115 Renal Replacement Therapy
Principles of Renal Replacement Therapy
The principles of renal replacement therapy have been extensively studied and described.1–3 The two fundamental principles of renal replacement therapy particularly relevant to critical care physicians are summarized here.
Water Removal
Solute Removal
where J is solute flux, D is diffusion coefficient, T is temperature of the solution, A is membrane surface area, dc is concentration gradient between the two compartments, and dx is diffusion distance (thickness of the membrane). In dialysis, blood and dialysate are separated by a membrane. Bidirectional diffusive transport of molecules occurs in response to a concentration gradient.
where Qf is filtration, Km is coefficient of permeability of the membrane, TMP is transmembrane pressure, Pb is hydrostatic pressure of blood, Puf is hydrostatic pressure in the ultrafiltrate compartment, and π is oncotic pressure of blood. In convective treatments, the transport (Jc) of solute x is governed by the formula:
Despite these distinctions, diffusion and convection often act simultaneously, and it is almost impossible to divide these transport mechanisms physically. The term hemodialysis may not aptly describe the mode of treatment in the case of highly permeable membranes. A more suitable term would be hemodiafiltration (if replacement solution is needed) or high-flux dialysis (if a filtration-back filtration mechanism is present and no replacement fluid is required). The various modalities are described in Figure 115-1.
Indications for Renal Replacement Therapy
The treatment of AKI requires a different style and philosophy from renal replacement therapy for chronic renal failure. In a critically ill patient, renal replacement therapy should be initiated early. It is physiologically irrational and clinically dangerous to wait for complications to appear before intervening. Fear of early dialysis stems from the well-known adverse effects of conventional intermittent hemodialysis with cuprophane membranes, especially hemodynamic instability, and from the risks and limitations of continuous or intermittent peritoneal dialysis.4–7 If extended dialysis techniques are used, they are minimized.8 Accordingly, the time-honored criteria for initiation of renal replacement therapy in patients with chronic renal failure may be inappropriate in critically ill patients.9 Modern criteria for initiation of renal replacement therapy in the intensive care unit (ICU) are presented in Table 115-1.
TABLE 115-1 Modern Criteria for Initiation of Renal Replacement Therapy in the ICU*
* If one criterion is present, renal replacement therapy should be considered. If two criteria are simultaneously present, renal replacement therapy is strongly recommended.
Once intermittent hemodialysis or continuous hemofiltration has been started, there are limited data on what is an “adequate” dose of dialysis. The concept of dialysis adequacy in AKI remains controversial and ill defined, and the current goal is maintenance of homeostasis at all levels.10 Emerging data suggest that better uremic control may translate into better survival.11–13 Patients at least should have urea levels maintained between 10 and 20 mmol/L throughout the treatment period. This level of uremic control should occur despite adequate nutrition support with a protein intake around 1.5 g/kg/d. If intermittent hemodialysis is used, daily treatment and/or extended treatment are more desirable,8 with the goal of ensuring at least some adequacy for small-solute removal. This means intermittent hemodialysis must guarantee at least a daily urea clearance in liters greater than or equal to the patient’s total body water. Total body water can be calculated from tables or simply as 60% of body weight. This relationship will be treated extensively in the following discussion.
Continuous Renal Replacement Therapy
Continuous renal replacement therapy (CRRT) is now the most common form of renal replacement therapy in Australian and European ICUs. In the United States, however, CRRT reportedly is used in only 10% to 20% of ICU patients.14 CRRT has undergone several technical modifications since it was first described in 1977. Initially it was performed as an arteriovenous therapy (continuous arteriovenous hemofiltration) in which blood flow through the hemofilter was driven by the patient’s blood pressure. Clearances were low, however, and countercurrent dialysate flow soon was added to double or triple solute clearances (continuous arteriovenous hemodialysis/diafiltration) with or without spontaneous ultrafiltration. Double-lumen catheters and peristaltic blood pumps have come into use with or without control of ultrafiltration rate.
Whatever the technique of CRRT, the clearances achieved can be adjusted by adjusting ultrafiltration rate or dialysate flow rate or both, typically aiming to achieve a daily clearance at least equal to the patient’s total body water. A standardized nomenclature is now available for CRRT techniques.15 To make the reading easy and to make the reader familiar with the most accepted definitions and treatment schemes, we have summarized in Figure 115-2 the complete set of available techniques, including some hints on operational parameters. No matter what technique is used, the following outcomes are predictable, and the most important will be described:
All critically ill patients need a high daily amount of volume infusions: blood and fresh frozen plasma, vasopressors and other continuous infusions, parenteral and enteral nutrition, which should be delivered without restriction or interruption. It is not uncommon for patients with AKI and associated septic shock to receive large amounts of fluid resuscitation, leading to fluid overload. The consequent positive fluid balance and tendency to interstitial edema causes the necessity for water removal and possibly the achievement of a negative daily fluid balance. Extracorporeal renal replacement therapies are typically utilized for ultrafiltration. Ultrafiltered water has a similar osmolarity to plasma water; for this reason, the process of “isolated ultrafiltration” substantially corresponds to blood dehydration, with possible increase of hematocrit values and smallest modification of solutes concentration.16 CRRT slowly and continuously removes a patient’s plasma water, mimicking urine output, whereas thrice-weekly intermittent hemodialysis must extract in few hours the equivalent of 2 days of administered fluids plus excess body water that may be present in the anuric patient. Intravascular volume depletion associated with excessive ultrafiltration rate is due to both the high rate of fluid removal required and the transcellular and interstitial fluid shifts caused by the rapid dialytic loss of solute. The major consequence of rapid fluid removal is hemodynamic instability. Consider the case of a septic patient with AKI who is receiving a high amount of vasopressors because of hemodynamic instability and needs appropriate fluid resuscitation, supplementation of nutrition, and blood product administration. The renal replacement modality of choice seems to be the one that warrants slow fluid removal, prolonged for many hours a day, to easily meet the highly variable required daily fluid balance. In particular, when volemic and uremic control is not a problem, an aggressive protein-rich nutritional policy (1.5–2.5 g/d) can be implemented in the care of AKI patients receiving CRRT, resulting in a marked improvement in daily nitrogen balance with possible favorable effects on immune function and overall outcome.17 Safe prescription of fluid loss during renal replacement therapy requires intimate knowledge of the patient’s underlying condition, understanding of the process of ultrafiltration, and close monitoring of the patient’s cardiovascular response to fluid removal. To preserve tissue perfusion in patients with AKI, it is important to optimize fluid balance by removing the patient’s excess water without compromising effective circulating fluid volume. It is still a matter of controversy which clinical parameter (actual patient weight/patient dry weight, mean arterial pressure, central venous pressure, wedge pressure, systemic saturation, mixed venous saturation, bioimpedance, etc.) or currently available monitoring (central venous catheter, Swan-Ganz catheter, transesophageal echocardiography, etc.) should be utilized to uniformly define the concept of “volume overload.” In patients who are clinically fluid overloaded, however, it is extremely important to accurately evaluate the amount of fluid to remove18; one of the main features of slow and constant ultrafiltration is the possibility for interstitial fluid to slowly and constantly refill the “dehydrated” bloodstream. This phenomenon is driven by hydrostatic and osmotic forces and allows for elimination of high plasma water volumes per day, with a reduced risk of hypovolemia and hypotension. In critically ill children, correction of water overload is considered a priority; it has been shown and recently confirmed that restoring adequate water content in small children is the main independent variable for outcome prediction.19 Similar results have been recently found in a large cohort of adult critically ill patients with AKI.20
One of the measures utilized to quantify urea/creatinine removal is dialysis dose. One of the main aspects of dose to be understood is the concept of clearance (K): K is the volume of blood cleared from a given solute over a given time. K does not reflect the overall solute removal rate (mass transfer) but rather its value normalized by the serum concentration. Even when K remains stable over time, the removal rate will vary if the blood levels of the reference molecule change. K depends on solute molecular size, intercompartmental transmittance (Kc), transport modality (diffusion or convection), and circuit operational characteristics (blood flow rate, dialysate flow rate, ultrafiltration rate, hemodialyzer type, and size). As originally conceived, K is utilized to evaluate renal function among disparate individuals whose kidneys are operating 24 hours a day and urea/creatinine blood levels are at steady state. For this reason, the concept of K is easily applicable to continuous treatments, and its use to describe intermittent therapy efficiency is a sort of adaptation. Because K represents only the instantaneous efficiency of the system, during treatments with different time schedules, information about the time span during which K is delivered is fundamental to compare the different RRT doses. For example, K is typically higher in IHD than in CRRT and sustained low-efficiency daily dialysis. However, daily mass removal may be greater during CRRT or sustained low-efficiency daily dialysis because the K is applied for 12/24 hours (Table 115-2). In any case, from a physiologic point of view, even if a continuous and an intermittent therapy were prescribed in order to provide exactly the same marker solute removal, still they could not be comparable: during continuous treatments, where a relatively low K is applied, a slow but prolonged removal of solutes approaches a pseudo-steady state slope (Figure 115-3). In highly intermittent therapies, the intensive K, limited to 4 to 6 hours per day, thrice a week, causes the sawtooth slope in solute removal and eventual rebound during the time span without treatment. These peaks and valleys of solutes, bicarbonate, electrolytes, plasma osmolarity, and volemia are not physiologic and might have a detrimental impact on a patient’s hemodynamics and the balance of electrolytes, acid-base, and other “osmoles.” Furthermore, in the case of intermittent hemodialysis, the Kc (i.e., the variable tendency of different tissues to “release” a solute into the bloodstream) is much more relevant than during low-efficiency treatments. As a matter of fact, solute control is optimized during CRRT.
< div class='tao-gold-member'>
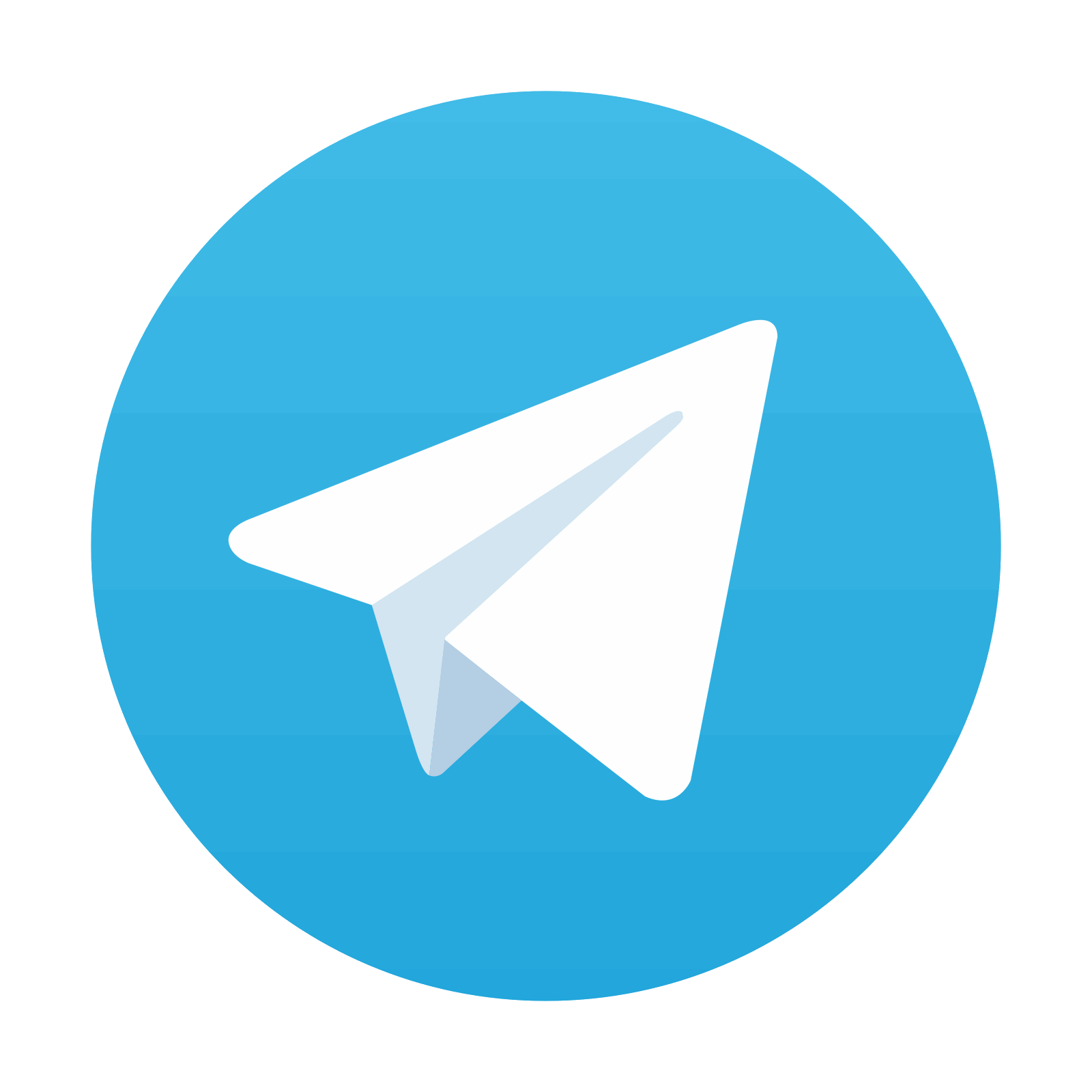
Stay updated, free articles. Join our Telegram channel

Full access? Get Clinical Tree
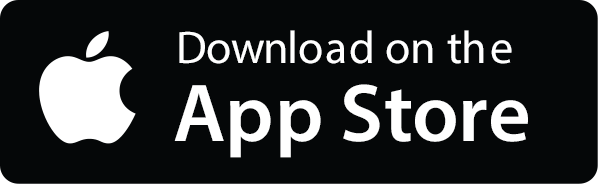
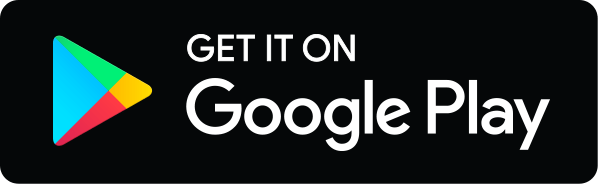