Chapter 6 Salient aspects of normal function of the torso
The spine or ‘backbone’ is the segmented connecting rod of the body common to all vertebrates. However, man is unique, in being the only one to have evolved to a consistent upright posture on two legs upon which the spine is further required to function both vertically and horizontally in relation to the earth’s surface while balancing its super incumbent load. More specialized function of the limbs has evolved more complex function in the proximal limb girdles and as we have seen their control is intimately related to control of the spine. Rolf1 describes the spine as the ‘vital core that integrates the human with his gravity environment poorly, well or adequately as the case may be’. Its function is complex and various aspects considered important in facilitating improvement in its control are explored. This is examined within four main functional components:
Part A: The axial spine
Structural mechanical aspects
The spine performs many roles
The spine is a remarkable piece of structural bio-engineering acting as the central support yet also capable of assuming different shapes in multiple planes. It has been described as an unstable structure stabilized by the nervous system.2 Its roles can be essentially distilled as providing:
The requirement for both effective stability and mobility is achieved through the interdependent function between the nervous, myofascial and osseoligamentous systems as has been suggested by Janda4 and White and Panjabi.5
The spine is more centrally located within the body than is generally appreciated. Its pyramidal shape and frontal plane symmetry means it can support, carry and control all its superimposed weights.6 Maintaining control of the regions where the curves change, particularly the thoracolumbar and lumbosacral is important in balancing the structure of the lower spine. The natural state of the lumbar spine is arched. Gracovetsky3 and Farfan7 have long stressed the biomechanical advantage of the lumbar lordosis and hip extension for effective upright activity.
A neutral spine and the ‘neutral zone’
While the spine can readily change its form, it is important that it is able to return to ‘home base’ or its neutral alignment. Here, the curves assume their physiologic position and act to balance one another; the head is balanced over the pelvis such that there is minimal displacement from the line of gravity, and minimal muscle work is needed to maintain the position. There is balance of all segments in all planes. The curves are characterized by a range defined by natural variability.8 This can also be influenced by differing postural habits, body types and training effect and so on. Clinically, those with spinal pain have altered perception of a neutral spine and difficulty achieving this throughout the spine.
The term ‘neutral zone’ was conceived by White and Panjabi5 who defined control of the neutral zone as ‘a low load response near or beyond the neutral position…. up to the beginning of significant resistance…due to the application of a small force’.5 Movement further into range is into the ‘elastic zone’ up to the physiological limit, after which, ‘failure’ occurs. This is more likely with the adoption of end range postures with or without superimposed movements. White and Panjabi5 state ‘a significant amount of spinal motion takes place around the neutral position (which) is not accounted for’. In most usual everyday activities, a lot of the required spinal movement ideally oscillates in and around the neutral zone or ‘mid position’ of every spinal segment in the axial column under fairly low loads and is directionally balanced. This is important to recognize when facilitating functional neuromuscular control of the spine. The active control of these small low load movements is largely performed by the systemic local muscle system (SLMS). Larger loads and movements into the ‘elastic zone’ involve more activity from the SGMS (see Ch. 5).
The spine comprises multiple functional spinal units (FSU)
Otherwise known as the motion segment, the FSU is the smallest segment of the spine that exhibits the biomechanical characteristics similar to those of the whole spine.5 It consists of two adjacent vertebrae, the articulating surfaces between them – the two facet joints, separated by an intervertebral disc, and the connecting ligamentous tissues. The behavior of the FSU is dependent upon the physical properties of each of these components. Generally speaking, motion at any FSU is extremely limited and consists of a small amount of gliding (translation) and rotation.9 This occurs from a combination of rocking through the disc30 and sliding of the facets which act to steer the movement. The total behavior of the spine results from the composite behaviors of the multiple FSUs connected in series which constitute its structure.
Significantly, between each intervertebral level, the spinal nerve exits through the intervertebral foramen (Fig. 6.1). Here it has important relations. In the front is the intervertebral disc and adjacent regions of the vertebral body. Behind are the facet or zygapophysial joints.10 Like any synovial joints, these can become inflamed and swollen when the vertebral mechanics change. This spinal joint inflammation not only disturbs local joint function but can also compromise the lumen of the foramen and directly impact upon the spinal nerve creating local and referred pain syndromes (Ch.12). The marked implications of facet inflammation are understood when injecting saline into the joints of pigs produced an immediate reduction in paraspinal muscle activity.11 Clinically, most spinal pain and related disorders stem from dysfunction of one (or multiple) FSUs where, through altered loading, both the disc and the facet are implicated. When the disc loses height the facet mechanics change. Effective clinical interventions generally result from restoring the movement in the facet and through the segment which reduces the inflammation and so the pain.
Movements of the axial spine
Spinal movements result from the contribution of small movements in some or all of the FSUs. Spinal motion is generally described as flexion/ extension, lateral bending and rotation occurring in the sagittal, frontal and horizontal planes respectively. Lateral bending produces translation and rotation of the vertebrae in the frontal plane as well as axial rotation because of the inherent properties of the FSU.5
Kinematic differences thus occur within each region of the spine and in depth analyses are provided by numerous authors.5,9,12 Figure 6.2 shows the segmental and regional movement characteristics.
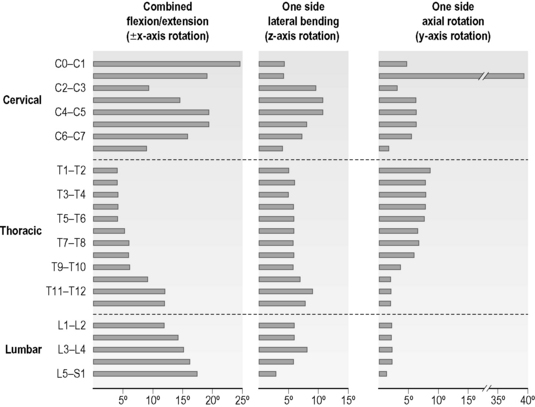
Fig 6.2 • Composite summary of segmental kinematics and regional variations.
Reproduced from White AA and Panjabi MM. 1990 with permission JB. Lippincott & Co.
A decrease in the range of all spinal movements with increasing age is apparent.19
Pelvic (sacral) spatial position affects spinal alignment
In the frontal plane when the pelvis is in the neutral balanced position the spine is vertical, symmetrical and balanced. If the pelvis is oblique or laterally tilted, the pelvis will shift to the high side, the lumbar spine will side bend to that side. This in turn will then create compensatory adjustments throughout the spine – a pronounced case of which is seen in scoliosis.23
In the sagittal plane the lumbar spinal curvature is clearly dependent upon pelvic tilt.23 A neutral lordosis is achieved with a corresponding neutral tilt of the pelvis. A neutral pelvis has been variously defined:
Mutual behavior between the curves
A change in one curve will generally be reflected in the others. Try lying down on the floor with your knees bent. If you flatten your low back to the floor, invariably your chin will poke forward and your neck hyper extend. If you increase the lumbar lordosis the neck will lengthen and the chin retract. This has implications for clinical practice. Black et al.22 showed this opposite relationship between lumbar and cervical posture in sitting – as the lumbar spine moved toward extension, the cervical spine flexed and vice versa. Marked stiffness in the thoracic spine will modify this response.
The junctional regions
According to Lewit,23 not all vertebral segments have the same importance for the functioning of the spine. There are ‘key segments or regions’, mostly the transition areas where the anatomy and the function in the column changes between very mobile and relatively immobile regions. These ‘junctional regions’ marry the transitions between the three principal units of body weight – the head thorax and pelvis. They accommodate forces while transmitting movement through the spine. They occur at the top and bottom of the segmented spine and the top and bottom of the thorax. Ideally, the line of gravity passes through them.24,25 Lewit maintains it is in these regions that the spinal column suffers first jeopardizing function of the spine as a whole, and causing secondary lesions.23 They all share a common tendency to hypomobility or stiffness creating compensatory relative increased mobility in segments adjacent and/or far removed.
The head–tail bone relationship
The head and the tail bone can be viewed as functional ‘limbs’. Thus there are six ‘limbs’ from which spinal movement may be initiated. Additionally the spine transmits sequences and adjusts movements between the upper and lower body and between the various ‘limbs’. Hackney28 notes that in all movements both simple and complex, where the spine is a dynamic link between the limbs and the upper and lower body, the ‘head and tail are in a constant and always changing interactive relationship’.
Spinal loading and the control of forces in movement
White and Panjabi5 define load as ‘a general term describing the application of a force and/or moment (torque) to a structure’. All structures including the body are subject to forces and in the physical universe action and reaction are always equal and opposite. Movement is loading. It can occur in response forces acting on the body which in turn creates other forces which further need to be controlled. While ‘forces’ and ‘loads’ may imply the idea of ‘maximal’, in the spine they can be minimal occasioning merely a tonus shift to counter them.
Interested in structural balance and the mechanical forces which affected this, Todd6 says ‘the direction in which any force acts upon an object in relation to its internal axis determines the nature of the stress endured by the object’. She lists ‘axial and other stresses’.
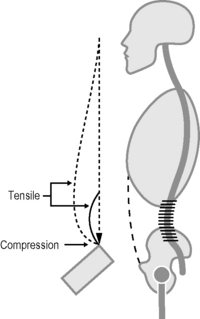
Fig 6.4 • Vertebral column as a spring. Balance of tensile and compression forces in the axial spine.
Reproduced from “The Thinking Body” Mabel E Todd 1937 with permission from Princeton book company.
The effect that loading has on the spine will also depend on the duration and the magnitude of the force. These can be divided into two main categories:5
The role of the ‘passive tissues’
These provide structural support and help counter the applied forces to the spine. Importantly, most exhibit viscoelastic behavior, in particular the disc. This means that when a constant load is applied to the tissues, over time they will deform or ‘creep’. The tissues are variably sensitive to the rate that the load is applied. The load can be tensile or compressive. When the load is removed the tissue may not recover and return to its origin dimensions, known as ‘hysteresis’. Both creep and hysteresis have been shown to increase with age.29 These phenomena play a significant role in spinal pain syndromes. The adoption of habitual altered posturomovement strategies creates chronic aberrant loading of the tissues.
The vertebrae
The architecture of vertebrae makes them well designed to bear compressive loads, most of which is taken through the vertebral body although some occurs through the facet joints.5,30 The vertebral bodies become progressively larger at the bottom of the spine and studies have shown these to be stronger in compressive loading as may be expected. Bone mineral content has been shown to increase in response to mechanical demand.31 In general though, vertebral strength decreases with age.5
The facet joints or posterior intervertebral joints30 contribute to load sharing. The amount of compressive load borne will depend upon the spinal posture and has been shown to vary between nil (becomes tension loading in marked flexion) and nearly half the load in extension.5 Their geometry affords them a significant role in checking the tensile, torsional, shear and bending stresses that the spine is subject to.
The intervertebral disc
Its design provides for the combined roles of weight bearing and load transference while allowing multidirectional rocking movements between the vertebrae. Besides compression, the dense annular fibres act like strong ligaments and help resist tensile, torsional, bending and shear stresses. However, combined loads make it more vulnerable. Flexion bending and torsional loads are probably more harmful than compression,5 although both cyclic and high compressive forces in hyperextension have demonstrated disc damage.32 The disc also has the capacity to absorb and store energy, has elastic recoil and provides shock absorption30 and damping through the spine. In its healthy state, acting as a tense pillow between the vertebrae, it assists the correct apposition of the facet joints and so helps ensure their function and stability. Intradiscal pressure within normal physiological ranges appears to provide an essential mechanical stimulus for maintenance of the proteoglycan matrix and the consequent load bearing capacity of the disc.33 As we age, the disc degenerates. This can be apparent from the age of 20 and by the age of 50, 97% of all lumbar discs have been shown to be degenerated, in particular the lowest three levels.5 When the disc degenerates, the facet joints tend to stiffen or can override, become sloppy and ‘unstable’. Segmental dysfunction involves the whole FSU – problems with the disc involve the facets and vice versa. It is quite extraordinary how this one structure has been assumed to be the mainstay of most low back pain. Treatment directed at the movement impairment of the whole segment invariably ameliorates the pain while the radiological changes remain the same, being usual and age related.
Ligaments
Binding the vertebrae together, ligaments readily resist tensile forces in the direction in which the fibres run. As such they are also good at resisting torsion, shear and bending. With the muscles, they share the role of providing stability to the spine within its physiologic curves and ranges of motion including maintaining the relationship between each vertebra, protecting the spinal cord and nerve roots. They demonstrate adaptive changes in response to increasing demand or immobilization.34 They are considered sensory organs and have significant input to sensation and reflexive/synergistic activation of muscles.35 Solomonow et al.36 demonstrated that mechanical overload of spinal ligaments recruits local muscles in a protective response which increases with the ligament stress. ‘The functional complexity of ligaments is amplified when considering their inherent viscoelastic properties such as creep, tension–relaxation, hysteresis and time or frequency-dependent length-tension behavior’.35 Dysfunctional ligaments thus result in various sensorimotor disorders.
The dynamic role of the neuromyofascial system
Fascial system
The thoracolumbar fascia has been ascribed a biomechanical role in the stability of the lumbar spine. In fact, Gracovetsky37 considers it the most important structure insuring the integrity of the spinal machinery. Traditionally though, fascia has not been considered as a system and accorded little importance in musculoskeletal mechanics. However, there is growing interest in the potential major role that fascia may play in providing structural support, stability and contributing to movement coordination. It has been described as:
The soft tissue component of the connective tissue system that permeates the human body forms a whole body, continuous three-dimensional matrix of structural support. It interpenetrates and surrounds all organs, muscles, bones and nerve fibres, creating a unique environment for body systems functioning. It includes all fibrous connective tissues, including aponeuroses, ligaments, tendons, retinacula, joint capsules, organ and vessel tunics, the epineurium, the meninges, the periostia and all the endomysial and intermuscular fibres of the myofascia.38
According to Rolf,1 there are different kinds of fascial sheaths. The superficial is a fibroareolar tissue that houses much of the body fats, can stretch in any direction and adjust quickly to strains of all kinds. The deep fascia is a denser layer, provides good resistance to tensile strain and its smooth coating permits neighbouring structures to slide over one another. She was particularly interested in the fascial envelope surrounding muscle. Rolf1 and her disciples and those interested in biotensegrity39 view the fascia as the prime organ of support and the bones, rather than providing support in the Newtonian sense, act as spacers serving to position and relate different areas of connective tissue. This may help explain how the ‘human spine can accept loads from any direction with arms and legs cantilevered out in any direction’.39 Muscles provide the source and direction of movement and as they are encased within it and attach to it, they can act as tensioners to the system. Variously described as the ‘internet’,40 ‘the endless web’,41 ‘a spider’s web reaching out to every nook and cranny’,42 being a continuous system – a force applied to one part or a local restriction – can have quite far reaching as well as local effects. The anatomical continuity of the myofascial and viscerofascial systems as well as the neuroanatomical relationship of somatic and visceral structures mean that recruitment patterns of spinal muscles may change due to dysfunction of structures outside the musculoskeletal system.42
Artificially increasing the tension in the lumbar fascia has been shown to alter lumbar segmental translation and rotation.43 Fascia is also capable of remodeling in response to changing mechanical loads and muscle activity.44 Recent research has demonstrated the presence of smooth muscle like contractile cells in fascia with higher densities found in the lumbar fascia indicating its ability to influence musculoskeletal mechanics.45 Smith46 provides a seductive view of its role: ‘fascia is now seen as an antagonist to muscular action, and movement is seen less as the coordinated action of antagonistic muscles and more in terms of the elastic and oscillatory properties of the myofascial network as a whole’. As well as its static attributes, fascia ‘has the potential for certain rhythmic or oscillatory movement patterns that arise from its elastic, hydraulic and tensegrity properties’. ‘These inherent rhythmic movement patterns are independent of muscle activity. However, they may be either reinforced or inhibited by muscle action’. ‘Muscular action works primarily to maintain the oscillatory patterns with an occasional and timely input of energy each movement cycle’. The whole body response seen in Craniosacral Therapy47 is based upon the concept of a fascial continuity throughout the body. Gracovetsky48 remarks ‘it is the viscoelastic behavior of collagen that drives the stability of the spine… the integrity of the collagen structure is as important as that of the muscles’. The fascial system appears to serve as the structural and functional link between the frank passive and active tissues in movement.
Neuromuscular system
This interdependent system converts a structural body into a functional body.
In our relationship with gravity, the body is constantly adjusting to changing circumstances. We do this through information coming from our senses, from within (proprioception) and without (exteroception). Proprioception essentially provides position and movement sense. It allows us to sense the position and movement of joints; to sense the force effort and heaviness associated with muscle contractions; and the ability to perceive the timing of muscle actions.49 Exteroception comes through the senses with which we orient ourselves to the environment and the sense of space around us – vision labyrinths and touch.
Effective motor control is dependent upon adequate peripheral afference from receptors in the joints, ligaments, fascia muscles tendons etc so that the magnitude and timing of the muscle response is appropriate to the loading conditions. The various kinesthetic receptors are in tissues which are viscoelastic and the adoption of slouched postures has been shown to subsequently affect subjects’ ability to reposition their spines.50 Normally we can accurately spatially position our spine and this is independent of the magnitude of the range of movement.51 Brumagne52 has shown that precise muscle spindle input from multifidus is essential for accurate positioning of the pelvis and lumbosacral spine in sitting.
The neuromuscular system essentially deals with balancing the intrinsic and extrinsic forces imposed upon the body as a result of gravity, movement and loads. This system is fragile and its dysfunction, according to Janda,53 is one of the first signs to be clinically recognizable and is responsible for the genesis of many spinal pain disorders. There are certain normal neuromuscular responses relating to spinal control which merit looking at.
Aspects of normal neuromuscular behavior around the spine
Muscle coactivation or cocontraction (see Ch. 4)
This is a normal muscle response from the trunk muscles in order that the spine may be controlled in a stable neutral posture54 and ready to respond to the complex and unexpected loading patterns during our everyday activities. The column is supported and controlled from all sides. Activity at low load states is primarily in the deep SLMS and as the load increases so does the magnitude of the co-activation, as the superficial muscles become more involved. Antagonistic trunk muscle coactivation occurs during sudden loading of the torso, accelerations of the torso as in slipping, isometric trunk moments, axial torques and heavy exertions in order to provide the necessary stability.54 Co-contraction is a normal response to fatigue as the spine attempts to protect itself.55 However, Radebold et al.56 showed that sudden perturbations to the trunk in normal people produced more flexible muscle recruitment responses in the superficial muscles and did not necessarily involve co-contraction. Their study did not access the deeper muscles.
At high loads, trunk muscle coactivation resembles muscle guarding or splinting and spinal motion is limited. Quint et al.57 showed this can be reduced by as much as 20%. High levels of co-contraction have been shown to degrade postural control.58 While stabilizing the spine to manage heavy loads, it imparts a high compressive loading on the spine. In the normal state this is not a problem, as they are generally short lived to say lift a box, or prevent a fall.
Flexion relaxation phenomenon
Studies have shown that when standing still and bending forward, the low back extensors eccentrically contract and then fall electrically silent, the load then being borne by the passive tissues – ligaments, disc, gut and some elastic recoil in the muscles,59 and the fascial system.60 The point in the flexion range where this occurs is variably reported as between two-thirds of maximum trunk flexion (and corresponding half-range hip flexion),61 to near the end of trunk flexion.59 The relaxation effect has also been reported to include the hamstrings and much of the thoracic erector spinae.60 However, relaxation of the lumbar extensors associated with reciprocal thoracic erector spinae activation has also been found.62 Bogduk et al.63 estimate that the thoracic erector spinae contribute to 50% of the total extensor moment exerted on L4/5. Andersson et al.64 found that quadratus lumborum did not relax with the lumbar extensors suggesting a postural stabilizing role for this muscle.59
Limiting the posterior shift of the pelvis and hip flexion has been shown to produce the response earlier in range.65 While a lack of this response is a common finding in subjects with LBP suggesting an increased ‘protective’ role from the superficial extensors, it is not universal. The question needs to be asked as to the desirability of depending on the passive tissues. In functional movement, is the flexion relaxation response different? Is it partly a consequence of decreased SLMS coactivation synergies affording poor adaptable support and control where limiting pelvic shift and locking the legs allows one to utilize the passive system more than is healthy?
O’Sullivan et al66 have examined the response when moving from sitting upright to slumped sitting. They found that superficial multifidus and internal oblique – both important in segmental control, exhibited a consistent and significant decrease in activity at mid range spinal flexion. The thoracic erector spinae response was highly variable with several different patterns of activity demonstrating the variable and complex nature of motor control. They found that adopting a neutral lordosis in sitting, best facilitated activity in the deep system muscles.
The reflex muscular responses to arousal/stress
Arousal, a state of internal alertness, is a component of several emotional responses including fear and anxiety and is mediated by the neuroendocrine system and includes the limbic system.67 The level of arousal can vary from deep sleep to the fight-or-flight response. The body and mind are inextricably linked, our emotions being reflected in our neuromuscular being. Stress is a natural phenomenon and necessary for our survival. There is a normal cyclic variation between periods of stress when we are aroused and periods of relaxation where depleted energy is restored. In times of stress or hyper arousal, the sympathetic system is activated initiating a ‘fight or flight response’. This occasions an increase in the cardiac and respiratory rate and other bodily changes to get ready for action. Hyperventilation is part of this normal reaction to sudden danger or excitement. There is over activity of the general body musculature, particularly of the facial and jaw muscles, neck and shoulders. Activity in the SGMS predominates. The upper limbs are held in flexion, the trunk is generally held stiff and because of tension in the abdominal musculature, diaphragmatic breathing can be inhibited and replaced by upper chest breathing.68 Classically adduction and flexion patterns are symptomatic of stress.68,74 The higher levels of muscle activation found in states of increased arousal have been shown to decrease performance in highly anxious subjects yet improve it in those less anxious.67
Repeated exposure to stress creates a risk that stress changes from a natural transient reaction to a chronic pathological state. The person finds it difficult to relax. Symptoms such as hyperventilation syndrome become common and will have effects on the whole body including the neuromuscular system.69,70,71 The line between normal and abnormal behavior becomes blurred.
The protective and defensive reactions
It is a basic instinct of all animals and humans to protect or defend themselves when threatened.
Hanna72 was influenced by Selye’s statement that stress is a response to good things as well as bad. He describes stress as being both positive and negative and creating a specific reflex response of the neuromuscular system in two basic ways:
Bond40 suggests that the horizontal, crosswise muscles (the vocal, thoracic and pelvic diaphragms) are the sites of our most internal motions of protection as they constrict whenever we hunker down under pressure. Acting like valves through the vertical axis of the body, their closure blocks the adaptive responses to gravity through the body. Again, these reactions are characterized by associated over activity in the superficial systemic global muscle system (SGMS). When repeatedly triggered, which is indeed common in today’s stress inducing society, these basic responses easily become habitual behavior. As Hanna says:
Habituation is the simplest form of learning. It occurs through the constant repetition of a response. When the same bodily response occurs over and over again, its pattern is gradually ‘learned’ at an unconscious level. Habituation is a slow relentless adaptive act which ingrains itself into the functional patterns of the central nervous system.72
And this occurs in ‘normal’ ‘healthy’ as yet ‘pain free’ people!
Effort response
Movement should on the whole be easy and effortless, achieved from well integrated activity between the SLMS and the SGMS. When performing a task that requires a high level of effort, there is a spread of activation to other muscles besides those principally responsible for the task. This enhances postural stability and enables the transfer of power across joints by the two-joint muscles.67
Laban73 was interested in the economy of effort afforded by the use of appropriate motor skills. Efficiency is gauging the right proportionality of weight, space, timing and control of the flow of movement. The inherent rhythm in movement is important and is disturbed by the use of excess and unnecessary effort. ‘Any inappropriate use of movement is just a waste of effort.’73 The harder we work the more the SGMS is called into action, a feature of their innate behavior (see Ch.5). In times of higher loading they serve to provide the added stiffness the spine needs. However, the amount of effort expended needs to match the demand of the situation. People with a poorly integrated systemic local muscle system rely more on their SGMS and so tend to use more effort than necessary in low load activities and with unhealthy kinematic patterns. Importantly, those actions requiring increased effort, should involve the adoption of biomechanically sound movement patterns.