Principles of Antimicrobial Therapy
BACKGROUND
In the past several decades, advances in the development of antimicrobial agents have supplied the physician with dozens of drugs effective against bacterial, fungal, and viral pathogens. Unfortunately, these advances have been paralleled by increasing antibiotic resistance and the emergence of new pathogens. More than half of Staphylococcus aureus bloodstream infections in the United States now derive from methicillin-resistant strains, and hospitalizations due to vancomycin-resistant Enterococcus (VRE) infections doubled between 2003 and 2006.1,2 In recent years, infections due to extended-spectrum beta-lactamase (ESBL)-producing gram-negative organisms and Clostridium difficile have increased in frequency, while carbapenemase-producing pathogens have emerged and spread worldwide.3–5 The incidence of sepsis also appears to be increasing,6–9 driven by an aging population and an intensified use of immunosuppressive medications. Overall, the prevalence and complexity of infectious diseases are greater than ever. The appropriate, rational use of antibiotics in the emergency department and intensive care unit requires a thorough understanding of principles of therapy and our current arsenal of antibiotics.
CHOOSING AN INITIAL EMPIRIC REGIMEN
Clinical Factors to Consider
Presumptive Diagnosis
Because microbiologic data generally take 24 to 72 hours to return, clinicians are usually forced to choose an empiric antibiotic regimen when encountering a patient with a likely infection. Making a presumptive infectious disease diagnosis is a critical step in deciding on an antimicrobial regimen, as many diagnoses tend to be associated with a predictable set of pathogens. The clinician should take into account the patient’s signs and symptoms, physical exam, and basic workup including labs, urinalysis, chest x-ray, and other imaging studies as appropriate. Suggested empiric regimens for common serious infectious syndromes are described in Table 33.1.
TABLE 33.1 Recommendations for Initial Empiric Therapy for Common Serious Infections
Organism Abbreviations: MSSA, methicillin-sensitive Staphylococcus aureus; MRSA, methicillin-resistant Staphylococcus aureus; CA-MRSA, community-acquired MRSA; ESBL, extended-spectrum beta-lactamase producer; VRE, vancomycin-resistant Enterococcus.
Comorbidities
The choice of antibiotics depends in large part on the presence of comorbidities, especially the degree of immunosuppression. A history of HIV, corticosteroid or immunomodulator therapy, chemotherapy, organ transplant, malignancy, or congenital immunodeficiency drastically increases the range of pathogens that could be causing disease. Infections in immunocompromised hosts are discussed in detail in a separate chapter.
Clinical Setting and Local Resistance Patterns
It is important to determine whether the patient is likely to have a community-acquired or hospital-/health care–associated infection, as the latter is associated with more resistant organisms. For example, methicillin-resistant S. aureus (MRSA) and Pseudomonas are leading causes of hospital-acquired pneumonia, while they are rare in community-acquired cases.10 For patients with a health care–associated infection, knowledge of local resistance patterns can be very helpful in choosing an appropriate regimen.
Recent Antibiotic Exposure
A history of the patient’s recent antibiotic exposure should be elicited. A recent study of critically ill patients with gram-negative sepsis showed that patients who received antibiotics within the past 90 days were more likely to have resistant organisms, receive inappropriate initial therapy, and die.11 In particular, recent broad-spectrum antibiotic exposure is a risk factor for MRSA and multidrug-resistant Pseudomonas, Acinetobacter, and Enterobacteriaceae.
Known History of Resistant Organism
A history of colonization (or prior infection) with resistant pathogens should also be noted. Patients colonized with MRSA are at higher risk for developing MRSA-invasive infection. In one study of patients in whom nares cultures were obtained on hospital admission, 19% of those colonized with MRSA developed MRSA infection in the following year, compared to 2% whose were not colonized.12 Similar results have been noted in patients colonized with VRE.13
Level of Illness
The acuity of the presentation dictates the breadth of coverage of the initial regimen, as well as the timing of therapy (discussed later in this chapter). Inappropriate initial antibiotic therapy (i.e., when the pathogen is later shown to be resistant in vitro) in patients with severe sepsis is associated with a fivefold increase in mortality risk.14 Therefore, when patients are severely ill, it is preferable to start with a broad-spectrum regimen; de-escalation can and should occur later as guided by microbiologic data. Conversely, a patient with a very mild presentation of an infection may not warrant broad-spectrum antibiotics at the onset.
Antibiotic Allergies
Taking an accurate allergy history prior to administration of antibiotics is necessary to avoid serious iatrogenic events. When assessing allergies, be sure to determine the severity of reaction and how long ago it occurred. For example, a history of anaphylaxis usually precludes reexposure under any circumstance, but patients with a remote history of mild rash or other vague symptoms can often be safely rechallenged. Penicillin allergies are common examples of this phenomenon, as fewer than 10% of patients who report penicillin allergies have a positive skin test.15 Avoid inappropriately labeling mild toxicities (e.g., gastrointestinal [GI] upset) as drug “allergies,” as this may prevent future providers from administering drugs that may be vitally important. Skin testing and desensitization are not typically practical in the acute setting, and so with a history of a potential allergy, the clinician must weigh the risks and benefits of administering that antibiotic. In most scenarios, however, a reasonable alternate choice is available.
Pharmacologic Factors to Consider
Bactericidal Versus Bacteriostatic Therapy
A common distinction is made based on the mechanism of action of antimicrobial agents. Agents that act on the cell wall tend to be bactericidal and cause cell death; beta-lactams are the classic example. Other bactericidal drugs include daptomycin (which causes cell membrane depolarization) and fluoroquinolones (which disrupt bacterial DNA). Drugs that act on protein synthesis, such as tetracyclines, macrolides, and clindamycin, tend to be bacteriostatic and inhibit growth of the organism without killing it. However, these distinctions are not absolute, and clinical outcomes do not necessarily parallel the mechanism of action. Furthermore, the relative bactericidal or static nature of an antibiotic depends on the organism and the minimum inhibitory concentration (MIC), defined as the lowest concentration of an antibiotic that inhibits growth of the organism. For example, vancomycin is considered to be a slowly bactericidal agent but is generally considered bacteriostatic against Enterococcus. As a general rule, bactericidal agents are preferred for serious infections such as endocarditis and meningitis.16
Mechanism of Killing
The two major types of “killing” exhibited by antibiotics are time-dependent killing, where efficacy depends on maximizing the time the antibiotic concentration is above the MIC, and concentration-dependent killing, where efficacy depends on achieving peak concentrations far above the MIC. Drugs that act via time-dependent killing include beta-lactams and vancomycin; those that act via concentration-dependent killing include aminoglycosides, fluoroquinolones, metronidazole, and daptomycin. The mechanism of killing has implications for dosing strategies. For time-dependent beta-lactams, there is increasing evidence that continuous or prolonged infusion strategies (to maximize time above the MIC) may lead to better microbiologic and possibly clinical outcomes in critically ill patients with resistant organisms.17–19 For concentration-dependent aminoglycosides, once-daily dosing strategies are in many circumstances as efficacious and less toxic than traditional q8h dosing.20
Sites of Penetration
Choosing an initial appropriate regimen requires knowledge of the distribution and penetration of antibiotics into different tissue compartments, as well as determination of the patient’s most likely site of infection. A particular antibiotic may match a pathogen under most circumstances, but if it does not act at the site of the infection, it will be clinically ineffective. Important examples of deficiencies in site penetration include the following:
- Central nervous system: First- and second-generation cephalosporins, macrolides, clindamycin, daptomycin, and aminoglycosides tend to have poor penetration and are suboptimal for treatment of meningitis.21 Third-generation cephalosporins and carbapenems are better choices.
- Lungs: Daptomycin is inactivated by surfactant, making it ineffective for pneumonia.22 Beta-lactams and fluoroquinolones are better choices.
- Urine: Moxifloxacin does not achieve adequate levels in the urine, making it ineffective for urinary tract infections (UTIs). Levofloxacin and ciprofloxacin are better choices among the fluoroquinolones.
Antibiotic Metabolism
The kidneys or the liver metabolize most antibiotics, and dysfunction in those organs may affect the dose or force the clinician to choose another drug class entirely. Dose adjustment for renal dysfunction in particular is frequently necessary; in these cases, creatinine should be routinely checked prior to antibiotic administration. Antibiotics whose dosing is dependent on renal function include vancomycin, aminoglycosides, and some beta-lactams; failure to account for this can lead to serious toxicities.
Combination Therapy
In critically ill patients, it is common to use two or more antibiotics in an empiric regimen to cover a broad range of pathogens (e.g., combining vancomycin and cefepime to cover gram-positive and gram-negative infections). Other than this, the reasons to consider combination therapy are as follows:
- To increase the likelihood of empiric therapy being active against the pathogen: In severely ill patients in whom microbiologic data are still pending and who are at risk for resistant organisms, administration of two antibiotics with similar spectrum of activity (typically gram-negative activity) increases the chance that at least one antibiotic will be active against the suspected organism. This is the rationale for the use of two gram-negative agents in nosocomial pneumonia and is often considered in patients in whom Pseudomonas is suspected (see “Empiric Pseudomonas Therapy” below).
- To achieve synergistic activity against an organism: Combinations of antibiotics can be synergistic in vitro, where the combined effect of the agents is greater than each alone. This scenario, however, is relatively uncommon in clinical practice and is mainly limited to endocarditis due to aminoglycoside-susceptible strains of Enterococcus and some streptococci (the synergistic agents in those cases are beta-lactams and aminoglycosides).23 Use of aminoglycosides for synergy in S. aureus infections and endocarditis was more common in the past, but is used less commonly now due to weak evidence of benefit and strong evidence of nephrotoxicity.24
- To prevent emergence of resistance: Certain organisms are prone to develop resistance when exposed to antimicrobial agents, and the simultaneous administration of several antimicrobials can decrease this risk. Examples include HIV and tuberculosis. This practice tends to be the exception rather than the norm in cases of routine bacterial pathogens, although it is often considered for Pseudomonas.
When combination therapy is used, antibiotics should be chosen from different classes (e.g., a beta-lactam plus a fluoroquinolone) in order to minimize overlapping toxicities as well as possible pharmacologic antagonism. In particular, double beta-lactams should be avoided whenever possible.
Empiric Pseudomonas Therapy
An important consideration in choosing an empiric antimicrobial regimen is the need to cover Pseudomonas, a nonfermenting gram-negative bacillus notorious for both its inherent resistance to most antibiotics as well as its propensity to develop resistance. Pseudomonas is responsible for many nosocomial infections, including pneumonia, catheter-related infections, UTIs, and postsurgical infections. It also commonly affects immunocompromised patients (commonly causing neutropenic fever), those with cystic fibrosis, and burn patients. For immunocompetent patients, invasive Pseudomonas bacteremia at the time of hospital admission is rare. Based on a review of 4,114 episodes of gram-negative rod (GNR) bacteremia on admission, empiric antipseudomonal treatment in patients without immunodeficiency is warranted for patients with two or more of the following predictors: age >90 years, antimicrobial therapy within the preceding 30 days, presence of a central venous catheter, or presence of a urinary device.26 In this study, the percentage of episodes of GNR bacteremia that were due to Pseudomonas was 2% with no risk factors, 8% with one risk factor, and 28% with two risk factors.
For serious infections due to suspected Pseudomonas, it is generally recommended to “double cover” empirically with two antibiotics from different classes until susceptibilities are available, and then narrow to one drug. The rationale (as discussed in the “Combination Therapy” section above) is to increase the chance that one of the agents will be active against the isolate; this approach has been associated with improved mortality in patients with severe sepsis and gram-negative bacteremia.27
The benefit of continuing double coverage after antibiotic susceptibilities are identified for Pseudomonas remains a long-standing point of controversy. Two meta-analyses published in 2004 reached conflicting results for the combination of beta-lactams and aminoglycosides in gram-negative and pseudomonal infections. One study showed no clinical benefit and increased nephrotoxicity; the other showed a mortality benefit in the subset of GNR bacteremia due to Pseudomonas.28,29 Despite this uncertainty, several experts do recommend continuing combination therapy for serious infections due to Pseudomonas, including bacteremia in neutropenic patients, endocarditis, meningitis, and possibly pneumonia. If double coverage is utilized, as either empiric or definitive therapy, the regimen should include a beta-lactam plus either a fluoroquinolone or an aminoglycoside. The antibiotics with activity against Pseudomonas are described in further detail later in this chapter.
Empiric MRSA Therapy
Choice of an appropriate empiric regimen is often driven by the need to cover MRSA, which is feared for both its virulence and its resistance to many antibiotics. Failure to initially include an agent with activity against MRSA, in those who turn out to have a MRSA infection, is associated with increased mortality.25 MRSA is typically categorized as community acquired or health-care associated; the two strains have different genetics and epidemiology, although the difference between them has begun to blur. Risk factors for MRSA include known colonization or prior disease with MRSA, indwelling lines or hardware, prior antibiotic use, residence in a long-term care facility, immunosuppression, injection drug use, hemodialysis, and prolonged hospital stay. Therapy directed at MRSA should be considered in patients with risk factors, as well as in patients presenting with severe illness. Specific drugs with MRSA activity are detailed later in this chapter.
Timing of Antimicrobial Therapy
In critically ill patients, timely administration of antibiotics is essential. A retrospective study of 2,731 patients with septic shock found that time to appropriate antibiotic therapy was the factor most strongly associated with survival; each hour of delay after the onset of hypotension until administration of effective antibiotic therapy increased mortality by 7.6%.30 Similar results were reported in a recent single-center prospective cohort study of patients with severe sepsis or septic shock, where a delay in starting antibiotics more than 1 hour from hospital triage, or from the moment the patient qualified for early goal-directed therapy, increased in-hospital mortality risk by more than 50%.31 Administration of appropriate antibiotic therapy is also a medical emergency in bacterial meningitis and neutropenic fever. Practitioners should make every effort to obtain diagnostic blood (+/− cerebrospinal fluid, sputum, and urine) cultures prior to initiating antibiotics, but not at the cost of significant delay in starting antibiotics for critically ill individuals. In contrast, for stable patients, especially those for whom prolonged antimicrobial therapy is likely, it is crucial to obtain adequate specimen cultures prior to antibiotics. This sometimes requires invasive diagnostic tests and biopsies. Classic examples include subacute bacterial endocarditis and osteomyelitis, where symptoms have usually been present for weeks to months. In these situations, starting antibiotics before obtaining specimens for culture ultimately proves harmful, as the lack of a microbiologic diagnosis may lead to long treatment courses of excessively broad-spectrum antibiotics.
CONTINUING ANTIMICROBIAL THERAPY
Definitive Therapy and De-escalation
Once the organism causing an infection has been identified, the antibiotic regimen should be narrowed in order to reduce costs, toxicities, and emergence of resistance. Failure to de-escalate antibiotics is unfortunately common in clinical practice, and in certain scenarios, such as hospital-acquired pneumonia, it may be associated with worse outcomes.32 The decision to de-escalate for a specific infection may be complicated by other concurrent infections; in the absence of multiple infections, every effort should be made to narrow to the simplest regimen possible.
An important principle of therapy is the appropriate discontinuation of anti-MRSA therapy after 48 to 72 hours of negative cultures, assuming that adequate cultures were drawn prior to antibiotics. MRSA is a pathogen that grows easily on culture medium, so failure to recover MRSA strongly suggests an alternate organism. A similar rationale should be used to discontinue or narrow broad-spectrum gram-negative agents, such as the carbapenems, in the absence of positive cultures.
Interpretation of Antibiotic Susceptibility Results
After a pathogenic organism is identified on culture, it is usually subjected to antimicrobial susceptibility testing, whereby the ability of the organism to grow in the presence of various antibiotics in vitro is measured and interpreted using guidelines established by the Clinical and Laboratory Standards Institute. A report of “susceptible” indicates likely inhibition of the organism when the antimicrobial agent is used at the recommended dosage; a report of “resistant” indicates the opposite. A report of “intermediate” indicates that the MIC of the drug falls within attainable blood and tissue levels, but the response rates may be reduced compared to susceptible organisms (and may even be ineffective in sequestered body sites). MICs of different agents for an organism are not directly comparable; an antibiotic with an MIC of 1 is not necessarily superior to a different antibiotic with a reported MIC of 2. The susceptibility data are very useful but are subject to important limitations:
- Not all antibiotics to which an organism is susceptible are equally effective: Although an organism may be susceptible to multiple antibiotics, clinical evidence often points to a superior match. For example, methicillin-susceptible S. aureus (MSSA) is susceptible to both vancomycin and nafcillin; however, nafcillin (and cefazolin) has a much higher clinical success rate than vancomycin for serious infections.33,34 Only in the case of a severe beta-lactam allergy should vancomycin be used over nafcillin or cefazolin. Similarly, C. difficile is uniformly susceptible to intravenous or oral metronidazole and oral vancomycin; however, oral vancomycin is associated with superior cure rates, especially in severe infections.35
- Some organisms carry enzymes that mediate resistance in vivo to antibiotics that are active in vitro: For example, ESBLs, which are enzymes that confer resistance to almost all beta-lactams except carbapenems, may not be apparent on routine testing. One prospective study of patients with bacteremia from ESBL Klebsiella pneumoniae showed that use of carbapenems was associated with significantly lower short-term mortality than was use of other antibiotics that were active in vitro.36
- Inducible chromosomal resistance may not be detected on initial susceptibility testing: Some Enterobacteriaceae produce an inducible chromosomal beta-lactamase that can be expressed during antimicrobial therapy. Although initial tests may report susceptibility, clinical failure and development of resistance to beta-lactams occasionally occur with agents other than fourth-generation cephalosporins and carbapenems.37 This is a risk with the so-called “SPICE A” group of organisms: Serratia, Pseudomonas, Indole-positive Proteus, Citrobacter, Enterobacter, and Acinetobacter.
Duration of Therapy
The optimal duration of therapy for many types of infections is based on expert opinion rather than well-designed studies. There are, however several important publications that have defined optimal courses of therapy, usually with an emphasis on shorter courses. One frequently cited study compared 8 versus 15 days of therapy for ventilator-associated pneumonia (VAP) diagnosed by bronchoalveolar lavage and found similar outcomes between the courses.38 Another meta-analysis of studies examining antibiotic courses for community-acquired pneumonia reported successful treatment of mild to moderate cases within 7 days or fewer.39 The appropriate duration of therapy will ultimately be determined by the clinical response to therapy, pathogen-specific factors, and host factors. For example, the study that examined the 8-day course of antibiotics for VAP excluded immunocompromised patients, and the optimal duration of therapy for this population remains unknown. Furthermore, patients in that study with Pseudomonas as the pathogen causing VAP had increased recurrence rates with the shorter course; this has led to recommendations that longer courses of therapy be used in this scenario.40 Although many studies have emphasized short durations of therapy, certain deep-seated infections (such as endocarditis and osteomyelitis) clearly require prolonged courses of therapy to minimize relapse and treatment failure.
Clinical improvement is usually monitored by resolution of symptoms and normalization of laboratory values. Radiologic improvement can sometimes be misleading and lag behind clinical improvement. The importance of microbiologic cure depends on the type of infection; it is a crucial factor in bacteremia where failure to clear blood cultures generally indicates inadequate antimicrobial therapy and/or source control.
Route of Administration
For critically ill patients, an initial intravenous route of administration is generally preferable. However, several antibiotic classes have excellent bioavailability, making transition to oral therapy easy. These include fluoroquinolones, trimethoprim–sulfamethoxazole, metronidazole, clindamycin, azithromycin, and linezolid. Beta-lactams tend to have poor bioavailability, making the oral route for this class inappropriate for most serious or deep-seated infections. Even for those drugs with good bioavailability, an oral route is generally considered to be less effective than intravenous therapy for certain serious infections such as endocarditis, meningitis, and S. aureus bacteremia.
OVERVIEW OF MAJOR ANTIBIOTIC CLASSES
An overview of the mechanism, spectrum, common indications, and side effects of the major antibiotic classes is presented in Tables 33.2 to 33.5.
TABLE 33.2 Major Antibiotic Classes: Beta-Lactams
Organism Abbreviations: MSSA, methicillin-sensitive Staphylococcus aureus; MRSA, methicillin-resistant Staphylococcus aureus; CA-MRSA, community-acquired MRSA; ESBL, extended-spectrum beta-lactamase producer; VRE, vancomycin-resistant Enterococcus.
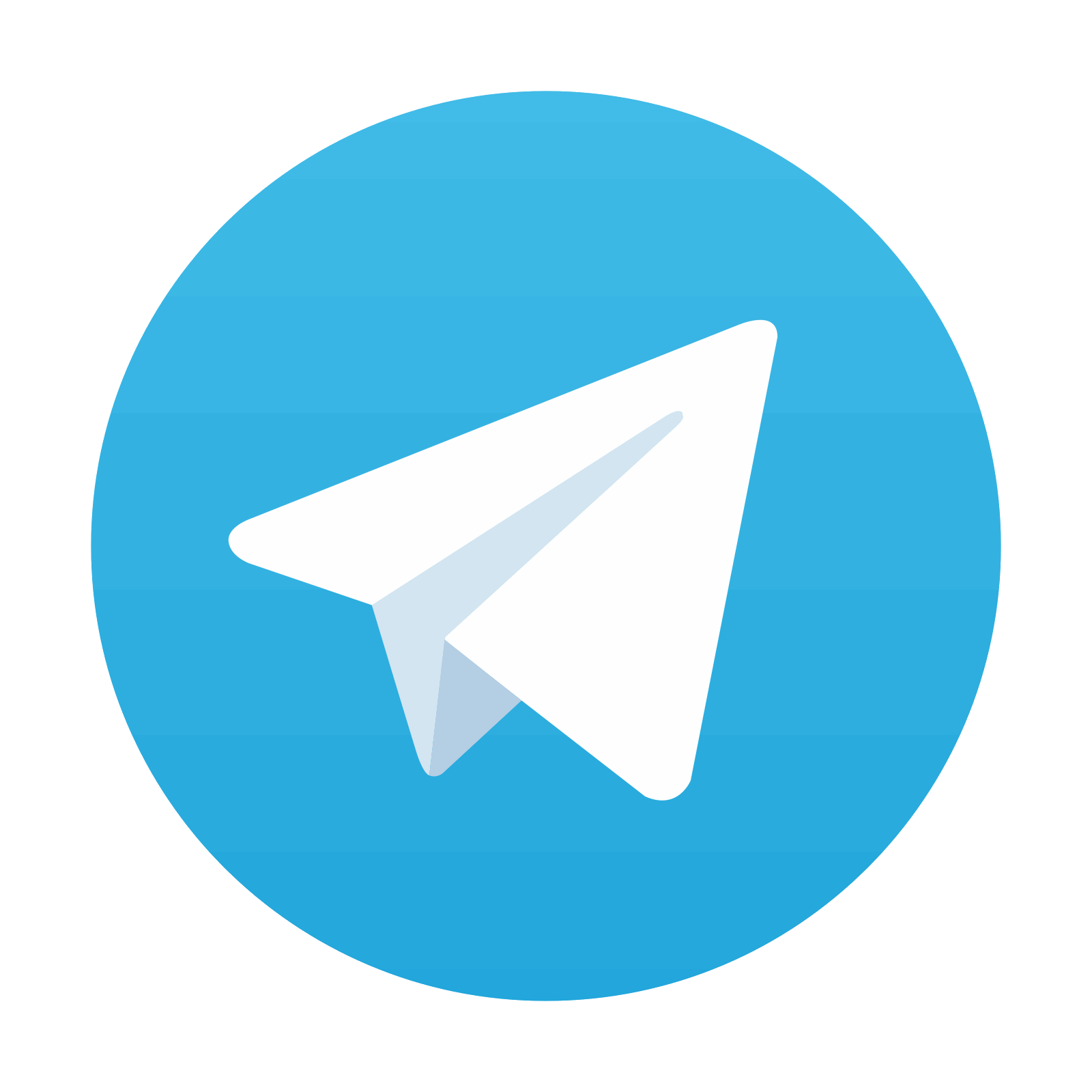
Stay updated, free articles. Join our Telegram channel

Full access? Get Clinical Tree
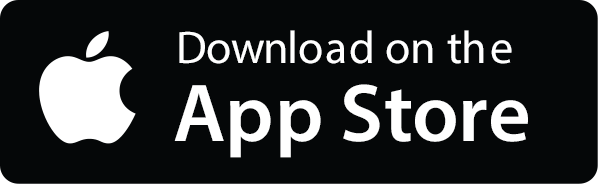
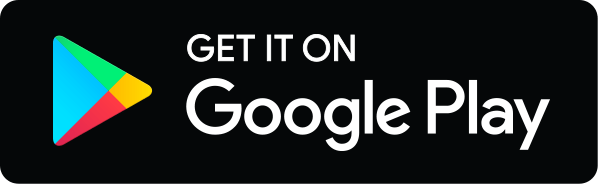