Figure 6.1. Vertebral column, lateral (A) and posterior (B) views, illustrating the cervical, thoracic, lumbar, sacral, and coccygeal segments. Note the curvatures, intervertebral foramina, and interlaminar spaces. (Adapted from Cousins MJ, Bridenbaugh LD, eds. Neural blockade in clinical anesthesia and management of pain, 3rd ed. Philadelphia: Lippincott Williams & Wilkins, 1998:205.)
3. The vertebral column has characteristic curves in the lumbar and thoracic regions that (Figure 6.4), in conjunction with patient position and baricity of local anesthetics, influence the distribution of local anesthetics within the subarachnoid space in patients placed in the supine horizontal position. Local anesthetic solution injected at the peak height of the lumbar anterior convexity (lumbar lordosis) will distribute both caudad and cephalad to varying degrees depending on the baricity of the local anesthetic. The cephalad spread of hyperbaric local anesthetic solutions is limited to the mid-to-upper thoracic dermatomes by encouraging pooling within the thoracic concavity (thoracic kyphosis).
Figure 6.2. Typical lumbar vertebra illustrating superior and lateral views of the anterior vertebral body, the elements that form the vertebral arch (the paired pedicles and paired lumina), and single midline spinous process. Note the superior and inferior vertebral notches of the adjoining pedicles, which form the intervertebral foramen. (Adapted from Moore KL, Dalley AF. Clinically oriented anatomy, 5th ed. Philadelphia: Lippincott Williams & Wilkins, 2006:480.)
Figure 6.3. Sagittal section of vertebral column illustrating the supporting ligaments and their bony attachments. The interspinous ligaments connect adjacent spinous processes, and the ligamentum flavum connects adjacent lamina. (Adapted from Cousins MJ, Bridenbaugh LD, eds. Neural blockade in clinical anesthesia and management of pain, 3rd ed. Philadelphia: Lippincott Williams & Wilkins, 1998:205.)
Figure 6.4. Normal curvature of the spinal column in the supine horizontal position. Hyperbaric solutions injected at the peak of lumbar lordosis will distribute (through gravity) to the lower sacral and thoracic concavities. (Adapted from Raj PP. Handbook of regional anesthesia. New York: Churchill Livingstone, 1985:225.)
B. Meninges. The spinal meninges consist of three membranes (the dura mater, arachnoid mater, and pia mater) that in conjunction with the CSF in the intrathecal space envelop, support, and protect the spinal cord and nerve roots (Figure 6.5).
1. The spinal dura mater (“tough mother”) is the outermost and thickest meningeal membrane and is composed primarily of collagen fibrils, interspersed with elastic fibers and ground substance in an anatomic arrangement that allows ready passage of drugs (1). Therefore, the dura has been incorrectly assumed to be the primary barrier to diffusion of epidurally administered drugs into the subarachnoid space. The dura mater forms the dural sac, which is a long tubular sheath contained within the surrounding spinal canal that extends from the foramen magnum to the lower border of the second sacral vertebra, where it fuses with the filum terminale. The dura extends laterally along the spinal nerve roots, becoming continuous with connective tissue of the epineurium of the spinal nerve at the level of the intervertebral foramina.
2. The arachnoid mater is closely applied to the inner surface of the dura mater, and is composed of overlapping layers of flattened epithelial-like cells that are connected by frequent tight junctions and occluding junctions (2). Although much thinner than the dura, it is the anatomic arrangement of the arachnoid that accounts for the vast majority of the resistance to drug diffusion through the spinal meninges.
3. The pia mater closely invests the surface of the spinal cord and nerve roots and is composed of three to six layers of cells. There is underlying subpial tissue composed primarily of collagen that separates the pial cellular layer from the neural tissues. Scanning electron photomicrograph examination demonstrates fenestrations on the pial surface of the spinal cord and nerve roots that allow direct contact with the subarachnoid space (3). The intrathecal (or subarachnoid) space, which lies between the arachnoid mater and the pia mater, is the target compartment for spinal anesthesia. Additionally, the spinal nerve roots and rootlets traverse the intrathecal space, allowing for local anesthetic uptake. The pia mater extends to the tip of the spinal cord where it becomes the filum terminale, which anchors the spinal cord to the sacrum (Figure 6.5).
C. Spinal cord
1. The spinal cord is a cylindrical structure that gives rise to 31 pairs of spinal nerves, which arise from segments of the spinal cord specified by the intervertebral foramina through which the spinal nerves exit the spinal canal. Each spinal cord segment gives rise to paired ventral motor roots and paired dorsal sensory roots, which cross the intrathecal space and traverse the dura mater separately, uniting in or close to the intervertebral foramina to form the corresponding paired mixed spinal nerves. Although consistently larger than the corresponding ventral nerve roots, anatomic studies have demonstrated that dorsal nerve roots commonly divide into two to three separate bundles upon exiting the spinal cord, whereas most ventral nerve roots exit as a single bundle (4). Additionally, as the dorsal nerve root bundles course further laterally, they further subdivide into as many as one to ten fascicles before the dorsal root becomes the dorsal root ganglion (5). Therefore, the larger, but typically multistranded dorsal nerve roots offer a substantially larger surface area for local anesthetic uptake compared to the smaller, single ventral nerve roots. This anatomic arrangement may partially explain the relatively faster onset and ease of obtaining sensory blockade versus motor blockade.
Figure 6.5. Lumbosacral spinal cord and the spinal meninges (dura, arachnoid, and pia). Note also the terminal portion of the spinal cord (conus medullaris) and the nerve roots of the lower lumbar and sacral spinal cord segments, giving rise to the cauda equina. (Adapted from Cousins MJ, Bridenbaugh LD, eds. Neural blockade in clinical anesthesia and management of pain, 3rd ed. Philadelphia: Lippincott Williams & Wilkins, 1998:209.)
2. The portion of the spinal cord that gives rise to paired nerve roots and spinal nerves is called a spinal cord segment. The skin area supplied by a given spinal nerve and its corresponding cord segment is called a dermatome (Figure 6.6). Because measuring local anesthetic concentration within the subarachnoid space is impractical, and dermatomes can be considered the sensory projections of the spinal cord segments, qualitative assessment of the loss of afferent sensory functions (such as temperature, pinprick, and touch) provides an indirect, but clinically useful estimate of local anesthetic distribution within the intrathecal space, and more importantly, an estimate of the extent of surgical anesthesia.
3. In adults, the spinal cord is shorter than the vertebral column and typically, the caudal extent (known as the conus medullaris [Figure 6.5]) most commonly extends to the lower third of the first lumbar vertebral body, but may be as low as the upper third of the third lumbar vertebral body (6). Therefore, attempting spinal anesthesia at or above the L2-3 intervertebral space (IVS) may potentially increase the risk of mechanical trauma (with the spinal needle) to the spinal cord in a small number of patients.
Figure 6.6. Sensory dermatomes. (Adapted from Agur AMR, Lee MJ, eds. Grant’s atlas of anatomy, 10th ed. Philadelphia: Lippincott Williams & Wilkins, 1999:296.)
4. Because the spinal cord is shorter than the vertebral column, there is progressive obliquity of the lower thoracic, lumbar, and sacral spinal nerve roots, which makes them travel increasingly longer distances within the subarachnoid space (from their spinal cord segments of origin) to the intervertebral foramina through which they exit as the corresponding spinal nerve. The collection of spinal nerve roots within the intrathecal space caudal to the conus medullaris is collectively termed the cauda equina due to it resemblance to a horse’s tail (Figure 6.5). The enlargement of the intrathecal space containing the cauda equina is termed the lumbar cistern. It is within the lumbar cistern where local anesthetics are initially injected for spinal anesthesia.
D. Surface anatomy
1. When preparing for spinal anesthesia, it is critical to accurately identify surface landmarks on the patient. Identification of the lumbar vertebral (or more correctly, intervertebral space level) level is the initial step during the performance of spinal anesthesia. The desired level is determined by inspection and palpation of surface landmarks. A line connecting the iliac crests (the intercristal or Tuffier’s line) most commonly intersects the vertebral column at the L4-5 IVS (Figure 6.7) (6). There is substantial patient variability and the intercristal line may intersect with vertebral column as cephalad as the L3-4 IVS and as caudad as the L5-S1 IVS (Figure 6.8). Additionally, even experienced anesthesiologists correctly identify the IVS only 30% of the time, and the actual level of the IVS was one space higher than estimated in 50% of patients (7). A recent ultrasonographic investigation increased the accuracy of identifying the correct IVS to 70% and confirmed the low accuracy (30%) of correctly identifying the correct IVS by palpation of external bony landmarks (8). On the basis of the anatomic and clinical data mentioned earlier, one should make an attempt to perform spinal anesthesia at the lower lumbar (L4-5 or L3-4) IVS to minimize the potential for spinal cord injury (9,10). Fortunately, lumbar flexion (which significantly improves access to the intervertebral–interlaminar space) does not usually change the position of the intercristal line, and even in cases in which change occurs (with full flexion), the intercristal line does not move beyond the next level (11).
Figure 6.7. Patient position for spinal or epidural blockade. The patient’s knees are drawn up toward the chest and the head flexed downward to provide the maximum anterior flexion of the vertebral column. The pillow should be placed under the head but not under the shoulders to avoid rotation of the spine. The hips and shoulders should be perpendicular to the surface of the bed, resisting the usual inclination of the patient to roll the superior shoulder forward. A line drawn between the posterior iliac crests usually crosses the spinal column at the L4-5 interspace or L4 spinous process. Similarly, for thoracic epidural injection, a line between the inferior tips of the scapulae crosses the T9 spinous process.
Figure 6.8. Distribution of the vertebral level in segments at which the conus medullaris, the intercristal (Tuffier’s) line, and the dural sac cross. The segmental levels where the spinal cord ends, Tuffier’s line crosses, and the dural sac ends follow a normal distribution. Each vertebra was divided into four segmental thirds of the vertebral body—upper (U), middle (M), and lower (L)—and the intervertebral space (IVS). The most caudal distribution of the conus should not cross with the most cephalad level of the actual intercristal line in this patient population. (Adapted from Kim JT, Bahk J, Hung JH. Influence of age and sex on the position of the conus medullaris and Tuffier’s line in adults. Anesthesiology 2003;99:1359–1363.)
III. Indications and Contraindications
A. Local anesthetics injected into the intrathecal space generally produce sensory afferent blockade and varying degrees of efferent motor block cephalad and caudad to the site of intrathecal injection. The cephalad extent of spinal anesthesia is dependent on the baricity of the local anesthetic solution relative to patient position at the time of intrathecal injection and patient position during the surgical procedure. There is a gradually decreasing concentration of local anesthetic within the CSF as a function of distance from the initial site of intrathecal injection, which causes a gradient of afferent and efferent conduction block due to the differential sensitivities of spinal nerve fibers based on their axon diameter and conduction velocity (Table 6.1). Preganglionic efferent fibers are the most sensitive to local anesthetic conduction block and therefore may be anesthetized two to six dermatome segments higher than afferent sensory block (most typically assessed as loss of pinprick sensation), which in turn extends two to three dermatomes higher than efferent motor block (12). A more clinically relevant assessment is the extent of surgical anesthesia, both in terms of the sensory extent (local anesthetic distribution within the CSF) and the density (local anesthetic concentration within the CSF). A clinically useful assessment of the dermatomal level of surgical anesthesia is the use of transcutaneous electrical stimulation (TES). Tolerance to TES (of pain or discomfort) of 10-mA, 50-Hz continuous square wave (easily produced with most peripheral nerve stimulators) for 5 seconds has been shown to be a reliable predictor of tolerance to surgical stimulation (13).
B. Knowledge of the correlation between surface anatomy and dermatomal projections of the spinal cord segments is essential to assess whether the extent (or peak block height) of sensory block is adequate for the planned surgical procedure, as well as assessment of regression of sensory block to plan discharge from the recovery room. For example, the fourth thoracic dermatome corresponds to the nipples, the sixth thoracic dermatome to the xyphoid process, the tenth thoracic dermatome to the umbilicus, and the perineum to the second through fourth sacral dermatome (Table 6.2 and Figure 6.6). It is also essential to know that the internal organs are innervated differently and anesthesia of an overlying cutaneous region does not necessarily confer surgical anesthesia to an underlying visceral organ (Table 6.3).
1. For example, a low level of spinal anesthesia (S4-L1) is useful for perianal or perineal surgery.
2. Although foot-ankle (S1-L5) and knee surgery (L3-4) occur at the lumbosacral dermatomes, the use of thigh tourniquet (and tolerance to tourniquet pain) will often require a peak sensory block height to T10-T8 (14).
3. A peak block height of T10 is ideal for transurethral prostatectomy or hysteroscopy procedures because it provides excellent surgical anesthesia while preserving sensation in the abdomen and dome of the bladder, allowing earlier warnings signs and the diagnosis of extravasation of fluid into the abdominal cavity.
4. Higher peak sensory levels of spinal anesthesia (T6-T4) are required for lower abdominal surgery such as inguinal herniorrhaphy, appendectomy, abdominal hysterectomy, or cesarean delivery. In such procedures, however, patients may perceive an unpleasant discomfort associated with traction on the peritoneum or abdominal viscera. In this case, higher levels of spinal anesthesia are required (although usually impractical) and judicious use of supplemental intravenous sedation and analgesia are indicated.
5. Higher levels of spinal anesthesia (T1) have been advocated for upper abdominal procedures (such as open cholecystectomy, bowel resection, and exploratory laparotomy. This level of spinal anesthesia provides the surgeon with a contracted bowel because of the complete sympathetic efferent block. However, this level of spinal anesthesia is associated with changes in both cardiovascular and pulmonary function. The complete efferent sympathetic efferent block will decrease resting arterial vascular tone (after-load), significantly decrease venomotor tone (preload) resulting in variable degrees of hypotension, which may be exacerbated in the patient who is hypovolemic. Additionally, this level of sympathetic efferent blockade may result in severe bradycardia (due to blockade of the cardiac accelerator fibers at T1-4). This may exacerbate any vagally mediated reflex decrease in heart rate secondary to peritoneal traction. Although high levels of spinal anesthesia do not have clinically significant effects on pulmonary function in patients without respiratory disease, motor blockade of the diaphragm and abdominal muscles does result in clinically relevant decreases in peak expiratory flow rate and peak expiratory pressure. Therefore, high levels of spinal anesthesia are not appropriate for patients who are dependent on abdominal muscles to maintain adequate ventilation.
C. There are absolute and relative contraindications to spinal anesthesia. Absolute contraindications include patient refusal, infection at proposed site of skin puncture and injection, severe untreated hypovolemia, intrinsic or iatrogenic (systemic anticoagulation and platelet inhibitors) defects in hemostasis, and increased intracranial pressure (as this may potentially increase the risk of uncal herniation when intracranial pressure is decreased as CSF is lost through the needle). Performance of spinal anesthesia in patients with preexisting neurologic deficits such as radiculopathies or peripheral neuropathies (15, 16), demyelinating diseases such as multiple sclerosis (17) is controversial. Although no clinical study has demonstrated that spinal anesthesia worsens preexisting neurologic disease, the vast majority of anesthesiologists would consider it a significant contraindication. Aortic stenosis, once considered an absolute contraindication to spinal anesthesia, does not necessarily preclude a carefully conducted spinal anesthetic (18). Despite conflicting results in the literature, except in the most extraordinary circumstances (where the benefits of regional anesthesia outweigh the potential risk of central neuraxial infection); spinal anesthesia should not be performed in patients with untreated systemic infection. In contrast, available data suggest that patients with evidence of systemic infection may safely undergo spinal anesthesia, provided appropriate antibiotic therapy is initiated before dural puncture and the patient has demonstrated response to therapy, such as decrease in fever and decrease in granulocystosis (19).
IV. Determinants of local anesthetic distribution and duration of action
A. The physiochemical properties and clinical pharmacology of local anesthetics and analgesic adjuvants (to local anesthetics) are addressed in more detail in Chapters 1 and 2. This section will discuss the determinants of local anesthetic distribution within the subarachnoid space as well as duration of action. Application of spinal anesthesia into daily clinical practice requires a block of sufficiently rapid onset to facilitate the timely start of surgery and more importantly a block of adequate duration for the planned (type and anatomic) surgical procedure, as well predictable (and prompt when needed) return of neurologic function to facilitate a timely discharge from either the recovery room or to home. Conversely, a spinal anesthetic with an unnecessary cephalad spread may increase the risk for cardiovascular complications of hypotension and bradycardia. A basic definition of a few terms is essential for understanding the determinants of the clinical efficacy of spinal anesthesia. Distribution of local anesthetics within the intrathecal space determines the spatial extent of sensory, motor, and sympathetic block. Uptake of local anesthetics into the neuronal tissues determines which neuronal functions (Table 6.1) are affected during spinal anesthesia. Uptake into neuronal tissues is a function of CSF concentration relative to the neuronal and perineuronal tissue concentration, which is largely determined by distribution, as the CSF concentration of local anesthetic will progressively decrease from the area of initial highest concentration (injection site) as a function of distance. Elimination of local anesthetics from the intrathecal space determines duration of action.
B. Determinants of intrathecal local anesthetic distribution. Many factors have been proposed to influence the distribution of local anesthetic solutions with the intrathecal space (20, 21). After injection, the local anesthetic will initially spread simply by bulk flow created by displacement of CSF. Subsequently, the most important factor in determining intrathecal local anesthetic distribution is the baricity of the local anesthetic solution relative to the influence of gravity (patient position). Other clinically relevant factors include total dose given, the IVS chosen for injection, and several patient characteristics.
1. Baricity. Baricity is defined as the ratio of the density of the local anesthetic solution relative to the density of the patient CSF at 37°C. Local anesthetic solutions that have the same density as CSF are termed isobaric. Local anesthetic solutions that have a greater density than CSF are classified as hyperbaric, whereas solutions that have a lower density than CSF are classified as hypobaric. Hyperbaric solutions will distribute (sink) to the most dependent areas of the intrathecal space, whereas hypobaric solutions will distribute toward (rise upward) the nondependent areas of the intrathecal space. The effects of gravity are determined by the choice of patient position (supine, prone, lateral, and sitting), and in the supine position, by the curvatures of the spinal column. The mean density of CSF varies significantly among specific patient population subgroups (Table 6.4). Because of the wide variability in CSF density, hyperbaric and hypobaric solutions must have densities three standard deviations above or below a patient population mean CSF density, respectively to predictably behave in a hyperbaric or hypobaric manner. Therefore, based on patient variability and the known density of local anesthetic solutions, commonly used local anesthetics (specifically, plain bupivacaine 0.5% and plain lidocaine 2.0%) classified as “isobaric” may in fact behave in a hypobaric manner depending on patient position. Table 6.4 lists the density range and classification of commonly used local anesthetics.
a. Hyperbaric spinal anesthesia. Hyperbaric solutions are commonly prepared by mixing the local anesthetic solutions with dextrose. When the patient is placed in the supine horizontal position after injection of a hyperbaric solution (in the lumbar region), the curvatures of the spinal column will influence the subsequent distribution within the intrathecal space. Hyperbaric solutions will tend to distribute by force of gravity toward the lowest points of the thoracic (T6-7) and sacral (S2) curvatures (Figure 6.4) (22). Therefore, pooling of hyperbaric local anesthetic solutions within the thoracic kyphosis has been postulated to explain the clinical observation that they tend to produce spinal anesthesia with an average peak sensory block height in the midthoracic region. Individual anatomic variations in the lowest point of the thoracic kyphosis, the maximum angle of decline of the lumbar spinal canal, and the maximum angle of incline of the upper thoracic spinal canal, may also contribute to the variability in peak sensory block height of hyperbaric solutions (23).
Figure 6.9. Sitting position for spinal anesthesia. The patient’s legs are allowed to hang over the edge of the bed, and the feet are supported on a stool to encourage flexion of the lower spine. The shoulders are hunched forward and the patient is encouraged to grasp firmly onto a pillow held over the abdomen. If sedation is given, an assistant should maintain the position and monitor the vital signs. This position is optimal for identifying the midline in obese patients or those with unusual spinal anatomy.
(1) Injection of hyperbaric local anesthetic solutions with the patient in the sitting position (for 5–10 minutes) has been advocated as a means to restrict distribution to the lumbosacral dermatomes, producing a “saddle block” (Figure 6.9). However, this practice is based on the misconception that hyperbaric solutions will then behave in an “isobaric manner” as they become diluted sufficiently in the CSF and are no longer influenced by gravity once the patient is placed in the supine (or supine lithotomy) position. Clinical studies, however, have demonstrated that a hyperbaric spinal anesthetic block initially restricted to the lumbosacral dermatomes will eventually distribute to the peak thoracic sensory block height equivalent to that which would have been obtained had the patient been immediately placed in the horizontal supine position (24).
(2) Similar to the concept of obtaining a saddle block (by restricting the distribution of hyperbaric local anesthetic solution based on gravity), injection of hyperbaric solutions with a patient in the lateral position (with the operative side dependent) and maintaining the operative side in the dependent position for 10 to 15 minutes has also been advocated as a means to achieve “unilateral spinal anesthesia”. One of the proposed advantages of unilateral spinal anesthesia is that limiting the sympathetic blockade to one side may decrease the incidence of hypotension (25). The major disadvantage of unilateral spinal anesthesia is that it requires at least 15 minutes in the lateral position after injection to predictably obtain a unilateral blockade.
b. Hypobaric spinal anesthesia. Hypobaric local anesthetic solutions are typically prepared by diluting commercially available plain local anesthetic solutions with sterile distilled water. For example, lidocaine 2% diluted with sterile water to lidocaine 0.5% (26) or bupivacaine 0.5% diluted with sterile water to 0.35% (27) will reliably result in “clinically hypobaric” spinal anesthesia. Although less commonly used, hypobaric spinal anesthesia is ideally suited for perineal and perirectal surgical procedures performed with the patient in the prone jackknife position (Figure 6.10). The advantages of this technique are twofold.
(1) First, the local anesthetic is injected with the patient in the operative position, thereby minimizing the need to change patient position.
Figure 6.10. Jackknife position. Hypobaric spinal anesthesia can be administered with the patient positioned on a flexed operating table, such as for rectal procedures. The flexion point of the table should be directly under the hip joint, and the use of a pillow under the hips will help accentuate the flexion needed to identify the lumbar spinous processes. Aspiration of the spinal needle is often necessary to confirm dural puncture, because the lower cerebrospinal fluid (CSF) pressure in this position will not necessarily generate a spontaneous flow of fluid.
(2) Second, the local anesthetic distribution will be restricted to lumbosacral dermatomes as long as the patient is maintained in the operative position.
Care must be taken when recovering the patient who still has a functional block, as placing the patient in the head-up position during the recovery period may cause the block to rise to the thoracic dermatomes (26). Hypobaric spinal anesthesia may also provide significant advantages for major hip surgery performed in the lateral position (with operative side uppermost). Compared with isobaric bupivacaine spinal anesthesia, hypobaric bupivacaine spinal anesthesia demonstrated a significantly delayed onset of sensory regression on the operative side and more importantly, time needed until need for analgesia after surgery (27).
c. Isobaric spinal anesthesia. An isobaric local anesthetic solution is most easily prepared by mixing equal volumes of tetracaine 1% solution with either CSF or sterile saline. As stated before, plain bupivacaine 0.5%, commonly referred to as isobaric, is clearly a hypobaric mixture (Table 6.3). Therefore, depending on the patient position at the time of injection and during surgery, this solution will behave “unpredictably” if one expects it to behave in an isobaric manner. The major advantage of truly isobaric solutions is that patient position during and after injection should have no effect on intrathecal distribution. Isobaric solutions tend not to distribute far from the site of initial injection and are particularly useful when it is undesirable to obtain sensory block in the higher thoracic dermatomes.
2. Dose, volume, and concentration. Clinical studies attempting to separate the individual effects of dose, volume, or concentration on local anesthetic distribution are difficult to interpret due to the fact that manipulating one of these factors affects either one or both of the other factors. In two well-done studies that compared different doses of plain local anesthetic solutions (28,29), as well as the same dose administered in lower concentrations (as much as 20-fold lower) by increasing the volume of the injected local anesthetic solution, the predominant factor in increasing the extent of sensory block (peak block height) was the total mass of drug given, regardless of volume or concentration. In contrast, drug dose is relatively less important in determining the distribution of hyperbaric local anesthetic solutions in patients placed in the supine horizontal position after initial injection. Several studies have demonstrated when the total dose of hyperbaric bupivacaine is greater than 7.5 to 10.0 mg; there is no difference in peak block height in patients placed in the supine horizontal position (30, 31). Conversely, in a dose–response study of hyperbaric bupivacaine 0.75%, 3.75 mg, 7.5 mg, and 11.25 mg, the peak sensory block heights obtained were T9, T7, and T4(32).
3. IVS injection site. The location of the lumbar IVS injection site has been proposed to have a clinically significant effect on peak sensory cephalad distribution of plain local anesthetic solution. Conflicting studies can be explained by the use of plain bupivacaine 0.5% in these studies. In one study, the mean peak block height was decreased from T6 to T10 when plain bupivacaine 0.5% was injected initially at L3-4, followed by a repeat injection in the same patients at the L4-5 IVS (33). In this study, plain bupivacaine 0.5% solutions were injected at ambient room temperature with the patient in the lateral position and then immediately placed in the supine horizontal position. In a conflicting study (34), when plain bupivacaine 0.5% solutions adjusted to 37°C are injected into patients in the sitting position and maintained in the sitting position for 2 minutes before placing them supine, there was no difference in peak sensory block height when injections at the L2-3 versus L3-4 IVSs were compared. These seemingly conflicting data can be explained as follows. First, plain bupivacaine 0.5% is clearly hypobaric and not isobaric as these previous studies assumed. Second, there were differences in the baricity of the plain bupivacaine solutions, as the density of local anesthetic solutions is inversely proportional to temperature. Third, there were differences in patient position during initial injection. Therefore, it appears that when hypobaric solutions are injected with the patient in the sitting position, the effect of the IVS is outweighed by the effect of gravity (as the hypobaric solutions will float cephalad within the intrathecal space) relative to local anesthetic baricity.
4. Needle aperture direction. The use of a pencil point needle (such as a Whitacre or Sprotte, see Chapter 5) results in directional flow out of the needle aperture, diverting the direction of local anesthetic direction from the longitudinal aspect of the needle (35). In a study of plain lidocaine 2%, patients were randomly assigned to local anesthetic injection (in lateral position) with the needle aperture either oriented in a cephalad direction or a caudad direction (36). The group with the local anesthetic injection directed in the cephalad direction had a mean higher peak sensory block height (T3 versus T7), as well as a shorter duration of lumbar (L1-5) sensory anesthesia (149 versus 178 minutes), and shorter time to spontaneous urination and discharge. Therefore, it appears that with plain-isobaric solutions, needle aperture direction does influence local anesthetic distribution. Additionally, by presumably providing sacral sparing in this study, there was a faster return of sacral autonomic function, thereby, facilitating a more timely discharge.
5. Patient factors. Although there is significant variation in the extent of local anesthetic distribution among patients using a standard technique, spinal anesthesia is very reproducible in the individual patient (37). However, individual patient factors such as age, height, body mass index, and gender have little if any clinically relevant significance in accurately predicting local anesthetic distribution differences from patient to patient.
C. Determinants of duration of action of spinal anesthesia. Clinically, spinal anesthetic blockade recedes in a cephalad to caudad manner from peak block height to the sacral dermatomes. Depending on the criteria chosen in any given study, duration of action can be defined as time to onset of twodermatome regression from peak sensory block height, or as time to complete regression to sacral dermatomes. More clinically relevant, the duration of surgical anesthesia is dependent on the interaction of the spatial extent of the block, time-course regression, and anatomic location of the surgical procedure. Additionally, time course to complete regression of sensory and motor block are important considerations for ambulatory spinal anesthesia. Resolution of blockade after spinal anesthesia occurs when the neural tissue concentration of local anesthetic falls below the minimum blocking concentration for given neural function. Elimination does not involve metabolism of local anesthetics with the intrathecal space, but occurs completely through vascular absorption within both the intrathecal and epidural space. Therefore, the duration of spinal anesthesia is primarily determined by the physiochemical properties of the specific local anesthetic agents that determines it availability for vascular absorption, the total mass of local anesthetic administered, and the degree of vascular absorption.
1. Local anesthetic choice. One of the primary determinants of duration of action is the choice of local anesthetics. The physiochemical properties of lipid solubility and to a lesser extent, protein binding largely influences the time course of vascular absorption (see Table 1.1 in Chapter 1).
a. Procaine is the shortest acting local anesthetic for spinal anesthesia. Its short duration of action can be largely attributed to its very low lipid solubility and protein binding, relative to the longer-acting agents.
b. 2-Chloroprocaine is also a short-acting local anesthetic agent that has been shown in volunteer and clinical studies to have a comparable anesthetic profile to equivalent doses of lidocaine, with the notable exceptions of lack of transient neurologic symptoms (TNS) and a 20% faster time to complete recovery of sensorimotor function (14,38).
c. Lidocaine is considered a short-to-intermediate duration local anesthetic agent, and has historically been the most extensively used local anesthetic for spinal anesthesia. Plain lidocaine of dose 50 mg will produce a peak block of T6 with onset of 2-dermatome regression of 50 to 60 minutes, 100 minutes of lumbar anesthesia, and complete regression in 120 to 140 minutes. The use of lidocaine has fallen dramatically due to concerns regarding TNS (see discussion on complications). Depending on the type of surgery, the incidence of TNS ranges from 15% to 33%.
d. Mepivacaine is also a short-to-intermediate duration local anesthetic agent and provides a similar anesthetic profile compared to equivalent doses of lidocaine, with a lower incidence of TNS (3%–6%) compared to lidocaine.
e. Bupivacaine is the prototypical and most widely used l ong-acting aminoamide local anesthetic agent. The extent and duration are dose related (32). Within a clinically relevant dose range of 3.75 to 11.25 mg of hyperbaric bupivacaine 0.75%, it appears that for every additional milligram, there is an increase in the average duration of surgical anesthesia by 10 minutes and an increase until complete recovery of 21 minutes. There is, however, a wide degree of variability, largely due to its high degree of lipid solubility. Lower doses (5–7.5 mg) have been used for ambulatory anesthesia as an alternative to lidocaine, but are hampered by a higher degree of block failure and wide interpatient variability in complete resolution.
f. Ropivacaine and levobupivacaine are long-acting aminoamides developed as alternatives to bupivacaine as “less cardiotoxic” alternatives for epidural and peripheral regional anesthesia. Levobupivacine is less cardiotoxic by virtue of its formulation as the pure L-stereoisomer of bupivacaine, but is no longer commercially available in the United States. Ropivacaine is less cardiotoxic due to combination of its formulation as a pure L-stereoisomer and chemical structure (lower lipid solubility) conferring a lower potency. Because cardiotoxicity is not a clinically relevant concern when using bupivacaine for spinal anesthesia (given the doses used for spinal anesthesia and the doses required to produce significant cardiotoxicity), neither local anesthetic has gained widespread use.
g. Tetracaine is the prototypical long-acting aminoester local anesthetic agent. Its increased lipid solubility (compared to bupivacaine) confers greater potency and the dose can be decreased by 20% to 30% for equivalent blockade.
h. Although local anesthetic agents may be classified as short, intermediate, and long-acting, it is evident from Table 6.5 that there is wide interpatient variability. In a study (39) comparing 12 volunteers who underwent spinal anesthesia on three separate occasions with three different hyperbaric local anesthetic agents (lidocaine 100 mg, bupivacaine 15 mg, and tetracaine 15 mg) in random order and in double-blind manner, the average time until complete sensory resolution was not only different between the three agents, but was also highly variable within each local anesthetic agent group: lidocaine (234 minutes, range 137–360 minutes), bupivacaine (438 minutes, range 180–570 minutes), and tetracaine (546 minutes, range 120–720 minutes).
2. Local anesthetic dose. For any given local anesthetic agent, increasing the dose increases the duration of action. See Table 6.5 for details.
3. Block distribution. For a given dose of local anesthetic, spinal blockade with a higher peak sensory block will completely regress faster compared to a lower peak cephalad distribution (40). The most likely pharmacokinetic explanation for this phenomenon is based on the wider distribution within the CSF with a higher peak sensory block height. The wider CSF distribution presumably results in a lower local anesthetic CSF concentration throughout the intrathecal space, as well as a larger surface area leading to more rapid vascular absorption (41). Consequently, it requires less time for the local anesthetic concentration within the neural tissue to fall below the minimum blocking concentration. As noted earlier, the use of hypobaric spinal anesthesia for unilateral hip surgery performed with operative side uppermost not only prolongs the duration of anesthesia on the operative side (as compared to the dependent nonoperative side), but also prolongs the duration of postoperative analgesia (28).
4. Anesthetic adjuncts. The two most commonly used class of anesthetic adjuncts added to local anesthetic solutions to prolong (as well as intensify the depth of block) of spinal anesthesia are α-adrenergic agonists and opioids.
a. α-Adrenergic agonists. The primary mechanism by which intrathecal administration of epinephrine prolongs the duration of action is believed to be due to α-adrenergic–mediated vasoconstriction, thereby leading to decreased vascular absorption thus allowing for increased neural tissue uptake of local anesthetics. Clinically, the effectiveness of intrathecal epinephrine is dependent on the local anesthetic agent to which it is added. Although epinephrine 0.2 mg does not prolong the duration of thoracic anesthesia when added to lidocaine, it clearly prolongs the duration of lumbosacral anesthesia by 25% to 30%, which would be clinically relevant for lower extremity and perineal surgery (42). Similarly, epinephrine 0.2 mg added to plain bupivacaine 15 mg did not prolong thoracic anesthesia, but increased the duration of lumbar anesthesia by 20% (43). In contrast, epinephrine 0.2 to 0.3 mg significantly prolongs the duration of tetracaine spinal anesthesia by 30% to 50% at all dermatomal levels, with slightly greater effect in the lumbosacral dermatomes (44,45). Despite its usefulness in reliably prolonging the duration of spinal anesthesia, epinephrine significantly prolongs the return of sacral autonomic function (the ability to spontaneously void) and increases the risk of urinary retention (and possibly bladder overdistention) (46). Therefore, the use of epinephrine is not recommended for outpatient spinal anesthesia.
b. Opioids. Opioids interact synergistically when combined with local anesthetic solutions by blocking noxious afferent stimuli at a different site of action than local anesthetics. Specifically, opioids interact with and bind to opioid receptors located within the gray matter of the substanstia gelatinosa in the dorsal horn of the spinal cord. Spinally mediated analgesia is mediated by several mechanisms: (i) increased K+ conductance, which hyperpolarizes ascending postsynaptic second-order projecting neurons, (ii) release of spinal adenosine, and (iii) inhibition of the release of excitatory neurotransmitters including glutamate and substance P from primary afferent neurons (47,48).
(1) Fentanyl is by far the most commonly used intrathecal opioid. The lipophilic profile of fentanyl allows it to have a rapid onset of action (5–10 minutes) and an intermediate duration of action (60–120 minutes), which makes it a suitable agent as an analgesic adjunct to local anesthetic solutions to augment the efficacy of spinal anesthesia. Clinical studies have demonstrated that 20 to 25 μg of fentanyl added to lidocaine (49) or bupivacaine (50) for spinal anesthesia prolongs the duration of anesthesia without prolonging the time to complete recovery of sensorimotor function and bladder function.
(2) Morphine is the most commonly used hydrophilic opioid. Its physiochemical characteristics results in a slow onset of action (30–60 minutes), but also a prolonged duration of action, which makes it well suited for extended postoperative analgesia. Doses of preservative-free morphine in the range of 100 to 200 μg provide extended spinally mediated analgesia (up to 24 hours) for a variety of common lower abdominal and lower extremity procedures such as cesarean delivery, abdominal hysterectomy, radical prostatectomy, and total hip arthroplasty (51). At these lower doses, the risk of respiratory depression is very unlikely. In contrast, the minimum effective analgesic dose required after total knee arthroplasty is typically 300 to 500 μg (52,53). At these doses, the occurrence of side effects such as nausea, vomiting, urinary retention, and pruritus increase significantly compared to lower doses (53,54). The risk of respiratory depression with intrathecal morphine is dose related, with a few instances of clinically significant respiratory depression with doses in the 300 to 500 μg range (54,55).
V. Technique
The successful technique of spinal anesthesia begins with proper preparation well before insertion of the spinal needle. Because the induction of spinal anesthesia results in numerous physiological changes as well the occurrence of rare, but potentially serious complication, immediate detection, and corrective action must be taken to minimize significant morbidity and even mortality. The location where spinal anesthesia is induced must be equipped with an oxygen source, a means to administer positive pressure ventilation, airway management equipment, as well as immediate access to emergency drugs for resuscitation and intubation. Patient preparation includes proper monitoring, including continuous monitoring of heart rate and oxygen saturation and intermittent noninvasive blood pressure monitoring. Finally, intravenous sedation may be administered to facilitate patient cooperation to a degree that the patient is comfortable but cooperative and communicative.
A. Patient position. Proper patient positioning is critical to making spinal anesthesia efficient and successful. The choice of which position to perform spinal anesthesia is influenced by a combination of the preference of the anesthesiologist, patient characteristics (obesity, hemodynamic status, and the presence of painful conditions such as hip fracture), and the baricity of local anesthetic solution in conjunction with the site and position of surgery. The three positions in which spinal anesthesia may be performed are lateral decubitis, sitting, and prone jackknife.
1. Lateral decubitus position (Figure 6.7). The lateral decubitis position is especially useful for lower extremity surgery, depending on the baricity of the local anesthetics solution. The use of hyperbaric solutions with the operative side dependent allows initial preferential distribution to the surgical site depending on the duration of time spent in the lateral position after intrathecal injection. Hypobaric solutions are ideally suited for hip surgery performed in the lateral position as the position is then maintained for the duration of the surgical procedure. Ideally, the patient’s back is positioned parallel to the edge of the operating table (or hospital gurney) to allow the anesthesiologist easy access to the lower back. The hips and knees are flexed so as to draw the patient’s knees toward the abdomen and lower chest. The neck may also be flexed forward. The hips and shoulders should be aligned so that they are perpendicular to the edge of the bed, thereby preventing the spine from rotating. The head and lower legs may need to be supported with pillows or blankets, especially if the patient’s hips are broad. It is often very helpful to have a trained assistant help attain and maintain this ideal position. The patient should be encouraged to actively curve the lower back toward the anesthesiologist. All of these maneuvers will contribute to widening the lumbar intervertebral and interlaminar spaces.
2. Sitting position (Figure 6.9). Many anesthesiologists prefer the sitting position as this facilitates identification of the midline, especially in obese patients. The patient should be seated with the legs hanging off the operating table and the feet supported by a footrest to facilitate forward flexion at the hips and maintain position stability. A pillow placed on the patient’s thighs will also help encourage the patient to maintain forward flexion of the lumbar spine. Once again, an assistant can prove invaluable to encourage and help the patient maintain this position. Depending on the surgical site, the patient may need to be positioned supine immediately after intrathecal injection. If the patient is left in this position for several minutes after intrathecal injection of a hyperbaric local anesthetic solution, preferential lumbosacral anesthesia is produced, creating a classically referred to saddle block. Careful attention must be paid to the patient’s blood pressure if left in the sitting position for any length of time after intrathecal injection, as this will only enhance the degree of expected venous pooling in the lower extremities (and significant decreases in venous return) due to the sympathetic effects of the spinal anesthetic.
3. Prone jackknife position (Figure 6.10). For surgical procedures that require the patient to be in the prone jackknife position ( such as rectal or perineal procedures) the efficient induction of spinal anesthesia may be accomplished by injection of a hypobaric local anesthetic solution with the patient already placed in this position. The patient is positioned prone on the operating table with the hips placed directly over the break in the operating table to facilitate flexion at the hips (Figure 6.10). Additionally, a pillow placed under the lower abdomen helps flexion of the lumbar spine.
B. Anatomic approach to the subarachnoid space. Spinal anesthesia should be performed in the mid-to-lower lumbar IVSs, ideally at L4-5 IVS or L3-4 IVS. Spinal anesthesia attempted at or above the L1-2 IVS should be avoided to minimize the risk of needle trauma to the conus medullaris, as it may be located as low as the upper part of the L3 vertebral body in a small percentage of patients (Figure 6.8). After the patient is positioned and the desired IVS is located, there are two key steps to minimize the risk of infection: First, the anesthesiologist should clean his or her hands by either careful hand washing or preferably, with an alcohol-based hand rub. Next, wide and uniform application of skin disinfectant with either povidone-iodine (PI) solution or chlorhexidine-alcohol solution to the skin around the intended puncture site should be performed and then allowed to dry. All antiseptic solutions are neurotoxic, and care must be taken to prevent contamination of spinal needles or local anesthetic solutions with the disinfectant.
1. Midline approach. After the appropriate monitors and supplemental oxygen are applied, intravenous sedation is titrated to patient comfort, the appropriate patient position is obtained, the IVS space is chosen and marked, and sterile preparation and draping are completed, the technique of subarachnoid puncture is begun. In the midline approach, the spinal needle insertion is located in between the adjacent spinous processes (Figures 6.3 and 6.11).
a. After the patient is warned, a 25- to 27-gauge needle is used to slowly and gently raise a skin wheal of local anesthetic over the intended spinal needle insertion site. Next, a 22- to 25-gauge needle is then inserted and advanced with a 10 to 15 degree of cephalad angulation (while injecting local anesthetic) through the subcutaneous tissue, supraspinous ligament, and then, the interspinous ligament (Figure 6.11).
b. After local anesthetic infiltration, the patient position is rechecked, as the patient may pull away if there is discomfort associated with the initial local anesthetic infiltration and reduce the flexion of the lower back.
Figure 6.11. The needle insertion point and angulation of needle advancement are illustrated for paraspinous (a) and midline (b) approaches. (Adapted from Cousins MJ, Bridenbaugh LD, eds. Neural blockade in clinical anesthesia and management of pain, 3rd ed. Philadelphia: Lippincott Williams & Wilkins, 1998:231.)
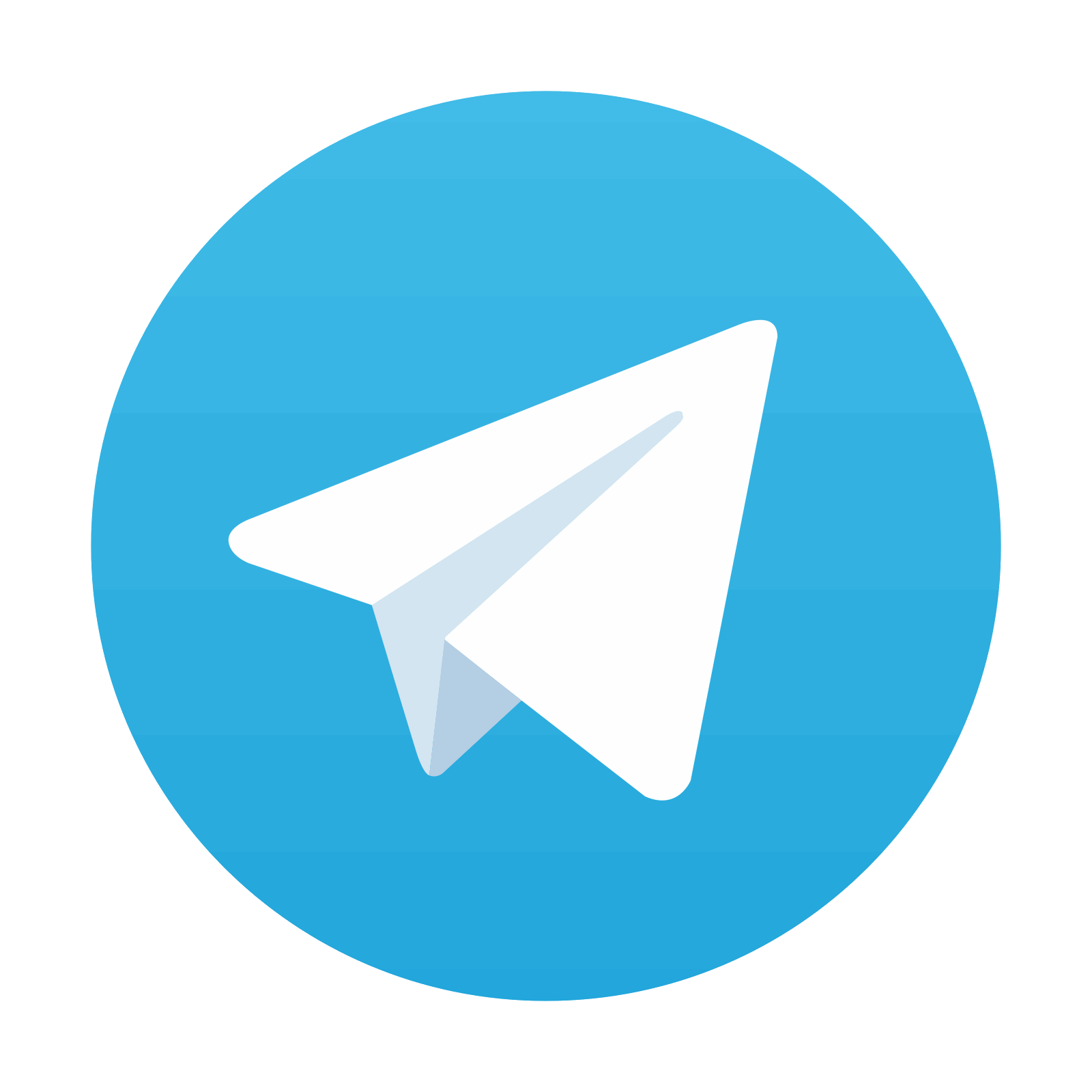
Stay updated, free articles. Join our Telegram channel

Full access? Get Clinical Tree
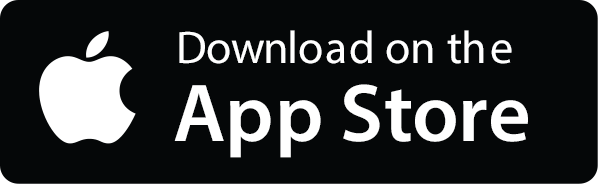
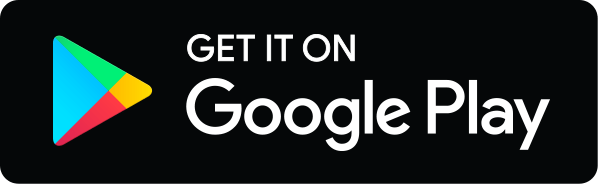