Pulmonary Edema
Avner Sidi
Emilio B. Lobato
CASE SUMMARY
A 24-year-old man known to be an addict was admitted for mandibular fracture repair after facial trauma. The patient admitted using heroin, cocaine, and “crack.” In the emergency room, he appeared to be mildly sedated, but oriented and coherent, without any complaints or symptoms. The patient was brought for surgery without completing his full toxicology screen. A rapid sequence induction with cricoid pressure was performed. Following endotracheal intubation, pink frothy fluid appeared in the tube, associated with a decrease in oxygen saturation from 100% to 90% SO2 despite an FiO2 of 1.0. Ten cm of positive end-expiratory pressure (PEEP) were added with some improvement. Surgery was postponed, and the patient was taken to the intensive care unit (ICU). Chest radiograph demonstrated pulmonary edema with widespread, patchy, bilateral airspace consolidations, ill-defined vessels, and peribronchial cuffing. The diagnosis of heroin-induced pulmonary edema was made, not associated with renal insufficiency or aspiration of gastric contents. Rapid resolution of the infiltrates was observed within two days, with no parenchymal sequelae. The patient then underwent surgery without complications.
What Is the Incidence of Perioperative Pulmonary Edema?
Pulmonary edema can occur preoperatively, intraoperatively, or postoperatively. It is defined as an abnormal accumulation of fluids in the extravascular lung space, and is associated with changes in lung volume, mechanics, and gas exchange. Pulmonary edema can result from various causes or conditions, and is potentially lethal.
The clinical presentation of perioperative pulmonary edema is relatively infrequent, with a calculated incidence of 0.2% to 7.6%, according to the type or group of patients and the severity of the underlying disease.1,2,3 In a certain subset of patients, 3.6% of whom developed clinical pulmonary edema after noncardiac surgical procedures, the mortality rate was 57%, whereas in patients who developed heart failure without the clinical presentation of edema, mortality was 15%.2 Another group of patients in a major tertiary medical center, with a relatively high incidence of edema (7.6%), had a lower mortality rate of 11.9%.3 The true incidence of pulmonary edema, however, may be greater because of numerous cases of increased extravascular lung water that do not demonstrate an obvious clinical presentation of edema. It is clear, however, that this serious complication has a significant implication on surgical outcome; therefore, anesthesiologists must be familiar with its diagnosis and management. Early diagnosis and appropriate care are the keys for improving perioperative outcome.
What Is the Clinical Presentation of Pulmonary Edema?
Pulmonary edema can be classified into three stages. The first involves accumulation of extravascular lung water in the interstitial space. Cuffs of fluid appear around the bronchi and blood vessels, without severe impairment of oxygenation. During the second stage, fluid enters the alveoli, and hypoxemia appears because of increased ventilation/perfusion mismatching. The third and most dramatic stage is airway flooding. With increased lung water, there is a reduction in compliance, increased work of breathing, and smaller functional residual capacity.
During the development stages of edema, the patient becomes tachypneic from attempting to compensate for the reduced lung volume. Clinical signs of increased inspiratory effort will appear: anxiety, alae nasi, and suprasternal and intercostal retractions. Patients present
with rapidly progressive dyspnea, orthopnea, and with or without hemoptysis. In rare instances, large amounts of frothy pink sputum may be seen. Cyanosis and hypoxemia can be present and may remain unresponsive to oxygen therapy.
with rapidly progressive dyspnea, orthopnea, and with or without hemoptysis. In rare instances, large amounts of frothy pink sputum may be seen. Cyanosis and hypoxemia can be present and may remain unresponsive to oxygen therapy.
What Are the Anatomic Considerations?
The structures involved in the pathogenesis of pulmonary edema are the microvascular endothelium, alveolar epithelium, pulmonary interstitium, and pulmonary lymphatic system.
▪ MICROVASCULAR ENDOTHELIUM
The endothelium is composed of a monolayer of cells situated on the basement membrane (located between the endothelium and epithelium). The junctions between endothelial cells contain pores that allow the passage of water and ions. They act as a sieve for protein molecules. Of these molecules, only the smaller ones (i.e., albumin) can pass normally with relative freedom; large molecules (i.e., globulin, fibrinogen) cannot.
▪ ALVEOLAR EPITHELIUM
The alveolar epithelial cells, which are situated on the other side of the basement membrane, are composed of type 1 and 2 pneumocytes. Type 1 are large, flat cells that line the alveoli as a continuous sheet and have tight gaps (pores) in their junctions. Therefore, the alveolar wall is much less permeable than the endothelial wall. This also explains the cases in which pulmonary edema remains in the interstitium without filling the alveoli. Type 2 cells are cuboidal stem cells that produce surfactant.
▪ PULMONARY INTERSTITIUM
The interstitium is the thin layer that lies between the endothelial and alveolar layers. This space contains fibrin, collagen matrix, pulmonary lymphatic vessels, and basement membrane. Its thicker component surrounds the large blood vessels and airways.
▪ PULMONARY LYMPHATIC SYSTEM
The lymphatic vessels are in the interstitium and drain the pulmonary microvascular filtrate.
▪ THE BLOOD-GAS BARRIER
Because of the vulnerability of the blood-gas barrier to stress failure when the capillary pressure rises, it is important to know what is responsible for the strength of the capillary wall. The thin side of the blood-gas barrier consists of the capillary endothelial layer, alveolar epithelial layer, and the extracellular matrix (ECM), which is made up of the fused basement membranes of the two cellular layers. There is strong evidence that most of the strength comes from the ECM, particularly the type IV collagen in the basement membranes. This suggests that the ECM is the strongest layer.4 The thickness of the capillary basement membranes is frequently related to the transmural pressure. For example, patients with mitral stenosis who have an increased pulmonary capillary pressure over several years have thickened basement membranes.5
What Is the Pathophysiology of Pulmonary Edema?
▪ GENERAL FUNCTIONAL-STRUCTURAL FORCES AND MECHANISM
Pulmonary edema is defined as an abnormal accumulation of fluid in the extravascular compartments of the lung. The relative amounts of intravascular and extravascular fluid in the lung are mostly controlled by the permeability of the capillary membrane, as well as the oncotic pressure.6 This relation is described by the Starling equation, which is used to determine the theoretic amount of fluid Qfilt filtered per unit area per unit of time:

In this equation, HPiv and HPev represent the intravascular and extravascular hydrostatic pressure, and OPiv and OPev represent the intravascular and extravascular oncotic pressure, respectively. Kfilt represents the conductance of the capillary wall and expresses the water resistance created by the capillary endothelial cell junctions with changes in HPiv and HPev, whereas t represents the oncotic reflection coefficient and indicates the permeability of the capillary membrane to macromolecules. The greater this reflection coefficient, the more the passage of macromolecules will be restricted, thereby decreasing overall fluid filtration. The net flow, Fnet, is defined as Qfilt– Qlymph, where Qfilt represents fluid transudation or exudation and Qlymph represents lymphatic absorption. Pulmonary edema develops when the equilibrium between fluid transudation or exudation Qfilt and lymphatic absorption Qlymph is disturbed. Therefore, although under normal conditions the endothelial cells are relatively impermeable to protein but remain permeable
to water and solutes, the tight intercellular junctions of the alveolar epithelium remain nearly impermeable to water and solutes, thereby constituting an effective barrier that is a major factor in preventing the development of pulmonary edema. Lymphatic drainage (Qlymph) represents another way of eliminating excess lung water. A manyfold increase in lymphatic flow has been observed with chronically increased hydrostatic pressure. This increase in lymphatic flow is very efficient in eliminating excess water, especially when there is diminished oncotic pressure due to hypoalbuminemia.7 However, its impact requires time, and therefore, it may not be as effective in acute settings.
to water and solutes, the tight intercellular junctions of the alveolar epithelium remain nearly impermeable to water and solutes, thereby constituting an effective barrier that is a major factor in preventing the development of pulmonary edema. Lymphatic drainage (Qlymph) represents another way of eliminating excess lung water. A manyfold increase in lymphatic flow has been observed with chronically increased hydrostatic pressure. This increase in lymphatic flow is very efficient in eliminating excess water, especially when there is diminished oncotic pressure due to hypoalbuminemia.7 However, its impact requires time, and therefore, it may not be as effective in acute settings.
Cardiogenic pulmonary edema (CPE) arises when HPev or the capillary pressure increases secondary to left atrial hypertension, causing transudation of fluid across the interstitium into the alveoli. Noncardiogenic edema most commonly arises when the reflection coefficient (t) is decreased because of endothelial injury. The variable t varies in value from 0 to 1, depending on the restriction of plasma protein by the endothelium. This injury allows the passage of normally restricted plasma proteins from the vascular space into the interstitium and alveoli, creating an exudative edema.
Pulmonary edema can be divided into four main categories on the basis of its pathophysiology:
Increased hydrostatic pressure edema
Permeability edema with diffuse alveolar damage (DAD)
Permeability edema without DAD and
Mixed edema, due to simultaneous increased hydrostatic pressure and permeability changes
This classification scheme is helpful because pulmonary edema is often seen perioperatively in the ICU and emergency department.
Unusual Findings
The clinical and radiologic manifestations of acute pulmonary edema are generally well established. However, pulmonary edema may also demonstrate unusual findings. Atypical pulmonary edema is defined as lung edema with an unusual radiologic appearance but with clinical findings usually associated with well known etiologies. Unusual forms of pulmonary edema are defined as lung edema from unusual causes (e.g., rare diseases, or rare manifestations of common diseases).
▪ STRUCTURE-FUNCTION RELATIONSHIP
The formation and morphology of hydrostatic pulmonary edema appear to be complex, and fluid and protein movement from the microvasculature into the interstitial and alveolar spaces cannot be explained solely by uniform membrane models of fluid exchange. Barrier leaks and, in particular, epithelial cell layer leaks play a role not only in permeability edema, but also in hydrostatic edema. The differences between these two types of edema reflect the type and extent of injury to the cellular layers of the blood-gas barrier, whether there is a causative, associated, or ensuing inflammatory process.
Site and Distribution of Edema Fluid
Chest radiographs of patients with early pulmonary edema usually manifest a rather inhomogeneous, patchy distribution of extravascular fluid accumulation with the apicobasal gradient.8 In addition, the volume of interstitial edema fluid does not correlate with the amount of fluid in the adjacent alveoli. The differences can be explained according to gravity, filtration pressure, and variations in albumin concentrations in the edema fluid. Apparently, the volume of endothelial leaks are not necessarily matched by that of the epithelial leaks. These observations strongly suggest that there are substantial differences in fluid filtrations and in reflection coefficients for macromolecules. Therefore, a simple, uniform membrane model of passive fluid and solute movements following pressure gradients does not necessarily explain how hydrostatic pulmonary edema occurs. This condition is, rather, the result of complex alterations in the structure and function of the central component of the blood-gas barrier (e.g., endothelial and epithelial cell layers), in addition to increased hydrostatic pressure.
Barrier Lesions
There are different types of epithelial lesions on the thick or thin side of the alveolar-capillary barrier. For example, sudden stress on the barrier can damage the endothelial layer by either opening the intercellular junctions or through cellular disruption of the epithelial layer. In this context, ultrastuctural findings in the lungs of patients with hemodynamic pulmonary edema, in particular neurogenic edema, are of interest in that numerous erythrocytes are found in the interstitial and alveolar edema fluid.8 Therefore, the “rusty” sputum produced by patients with lung congestion and edema appears to be of alveolar origin.
Spectrum of Cardiogenic to High-Permeability Edema as Capillary Pressure Increases
In practice, the traditional division between hydrostatic and permeability edema does not always meet expectations. For example, Fein et al.9 pointed out that there is a substantial overlap between the two groups, even in conditions where a pure form of hydrostatic or cardiogenic edema would be expected. Sprung et al.10 showed that there is a continuous fall in the ratio of protein in edema fluid-to-serum when plotted against pulmonary artery wedge pressure for a large group of patients. They referred to it as an “intermediate type of pulmonary edema” on the basis of the protein concentration of the alveolar
fluid, and suggested that a combination of increased permeability and high hydrostatic pressure may account for this intermediate form.
fluid, and suggested that a combination of increased permeability and high hydrostatic pressure may account for this intermediate form.
Initially, as the Starling equilibrium is disturbed, fluid moves from the capillary lumen into the alveolar wall interstitium, and possibly into the alveolar spaces. The result is interstitial edema, and perhaps alveolar as well, with a relatively low protein concentration, so-called hydrostatic or cardiogenic edema.
Figure 12.1 shows that as the capillary pressure is raised to higher levels, the phenomenon known as pore stretching11 can be seen. It has also been demonstrated that when the pulmonary capillary pressure is increased, large tracer molecules such as hemoglobin solution move between capillary endothelial cells into the interstitium of the alveolar wall.12
Finally, even higher pressures stress the blood-gas barrier and cause disruption of the capillary endothelial layer, alveolar epithelial layer, or sometimes all layers of the blood-gas barrier. The result is a high-permeability type of edema.
▪ INTERSTITIAL MECHANICS AND FLUID FLOW
Impaired Alveolar Fluid Clearance
Hypoxia decreases transepithelial sodium transport13 and accounts for decreased fluid clearance from the alveoli of hypoxic rats at an FIO2 of 0.08.14 Transalveolar sodium transport may be stimulated by β2 receptors. A recent field study demonstrated that hydrostatic edema can be successfully prevented with inhalation of salmeterol, a β2 agonist.15 A definitive evaluation of the role of depressed alveolar fluid clearance in the pathophysiology of hydrostatic edema will require more specific drugs. Sartori et al. also found that individuals susceptible to hydrostatic edema had a lower transepithelial potential in normoxic conditions than nonsusceptible controls,15 possibly leading to both lower sodium transport by the epithelial sodium channel and impaired alveolar clearance. These findings may possibly indicate a genetically determined reduction of sodium transport across the respiratory epithelium.
Inflammatory Process
It is conceivable that any process enhancing the permeability of the alveolar-capillary barrier would lower the pressure required to generate edema. Indeed, increased fluid accumulation during hypoxic exposure after priming by endotoxins or viruses in animals,16 and the association of previous viral infections (predominantly of the upper respiratory tract) with hydrostatic pulmonary edema in children,17 support this concept. In the presence of increased permeability, hydrostatic edema may also occur in individuals with a normal pulmonary vascular response to hypoxia.
▪ SURFACTANT-EDEMA INTERACTION
Pulmonary surfactant is a complex lipoprotein structure. Its primary physiologic function is to maintain flow, and reduce surface tension in the alveoli and small airways. The low surface tensions stabilize the lung at low transpulmonary pressures and decrease the work of breathing. Surfactant will improve lung compliance, arterial PO2, and minimum surface tension in animal models. Once edema is present, surfactant concentration falls, and its production can be inhibited by soluble proteins, lipids, or other substances.
Increased alveolar surface tension due to surfactant deficiency is thought to result in negative pressure surrounding the pulmonary capillaries, which promotes fluid filtration. Therefore, if pericapillary liquid pressure in the interstitium approximates Pliquid, surfactant deficiency will facilitate the formation of pulmonary edema.
▪ ISCHEMIA-REPERFUSION INJURY
Microvascular injury, manifested as an increase in microvascular permeability to fluid and protein, occurs in tissues subjected to periods of ischemia followed by reperfusion. The factors that promote, limit, and prevent ischemia-reperfusion microvascular injury to the lung are multiple and complex. The knowledge of how lung injury affects the microvascular barrier has been
considerably extended in the last 10 years. Several important discoveries, such as the reversal effects of cyclic adenosine 3′, 5′-monophosphate (cAMP) and the protective effect of nitric oxide, may eventually influence the clinical therapy.
considerably extended in the last 10 years. Several important discoveries, such as the reversal effects of cyclic adenosine 3′, 5′-monophosphate (cAMP) and the protective effect of nitric oxide, may eventually influence the clinical therapy.
What Are the Clinical Implications of Perioperative Pulmonary Edema?
▪ PRESSURE/HYDROSTATIC PULMONARY EDEMA
Two pathophysiologic and radiologic phases are recognized in the development of pressure edema: interstitial edema and alveolar flooding or edema. These phases are virtually identical for left-sided heart failure and fluid overload, and are the two most frequently observed causes of pressure edema in operating room, intensive care, and emergency patients. The intensity and duration of both phases are clearly related to the degree of increased pressure, which is determined by the hydrostatic-oncotic pressure ratio.
Interstitial edema occurs when mean transmural arterial pressure increases 15 to 25 mm Hg. This results in early loss of definition of subsegmental and segmental vessels, mild enlargement of the peribronchovascular spaces, appearance of Kerley lines, and subpleural effusions.18 If the quantity of extravascular fluid continues to increase, the edema will migrate centrally, with progressive blurring of vessels, first at the lobar level and later at the level of the hilum. At this point, lung radiolucency decreases markedly, making identification of the small peripheral vessels difficult. Peribronchial cuffing becomes apparent, particularly in the perihilar areas.
When transmural pressure exceeds 25 mm Hg, fluid drainage from the extravascular compartment is at maximum capacity. The second phase (alveolar flooding) then commences, leading to a sudden extension of edema into the alveolar spaces, and thereby creating tiny nodular or acinar areas of increased opacity that mature into frank consolidations (see Fig. 12.2).
Pulmonary artery catheters are frequently used to assess hydrostatic pressure in intensive care patients. The pulmonary capillary wedge pressure has been shown to reflect left atrial pressure and correlates well with the radiologic features of congestive heart failure and pulmonary venous hypertension (see Table 12.1). However, in acute heart failure, a time lag is often observed between the increased pulmonary capillary wedge pressure and the radiologic manifestation of pulmonary edema due to the relatively slow movement of water through the widened capillary endothelial cell junctions.8 Similarly, as pulmonary edema resolves, the radiologic findings will persist, with decreasing, or even normal, pulmonary capillary wedge pressure (see Fig. 12.3). In addition, pressure edema may show an asymmetric distribution on chest radiographs. The most frequent cause is an abnormal lung parenchyma, as seen in chronic obstructive pulmonary
disease (COPD). In cardiac failure, extensive lung emphysema of the apices (seen in heavy smokers) or marked destruction and fibrosis of the upper and middle portions of the lungs (seen in end-stage tuberculosis, sarcoidosis, or asbestosis) will result in pulmonary edema that predominates in the regions less affected by these disease processes.
disease (COPD). In cardiac failure, extensive lung emphysema of the apices (seen in heavy smokers) or marked destruction and fibrosis of the upper and middle portions of the lungs (seen in end-stage tuberculosis, sarcoidosis, or asbestosis) will result in pulmonary edema that predominates in the regions less affected by these disease processes.
TABLE 12.1 Correlation between Pulmonary Capillary Wedge Pressure and Radiologic Findings | ||||||||||
---|---|---|---|---|---|---|---|---|---|---|
|
Hemodynamic factors can also produce asymmetric distribution of pulmonary edema. Edema associated with severe mitral regurgitation has been shown to favor one particular lung, a result of flow impairment provoked by the reflux stream directed toward a particular upper pulmonary vein.19 Such asymmetric distribution occurs in 9% of adults and 22% of children with grade 3 or 4 mitral regurgitation.20
Finally, the position of the patient also influences intravascular and extravascular fluid distribution. In supine patients, axial computed tomography scan usually demonstrates an anteroposterior gradient, whereas more asymmetric distribution of edema secondary to prolonged surgery or immobilization is frequently observed in the lung fields of recumbent patients. This distribution is typically seen in congestive heart failure but is also observed in overhydration.
Unusual Forms of Hydrostatic Pulmonary Edema
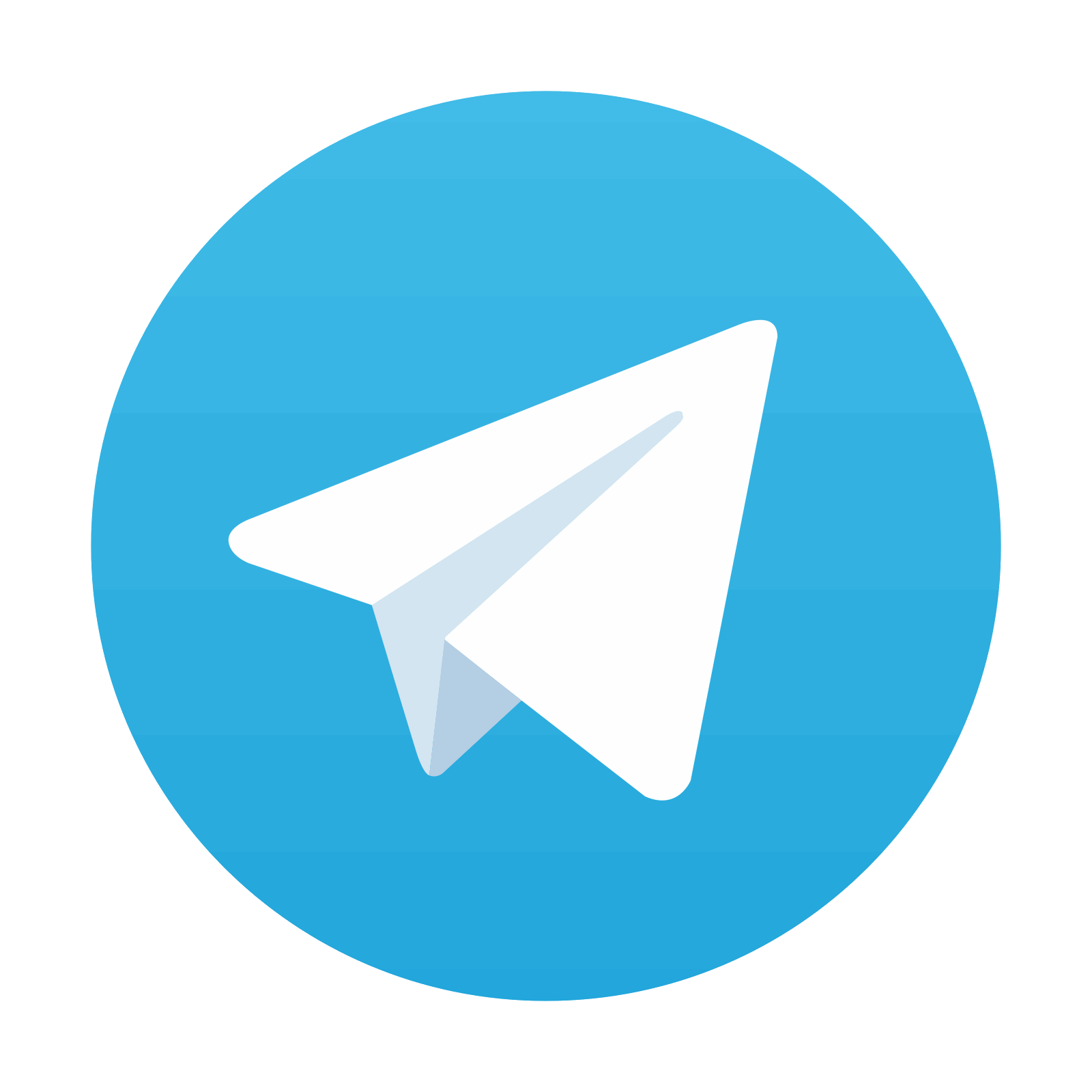
Stay updated, free articles. Join our Telegram channel

Full access? Get Clinical Tree
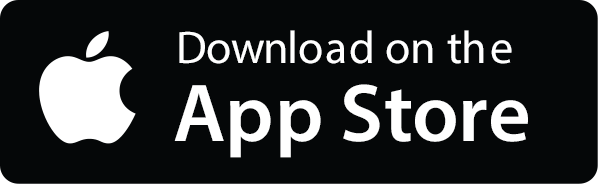
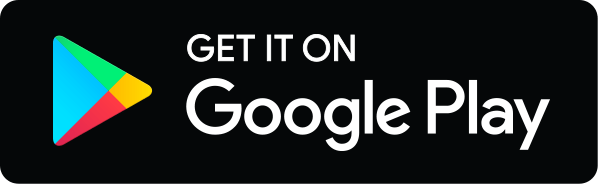
