Chapter 29 Shock States
Definition and Physiology
Delivery of oxygen is a direct function of cardiac output (CO) and arterial oxygen content (CaO2):
Calculation of oxygen delivery provides a measure of global oxygen delivery and may not reflect regional hypoperfusion and localized ischemia. Inadequate oxygen delivery can result from either limitation or maldistribution of blood flow. Reduced oxygen content (anemia, poor arterial oxygen saturation) necessitates higher cardiac output to maintain oxygen delivery. In certain situations (fever, sepsis, trauma), metabolic demands may exceed normal oxygen delivery. Impairment of the extraction or utilization of oxygen by cells and mitochondria creates a functional arteriovenous shunt and may be the harbinger of multiorgan dysfunction syndrome.1–3
Because of its progressive nature, shock can be divided into phases: compensated, uncompensated, and irreversible. In compensated shock, vital organ function is maintained primarily by intrinsic regulatory mechanisms. Previously healthy children can compensate and maintain normal blood pressure during hypoperfusion states. Therefore identification of the early compensated stage of shock is crucial. Diagnosing a patient as having early compensated shock, rather than mere dehydration, may be the difference between a patient who is appropriately resuscitated and one for whom resuscitative efforts are delayed. As shock progresses, the cardiovascular system’s ability to compensate is exceeded, and microvascular perfusion becomes marginal. Cellular function deteriorates, affecting all organ systems. Terminal or irreversible shock implies damage to key organs of such magnitude that death occurs even if therapy restores cardiovascular function to adequate levels.
Recognition and Assessment of the Shock State
The early diagnosis of shock requires a high index of suspicion and knowledge of conditions that predispose children to shock. Interviews of the parents, physicians, nurses, and emergency medical services personnel caring for the child provide valuable information. A rapid and focused physical examination of a patient in shock is essential (Box 29-1).
The contribution of laboratory tests to the initial evaluation of patients in shock is limited. Blood gases and serum lactate levels may quantify the degree of acidosis and are widely used as markers for the effectiveness of treatment. However, an increased understanding of microcirculatory aberrations and cellular hypoxia has raised awareness of the limitations of tests on pooled venous samples. This has stimulated a search for a minimally invasive means of sampling regional circulations.4 Gastric tonometry,5,6 near-infrared spectroscopy,7,8 rectal tonometry,9 and sublingual capnography10,11 are methods currently being investigated to evaluate regional circulation. Their clinical utility is unproven at this time.
Treatment of Shock
General Principles
Shock is a clinical syndrome of inadequate tissue oxygenation. In addition to treatment of the primary underlying process, therapeutic efforts involve optimizing and balancing oxygen delivery and oxygen consumption. Efforts to reduce oxygen requirements at a time when oxygen delivery is compromised are important. Intubation, mechanical ventilation, sedation, paralysis, and control of fever are ways to reduce oxygen consumption. Even routine nursing procedures can increase oxygen consumption by up to 20% to 30% in healthy adults.12
Intubation and Mechanical Ventilation
Viires et al.13 studied spontaneously breathing dogs during cardiogenic shock. During a low cardiac output state, blood flow to the diaphragm was substantially increased, while blood flow to the liver, brain, and quadriceps was significantly decreased. Intubation, mechanical ventilation, and paralysis resulted in redistribution of blood flow from the diaphragm to the liver, brain, and quadriceps. A similar study of endotoxic shock in dogs demonstrated that respiratory muscle blood flow rose significantly in spontaneously breathing dogs.14 During shock states, there is often increased work of breathing and respiratory distress related to capillary leak and acidosis.
Intubation and mechanical ventilation can allow redistribution of cardiac output from the muscles of respiration to vital organs during shock (when cardiac output and oxygen delivery are compromised). Positive pressure ventilation also has the effect of reducing afterload to the left ventricle (potentially improving stroke volume) (see Chapter 26).
Fluid Resuscitation
Regardless of the underlying insult, all patients in shock have an absolute or relative hypovolemia. A primary goal of initial therapy must be restoration of effective circulating volume. Early fluid resuscitation is the cornerstone of immediate therapy.15,16 In a study of pediatric septic shock patients, Carcillo et al.15 correlated the volume of fluid given in the first hour of presentation and reversal of hypovolemia to outcome. Patients who received the largest volume of fluid in the first hour of resuscitation had the lowest mortality. Persistent hypovolemia was associated with increased mortality. Fluid resuscitation must be guided by repeated evaluation of the patient’s hemodynamic status.
Vasoactive Infusions
Vasoactive infusions are commonly used when patients have been adequately fluid-resuscitated but hemodynamics remain deranged. Infusions of catecholamines (dopamine, dobutamine, epinephrine, norepinephrine), phosphodiesterase inhibitors (inamrinone, milrinone), and vasopressin are most commonly used. The choice of vasoactive infusion is dependent on the physiologic derangement requiring treatment (Table 29-1). Catecholamines work through stimulation of α1, α2, β1, β2, and dopaminergic receptors to increase intracellular cyclic guanosine monophosphate (cGMP) and cause the appropriate response (Table 29-2). Phosphodiesterase inhibitors increase cGMP by preventing its degradation within the cell (see Chapter 25). Vasopressin causes vasoconstriction by direct stimulation of vascular smooth muscle cell V1 receptors.17–22 Vasopressin also potentiates systemic adrenergic effects.12,24–26 Vasopressin20–22 and terlipressin27–31 (a synthetic analog of vasopressin with a similar pharmacodynamic profile, but with a significantly longer half-life) have also shown some utility in the treatment of catecholamine-resistant shock.
Other Therapies
The finding of hypocalcemia in infants who present in shock should raise the suspicion of left ventricular dysfunction. Hypocalcemia causes left ventricular dysfunction in neonates and is reversible with calcium therapy.32 Of note, 30% of neonates with DiGeorge syndrome are hypocalcemic.
Neonates, who have low glycogen stores and increased metabolic requirements during shock, may quickly develop hypoglycemia.32 Shock in neonates is frequently complicated by pulmonary hypertension.
Multisystem Effects of Shock
Management of the multisystem deterioration that occurs in shock states is as important as treating the underlying condition. Respiratory, gastrointestinal, central nervous system, renal, and hematologic abnormalities in shock must be identified and treated. Multiple organ dysfunction syndrome (MODS) is the derangement of two or more organs after an insult. The severity of MODS has been associated with increased mortality in PICU patients.33–35
Respiratory
Respiratory failure frequently accompanies shock states. It may result from failure of the ventilator pump (i.e., respiratory muscle fatigue) and/or deterioration of lung function (i.e., acute respiratory distress syndrome). For these reasons and for maximizing oxygen delivery, increased inspired oxygen is essential in all children with shock. Early tracheal intubation protects the airway, provides relief from respiratory muscle fatigue, facilitates provision of positive airway pressure, redistributes blood flow from the muscles of respiration to core organs, afterload-reduces the left ventricle, and reduces oxygen demands of respiratory muscles. Patients should be ventilated with a lung protective strategy (see Chapter 51).
Renal
Renal failure may develop in association with any of the shock syndromes. Shock-related renal failure is a continuum of acute prerenal azotemia through classic acute tubular necrosis to cortical necrosis. Renal support is essential to prevent prolonged renal shutdown in shock states. Volume augmentation to correct absolute or relative hypovolemia is essential. Although low-dose dopamine (3 to 5 μg/kg/min) improves renal blood flow,36,37 it also impairs renal oxygen kinetics, inhibits protective feedback loops with the kidney, and may worsen tubular injury.38,39 It has failed to show benefit in preventing or altering the course of acute renal failure in adults.38,39 Dopamine may also inhibit secretion of prolactin, growth hormone, and thyrotropin in critically ill children.40
Acute anuric renal failure may require treatment with peritoneal dialysis, ultrafiltration, continuous hemofiltration or hemodiafiltration, or hemodialysis (see Chapter 72). High-output renal failure may occur in shock states without previous oliguria. The polyuria associated with this condition may falsely suggest adequate renal perfusion and adequate vascular volume at a time when the patient’s intravascular volume is, in fact, depleted. Restoration of renal perfusion pressure remains the standard of care.
Populations for whom early renal replacement therapies result in decreased mortality have not been consistently identified.41,42 There is evidence that fluid overload is related to mortality in critically ill children with renal dysfunction.43 If renal dysfunction exists, all medications and therapies should be adjusted for creatinine clearance.
Coagulation
Coagulopathy is ubiquitous in all patients with severe sepsis.44 Recombinant activated protein C has been shown to decrease mortality in adults with severe sepsis (see section on septic shock).
Use of plasmapheresis, plasma exchange, and plasma filtration as therapy for treating sepsis-induced multiple organ system failure and improving outcome remains experimental.42,45–49
Gastrointestinal
Gastrointestinal disturbances after hypoperfusion and stress include bleeding, ileus, and bacterial translocation. Ileus may result from electrolyte abnormalities, administration of narcotic medications, or from shock itself. Abdominal distension from ileus or ascites may cause respiratory compromise, especially in infants. Use of prophylactic medications (H2 blockers, protein pump inhibitors, sucralfate) to prevent gastrointestinal hemorrhage is unproven.50 Use of histamine antagonists has been associated with an increased incidence of nosocomial pneumonias.50,51
Acute nonocclusive mesenteric ischemia is a devastating condition characterized by intense, prolonged splanchnic vasoconstriction, intestinal mucosal hypoxia, and acidosis. Mesenteric ischemia eventually leads to transmural necrosis of the bowel, bacterial translocation, sepsis, and multisystem organ dysfunction.52–54 Morbidity and mortality for this condition are high because the signs/symptoms are nonspecific, delaying diagnosis. Prevention of gut ischemia through adequate oxygen delivery may prevent bacterial translocation. Some clinicians advocate the use of selective gut decontamination and early enteral nutrition.55–56 Most children with shock will tolerate postpyloric enteral feeding, although GI feeding complications are more common than in critically ill patients without shock.57,58
Functional Classification and Common Underlying Etiologies
Shock states can be classified into six functional categories (Box 29-2). Such tidy classifications imply a degree of precision that will be misleading when approaching an individual patient. Vicious cycles play a prominent role in most shock syndromes. Any given patient, over time, may display features of any functional category or features of multiple categories. Hemodynamic profiles of these categories are summarized in Table 29-1.
Hypovolemic Shock
Etiology and Pathophysiology
Hypovolemia is the most common cause of shock in infants and children. Hypovolemic shock is best defined as a decrease in the intravascular blood volume to such an extent that effective tissue perfusion cannot be maintained. Etiologies include hemorrhage (see Chapter 112), fluid and electrolyte loss (see Chapter 67), endocrine disease (see Chapter 77), and plasma loss (Box 29-3).
Box 29–3 Etiologies of Hypovolemic Shock
Courtesy Mark S. McConnell, MD, and Ronald M. Perkin, MD.
Therapy
Initial treatment of the child in hypovolemic shock is similar regardless of etiology. Therapy begins with the establishment or assurance of adequate oxygenation and ventilation. Oxygen should always be the first drug administered. Once the airway is assured or established (may require intubation) and ventilation is adequate, measures to restore an effective circulating blood volume should begin immediately. Placement of an adequate intravenous or intraosseous catheter and rapid volume replacement are the most important therapeutic maneuvers to reestablish the circulation (see Chapter 15). Central venous catheterization is infrequently necessary during initial resuscitation.
The choice of fluid depends on the nature of the loss. Early correction of hypovolemia is the major factor preventing the later complications of shock. Isotonic crystalloid solutions, which are readily available, safe, and the least expensive, should be used in initial volume resuscitation. The first fluid bolus (20 mL/kg) should be administered as rapidly as possible. Heart rate, pulse pressure, blood pressure, peripheral perfusion, quality of mentation, and volume of urine output should be monitored. Improvement in these measurements should be expected if the blood volume loss is approximately 20%. Under these conditions, a rapid response to resuscitation can be anticipated. Maintenance fluid administration then can be initiated and vital signs monitored. The appropriate maintenance fluid depends on the measurements of serum electrolytes, total protein, and hematocrit.
In the case of hemorrhagic hypovolemia, blood must be obtained and transfused if hypotension persists despite early crystalloid infusions. The patient with severe anemia in shock may need emergency transfusion of uncrossmatched blood as part of the initial resuscitation. The hematocrit may be a poor indicator of the severity of hemorrhage because it may not immediately decline in the setting of acute hemorrhagic shock. The possibility of occult intrathoracic or intraabdominal bleeding must be considered. Concomitant with fluid resuscitation of hemorrhagic shock, early surgical intervention may be indicated to control the source of bleeding. In the setting of hemorrhagic shock caused by penetrating trauma in adults, surgical control of the bleeding site may be more important than initial fluid resuscitation in improving patient outcome.14
Cardiogenic Shock or Congestive Heart Failure
Etiology and Pathophysiology
Cardiac shock is the pathophysiologic state in which an abnormality of cardiac function is responsible for the failure of the cardiovascular system to meet the metabolic needs of tissues. The common denominator is depressed cardiac output, which in most instances is the result of decreased myocardial contractility. Cardiogenic shock or congestive heart failure (CHF) during infancy and childhood is a diagnostic and therapeutic challenge because of its myriad etiologies (Box 29-4).
Box 29–4 Etiologies of Cardiogenic Shock
Courtesy Mark S. McDonnell, MD, and Ronald M. Perkin, MD.
Cardiac function can be depressed in patients with shock of noncardiac origin. Myocardial dysfunction is frequently a late manifestation of shock of any etiology. Although the cause of myocardial dysfunction in such patients is not completely understood, the following mechanisms have been proposed: (1) specific toxic substances released during the course of shock that have a direct cardiac depressant effect, (2) myocardial edema, (3) adrenergic receptor dysfunction, (4) impaired sarcolemmic calcium flux, and (5) reduced coronary blood flow resulting in impaired myocardial systolic and diastolic function.20
Another form of cardiogenic shock is caused by diastolic dysfunction. Impaired myocardial relaxation changes the pressure-to-volume ratio during diastole and increases ventricular pressure at any volume. This lack of myocardial relaxation is hemodynamically unfavorable because increased left ventricular diastolic pressure is transmitted to the lung and results in pulmonary edema and dyspnea. Elevated left ventricular diastolic pressure also decreases myocardial perfusion pressure and can lead to subendocardial ischemia. Such patients present with “heart failure” but may have normal left ventricular systolic function. Diastolic properties of the ventricle appear to be the first to become abnormal in patients with ischemic heart disease or disorders associated with ventricular hypertrophy.61,62 Therefore when approaching a patient with cardiogenic shock, it is important to characterize both systolic and diastolic function. Therapy designed to improve systolic function may impair myocardial diastolic function (see Chapter 19).62
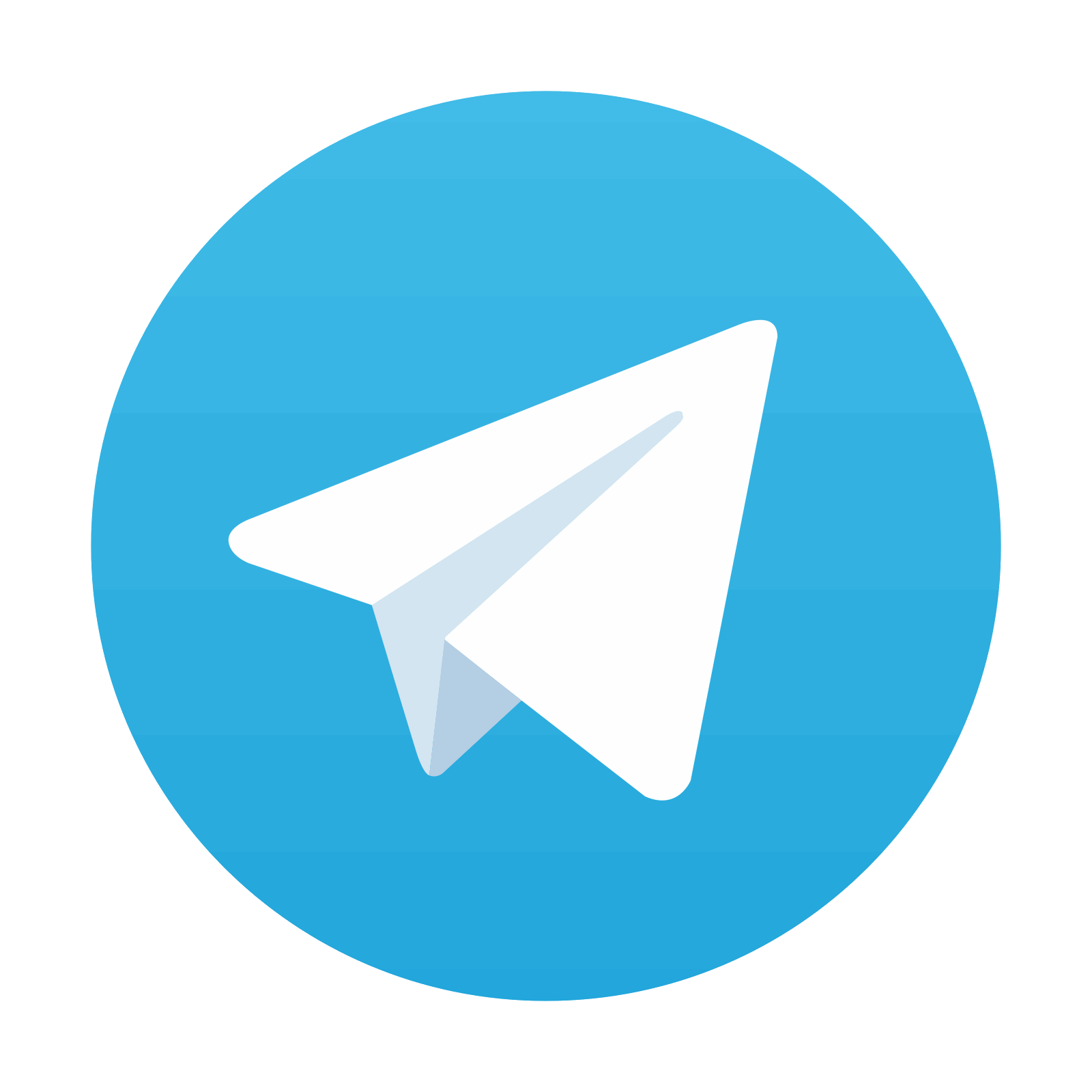
Stay updated, free articles. Join our Telegram channel

Full access? Get Clinical Tree
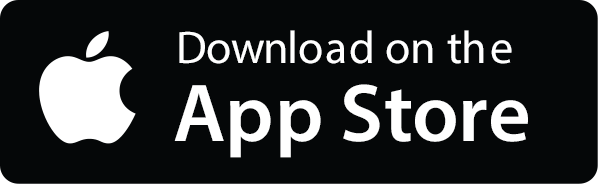
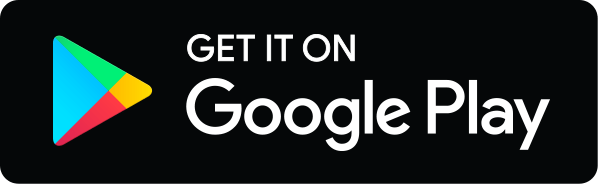