatively constant, it varies amongst and within individuals.41 It is derived from the nonenzymatic dehydration of muscle creatine and hence is highly dependent on muscle mass. Consequently, in children, creatinine generation fluctuates with growth in addition to diet and illness, as seen with adults.41 The reference range for serum creatinine levels representing normal GFR will thus vary with age, size, and gender after puberty. The relationship between GFR and serum creatinine is therefore particularly complex in children. Maturational changes in serum creatinine and GFR do not parallel one another. GFR is physiologically low at birth, whereas serum creatinine is elevated; however, because of fetal-maternal placental equilibration of creatinine, the elevated creatinine is not indicative of the infant’s renal function but rather the mother’s. Following birth, GFR steadily increases, reaching adult levels over the next 2 years. Serum creatinine, on the other hand, declines over the first few weeks, becoming reflective of the infant’s renal function. The creatinine level then remains stable until approximately 2 years of age as muscle mass accrues proportionally to the increase in GFR. Beyond 2 years of age, when GFR per BSA has fully matured, the ongoing accretion of muscle results in a progressive rise in serum creatinine until adolescence, when adult levels are achieved (0.7 mg/dL for adolescent females and 0.9 mg/dL for adolescent males). Superimposition of a severe or chronic illness associated with malnutrition and muscle wasting makes the interpretation of GFR from serum creatinine alone even more difficult in the pediatric patient. For example, at first glance, maintenance of a stable creatinine in a patient with a prolonged ICU course may be reassuring for preservation of renal function; however, if significant muscle atrophy has occurred, this actually suggests deterioration of renal function.
The second requirement, that excretion of the marker occurs only by glomerular filtration, is also flawed in the case of creatinine. Although the majority of serum creatinine is eliminated by glomerular filtration, a small but unpredictable amount is eliminated by tubular secretion and gastrointestinal degradation. Proximal tubular secretion of creatinine typically accounts for approximately 10% of its elimination, although considerable inter- and intra-individual variability exists.42 At normal levels of GFR, the impact of tubular secretion on GFR is minimal. However, with deteriorating renal function, the proportion of secreted versus filtered creatinine increases, resulting in a lower serum creatinine than predicted for the true level of GFR, thus decreasing the sensitivity for serum creatinine to detect mild decreases in renal function.42 A similar phenomenon occurs in the setting of moderate-to-severe renal failure, when the bacterial degradation of creatinine within the gastrointestinal tract can become clinically significant, leading to a decrease in serum creatinine concentration.43,44 Failure to recognize the influence of tubular secretion and gastrointestinal elimination on serum creatinine values can result in overestimation of renal function and may lead to higher, inappropriate dosing of medications. Conversely, in patients with advanced kidney failure, administration of medications (e.g., cimetidine, trimethoprim) that inhibit the tubular secretion of creatinine or administration of antibiotics that mitigate the gastrointestinal degradation of creatinine may result in an elevation of serum creatinine and subsequent underestimation of GFR without any true change in renal function. If not appreciated, this may be misconstrued as worsening renal function and lead to potential underdosing of medications.
Third, the requirement for a steady state cannot be overemphasized. When GFR abruptly declines with AKI, the production rate of creatinine exceeds its clearance rate leading to a gradual rise in serum creatinine that lags behind the true GFR. (Figure 69-4) During this period, serum creatinine does not accurately reflect the true GFR, which is unknown. Nonetheless, serum creatinine is still typically used to crudely estimate renal function as better alternatives do not currently exist. However, it must be recognized that use of the serum creatinine or creatinine-based estimating equations (see below) to estimate GFR prior to the establishment of a new steady state will result in overestimation of the renal function. Similarly, during the recovery phase of AKI, serum creatinine cannot be used to estimate GFR as it will again lag behind the true GFR until the kidney clears the accumulated creatinine and reaches a new steady state. Use of serum creatinine to estimate GFR during the recovery phase of AKI will therefore result in an underestimation of true GFR.
< div class='tao-gold-member'>
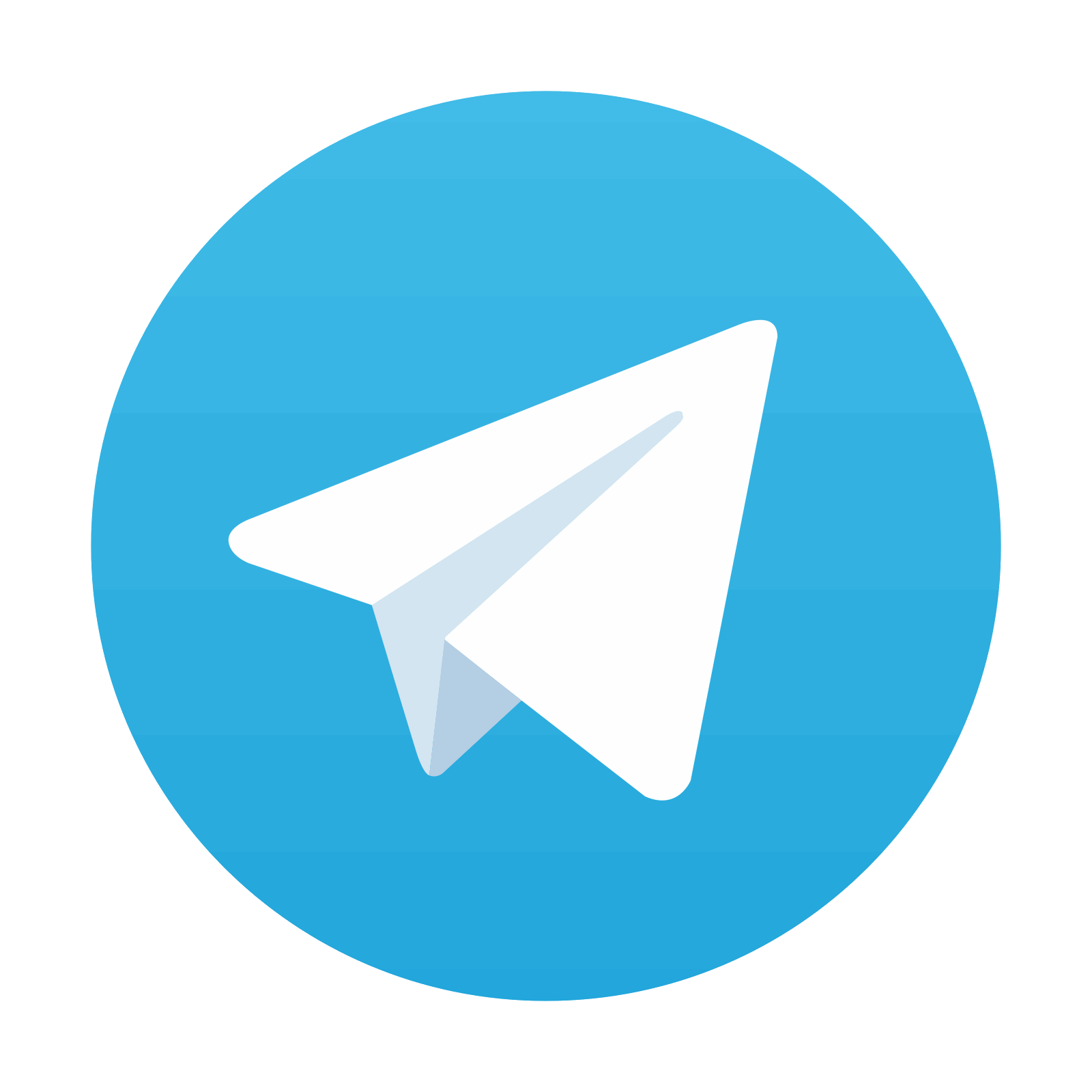
Stay updated, free articles. Join our Telegram channel

Full access? Get Clinical Tree
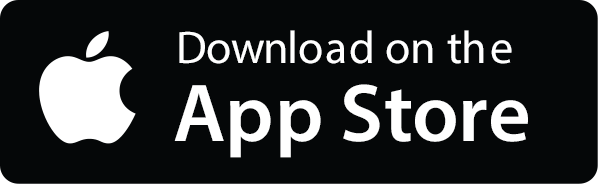
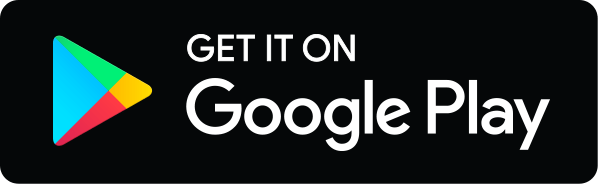