Extracorporeal Membrane Oxygenation
BACKGROUND
Extracorporeal membrane oxygenation (ECMO) is a term often used broadly to describe an extracorporeal circuit that provides short-term support of respiratory or cardiac function. In this chapter, we use the acronym ECMO in this expansive way, although ECMO is most accurately used to describe an extracorporeal circuit in which the primary goal is to provide oxygenation. Extracorporeal carbon dioxide removal (ECCO2R, pronounced ee-kor) is the more appropriate terminology when the primary function of the circuit is correction of hypercapnia. Extracorporeal cardiopulmonary resuscitation (ECPR) is used to describe the initiation of ECMO for resuscitation during cardiac arrest when conventional resuscitative efforts have failed.
The appropriate use of ECMO in the emergency department or intensive care unit requires an understanding of the principles behind extracorporeal support and an ability to identify circumstances in which its use would provide sufficient benefit to justify its associated risk. While ECMO is used in the neonatal, pediatric, and adult populations, this chapter only focuses on the use of ECMO in the adult population.
BRIEF HISTORY
In 1971, Dr. J.D. Hill documented the first successful case of ECMO for severe, posttraumatic hypoxemic respiratory failure.1 A subsequent multicenter, randomized, controlled trial published in 1979 evaluated conventional mechanical ventilation with and without venoarterial ECMO support for severe hypoxemic respiratory failure.2 This study failed to demonstrate a survival benefit from ECMO (9.5% in the ECMO group vs. 8.3% in the control group). Despite these findings, and inspired by the success of others in using ECCO2R to minimize respiratory rates and airway pressures in patients with severe hypoxemic respiratory failure,3–6 a subsequent randomized trial of ECCO2R was conducted in 1994, but again failed to demonstrate survival benefit (33% in the ECCO2R group, 42% in the control group).7 Both trials, however, had significant limitations, especially with regard to extracorporeal technology and mechanical ventilation practices, making their relevance in the era of modern-day ECMO and ventilation strategies questionable at best.
Enthusiasm for ECMO in the adult population was initially tempered by the lack of survival benefit in these early studies, but multiple subsequent observational reports, particularly during the influenza A (H1N1) pandemic in 2009, demonstrated high rates of survival with ECMO for the acute respiratory distress syndrome (ARDS).8–11 In an attempt to investigate the effect of ECMO on ARDS in the era of more advanced technology, the Conventional Ventilation or ECMO for Severe Adult Respiratory Failure (CESAR) trial was performed.12 One hundred eighty subjects with severe but potentially reversible respiratory failure were randomized to conventional mechanical ventilation or transfer to a single ECMO center for consideration of ECMO. Death or severe disability at 6 months occurred in 37% of the subjects in the ECMO-referred group, compared with 53% in the control group (relative risk 0.69). However, the lack of a standardized ventilation strategy in the control group; the differences in adherence to a low-volume, low-pressure strategy between the groups; and the fact that only 76% of patients referred for ECMO actually received ECMO limit the conclusions that can be drawn from this study. Nonetheless, the results of the CESAR study suggest there may be a benefit in referring patients with severe ARDS to a center capable of conducting ECMO as part of a larger management protocol.
INDICATIONS AND TECHNIQUE
ECMO may be initiated as salvage therapy in patients with life-threatening cardiac or respiratory failure that is refractory to conventional therapy. Regardless of the specific indication, it is important to note that extracorporeal support does not treat a patient’s underlying disease. Rather, it provides supportive care while the cause of cardiac or respiratory failure is addressed. In patients with end-stage respiratory failure, ECMO may be used, where appropriate, as a bridge to lung transplantation. In patients with end-stage cardiac failure, ECMO may be used as a bridge to either heart transplantation or a ventricular assist device (or total artificial heart), which itself may be used as destination therapy or as a bridge to transplantation.
If life-threatening respiratory or cardiac failure persists despite conventional management therapies, emergency medicine physicians should consider prompt ECMO consultation in appropriate patients. In centers where there is limited ECMO experience, early referral to a regional ECMO center is advised.
Respiratory Failure
ECMO and ECCO2R may be considered in cases of severe hypoxemic or hypercapnic respiratory failure. Etiologies of respiratory failure for which these modalities have been initiated include, but are not limited to, ARDS, refractory status asthmaticus, acute pulmonary embolism, pulmonary hypertensive crisis, end-stage respiratory failure pretransplant, and primary graft dysfunction in the posttransplant period. Uncommonly, ECMO has been instituted in cases of severe air leak syndromes.13,14
There is no universally accepted set of criteria for initiating ECMO in severe hypoxemic respiratory failure. However, reasonable proposed criteria include a partial pressure of oxygen to fraction of inspired oxygen ratio (PaO2/FIO2) <80 despite the use of high levels of positive end-expiratory pressure (PEEP) for several hours, uncompensated hypercapnia with acidemia (pH < 7.15), or excessively high end-inspiratory plateau airway pressures despite standard-of-care lung protective ventilation strategies.15
ECMO is considered a rescue strategy and should not be initiated, either in the medical intensive care unit or from the emergency department (ED), until conventional strategies to optimize oxygenation and ventilation have proven inadequate.
Cardiac Failure
ECMO can be used to provide hemodynamic support in cardiogenic shock that is refractory to aggressive inotropic support. Although it is an application that requires continued study, ECMO may also be employed in cases of respiratory failure with concomitant hemodynamic instability, including sepsis-induced cardiomyopathy or severe septic shock.16
With advances in extracorporeal technology that allow for rapid cannulation, there has been increasing interest in the use of ECPR for in-hospital cardiac arrest, typically in cases where return of spontaneous circulation has not occurred within 10 minutes or more of maximal resuscitative efforts.17,18 This application requires availability of an on-site ECMO team, which in some institutions may include emergency medicine physicians. Recent data show that patients with in-hospital cardiac arrest, who received conventional cardiopulmonary resuscitation (CPR) that was escalated to ECPR or to ECPR combined with intraarrest percutaneous coronary intervention, had better survival than patients receiving conventional CPR alone.19–21
Contraindications
While ECMO may benefit select critically ill patients, there are situations in which extracorporeal support has an unacceptable risk to benefit ratio. Relative contraindications include limitations in vascular access that would preclude cannulation and comorbid conditions or concomitant organ dysfunction for which aggressive management would not provide meaningful benefit (e.g., advanced metastatic cancer or devastating neurologic injury). Finally, since the use of systemic anticoagulation is strongly recommended to maintain the integrity of the ECMO circuit, a contraindication to the use anticoagulation is also a relative contraindication for ECMO.
Technique
The initiation and management of ECMO requires a trained, multidisciplinary team, typically consisting of surgeons, intensivists, ECMO specialists, and nurses. ECMO circuits most often require insertion of a drainage cannula into a central vein. Deoxygenated blood is withdrawn by a pump and passes through an oxygenator, where gas exchange occurs. The blood passes along one side of a semipermeable membrane, and “sweep gas,” typically 100% oxygen, is delivered to the other side. Oxygen is taken up by the blood, and carbon dioxide is removed. The oxygenated blood may be heated or cooled as necessary and is returned to the patient via a reinfusion cannula. The reinfusion cannula can be inserted into either a central vein (venovenous ECMO) or artery (venoarterial ECMO) (Fig. 13.1).15
FIGURE 13.1 A: Venovenous extracorporeal cannulation; deoxygenated blood is drained from the femoral vein with oxygenated blood being returned to the right atrium. B: Venoarterial cannulation; deoxygenated blood is drained from the femoral vein and returned to the femoral artery where oxygenated blood flows in a retrograde direction up along the aorta. When some residual cardiac function remains, oxygenated ECLS blood mixes with deoxygenated blood ejected from the left ventricle. ECLS, extracorporeal life support. Adapted from Gaffney AM, Wildhirt SM, Griffin MJ, et al. Extracorporeal life support. BMJ. 2010;341:982–986. Copyright ©2010, British Medical Journal, with permission from BMJ publishing group.
Venovenous ECMO is indicated in patients with hypoxemic or hypercapnic respiratory failure but preserved cardiac function. The configuration may consist of either single-site or multisite cannulation. In single-site cannulation, a dual-lumen cannula is inserted into the internal jugular vein and advanced through the superior vena cava and right atrium into the inferior vena cava under transthoracic echocardiographic or fluoroscopic guidance.22,23 Drainage ports of one lumen are positioned in the superior and inferior vena cavae, and the port of the second lumen is positioned such that the reinfusion jet is directed across the tricuspid valve. Multisite (typically dual-site) venovenous cannulation is commonly performed using the internal jugular and femoral veins and requires less sophisticated radiographic guidance and technical expertise radiographic guidance than single-site cannulation. While more expedient in emergent situations, this cannulation strategy is more prone to recirculation, which occurs when reinfused oxygenated blood is drawn back into the extracorporeal circuit by the venous drainage cannula instead of flowing across the tricuspid valve and contributing to systemic oxygenation. Single-site cannulation, when properly positioned, is less prone to recirculation. In venovenous ECMO, blood pressure and heart rate are managed in standard fashion, as they would be for any patient not receiving extracorporeal support. Because oxygenation in ECMO is dependent on the amount of blood passing through the oxygenator, some cases of severe hypoxemia may require a second venous drainage cannula to maximize the rate of blood flow through the circuit (veno–venovenous ECMO) (see Fig. 13.1).
Venoarterial ECMO is indicated in cases of severe cardiac failure refractory to conventional therapy, combined respiratory and cardiac failure, and ECPR. Femoral venous and arterial cannulation is the most common configuration and is the approach of choice in unstable patients and in the ED, as it can be performed rapidly and with minimal interference of ongoing resuscitative efforts in cases of ECPR. Vascular access is typically obtained using the Seldinger technique, though at times surgical cutdown or a hybrid technique may be required. Intrathoracic cannulation may be performed intraoperatively, but is less likely to be used in the ED. Mean arterial pressure and systemic perfusion in venoarterial ECMO are determined by a combination of the blood flow rate through the circuit, the native cardiac output, and systemic vascular resistance. Because blood reinfused from the femoral arterial cannula flows in a retrograde direction in the descending aorta, ECMO blood flow can be impeded by the patient’s native cardiac output and may not necessarily reach the aortic arch, great vessels, or coronary arteries. Furthermore, oxygen saturation measured in the lower limbs may not accurately reflect the amount of oxygenated blood being delivered to the brain and heart, and because of this, oxygen is preferably monitored from a site on the upper body, such as the right upper extremity. When there is concern for adequate upper body perfusion, a second reinfusion cannula can be inserted into the internal jugular vein (venoarterial venous ECMO), with that portion of oxygenated blood being pumped into the ascending aorta via the patient’s native cardiac function. Additional venoarterial configurations include internal jugular venous drainage and subclavian arterial reinfusion, though the latter requires placement in the operating room.24
MANAGEMENT GUIDELINES
Circuit-Related Factors
A trained ECMO team manages the extracorporeal circuit. A brief description of these management principles is provided here in order to highlight the possible complications and concerns associated with extracorporeal circuitry.
Anticoagulation
In preparation for vascular cannulation, patients are given a bolus of unfractionated heparin, followed by a continuous heparin infusion. To assess the adequacy of anticoagulation, activated clotting time (ACT) or activated partial thromboplastin time (aPTT) is followed; other evaluations, such as anti–factor Xa assays and thromboelastography, have also been employed.25–27 When heparin is contraindicated, direct thrombin inhibitors may be used.28,29 Platelet counts typically decrease with ECMO and should also be monitored. Although platelet transfusion thresholds vary by center, 20,000 platelets/cm3 is a reasonable trigger for transfusion in the absence of bleeding. The ECMO circuit should be periodically inspected for thrombi, whose presence may indicate a need to increase anticoagulation or change circuit components.
Gas Exchange
The sweep gas is the source of oxygen for the circuit and also provides a gradient for carbon dioxide diffusion out of the blood. The fraction of oxygen delivered in the sweep gas (FDO2) is adjusted by a blender, but is typically maintained at 1.0. Goal arterial oxygen saturation is generally ≥88%, although this may vary by patient and institution. The amount of oxygen supplied by ECMO is determined primarily by the blood flow rate through the circuit, since oxygen transfer across the membrane is highly efficient. A higher blood flow rate means that a greater proportion of the patient’s cardiac output passes through the oxygenator and, therefore, makes a greater contribution to systemic oxygenation. It is important to note that the proportion of cardiac output that passes through the circuit depends not only on the circuit blood flow rate but also on the total cardiac output of the patient. Any increase in a patient’s cardiac output, for a given extracorporeal blood flow rate, will translate to a lower proportion of blood flowing through the circuit and a decrease in ECMO’s contribution to systemic oxygenation. Oxygen delivery is also affected by hemoglobin concentration; however, there is no universally accepted transfusion threshold for packed red blood cells. Current recommendations for transfusion during ECMO are the same as for all critically ill patients.
Carbon dioxide removal is primarily dependent on the sweep gas flow rate, as carbon dioxide diffusion across the oxygenator is extremely efficient. The sweep gas flow rate should be adjusted as needed to achieve appropriate pH and PaCO2 goals.
Blood Flow
Blood flow through the ECMO circuit is determined by the revolutions per minute (RPMs) of the pump (although newer pumps allow the blood flow rate to be set with variability in RPMs), the sizes of the drainage and reinfusion cannulae, the intravascular volume of the patient, and the resistance to reinfusion flow. Increasing the RPMs on the pump will usually increase blood flow, but close attention must be paid to the pressure within the venous drainage cannula. As the RPMs are increased, pressure will become more negative in the venous limb; excessively negative pressures (typically more negative than minus 50 to 100 mm Hg) may cause trauma to red blood cells. High positive pressures (typically >300 to 400 mm Hg) in the reinfusion cannula are also potentially problematic. Adequacy of blood flow is monitored by levels of oxygen saturation and markers of end-organ perfusion.
Patient-Related Factors
Sedation, Analgesia, and Neuromuscular Blockade
During cannulation, sedation, analgesia, and neuromuscular blockade may be required to provide comfort and minimize patient movement. Following stabilization on ECMO, sedation and analgesia should be titrated as they would in other critically ill patients. Select patients, once in the ICU, will be able to tolerate decreased sedation. In such patients, sedation is minimized in favor of allowing the patient to be awake and potentially participate in physical rehabilitation.
Occasionally, ongoing neuromuscular blockade may be required in order to optimize cardiac or respiratory function. When neuromuscular blocking agents are administered, the patient must be adequately sedated, and the degree of neuromuscular blockade should be monitored with “train of four” sequences by a peripheral nerve stimulator.
Adequate dosing of sedatives can be challenging due to pharmacokinetic alterations in the ECMO circuit. The circuit provides an additional volume of distribution and may adsorb certain, especially lipophilic, medications onto the surfaces of the tubing and oxygenator, reducing their bioavailability. This is an area of active investigation, and the behavior of many medications in the setting of ECMO is not known. Frequent reassessment is recommended to ensure that the desired effect of a given medication is being achieved.
Hemodynamics
In patients receiving venovenous ECMO, manifestations of hemodynamic instability, including hypotension, tachycardia, dysrhythmias, and inadequate perfusion, are managed as they would be in any other critically ill patient. In venoarterial ECMO, blood flow rate may be adjusted to increase mean arterial pressure and enhance perfusion.
Ventilator Management
Ventilator management practices vary based on the underlying condition necessitating the use of ECMO. In patients with ARDS, once stabilized on ECMO, the goal of mechanical ventilation is to minimize the deleterious effects of positive pressure ventilation, using a low-volume, low-pressure strategy. The ARDS Clinical Trials Network ARMA trial demonstrated mortality benefit when using goal tidal volume and plateau airway pressure ≤6 mL/kg predicted body weight and 30 cm of water, respectively.30 Lower tidal volumes and plateau airway pressures than those used in the ARMA trial, a target often referred to as “lung rest,” may provide additional benefit for patients receiving ECMO for ARDS, although this remains an area of continued study.31–33 FIO2 should also be minimized as tolerated, though it is prudent to continue moderate to high levels of PEEP to maintain alveolar patency and minimize atelectasis. In patients with severe air leak syndromes, extracorporeal support could allow complete discontinuation of the ventilator to allow sealing of the leak.13 In patients requiring venoarterial ECMO for the treatment of cardiac failure, lung rest setting should be avoided to prevent the development of atelectasis.
Volume Status and Electrolyte Management
Patients are often volume overloaded at the time of ECMO initiation as a result of resuscitative efforts related to their critical illness. In patients with ARDS, additional volume infusions should be minimized, and aggressive diuresis should be instituted, as tolerated, to minimize extravascular lung water. If diuresis is limited by renal dysfunction, or if it alone does not achieve adequate euvolemia, ultrafiltration should be considered.
In patients without ARDS who are receiving venoarterial ECMO, optimal volume status should be determined clinically. Patients receiving ECPR and therapeutic hypothermia may experience cold diuresis; their volume status must be closely assessed in order to avoid hypovolemia. Electrolyte derangements may also occur following resuscitation or during diuresis and should be managed as in other critically ill patients.
Infection
In ECMO, as with any vascular access, precautions must be taken to minimize infection. Antibiotic prophylaxis is not specifically required, but patients should continue to receive any antibiotic therapy clinically indicated prior to ECMO initiation as well as standard monitoring for general infection. Cannula maintenance and dressing changes require strict aseptic techniques, and access points within the circuit should be kept to a minimum. It is important to note that the pharmacokinetics of antibiotics, much like sedative agents, may be altered with the use of the extracorporeal circuit, and antibiotic drug levels should be monitored to ensure adequate dosing.
Temperature Regulation
Temperature regulation can be achieved within the ECMO circuit. In most cases of venovenous and venoarterial ECMO, normothermia is the goal; warming of the blood within the oxygenator counteracts the effect of the extracorporeal blood cooling.
In cases of ECPR or other neurologic injury, therapeutic hypothermia may be induced following the initiation of ECMO and stabilization of the patient. Counterregulatory mechanisms, such as shivering, electrolyte derangements, insulin resistance, platelet dysfunction, and hemodynamic changes associated with cutaneous vasoconstriction, must be recognized and appropriately managed.
Procedures
Procedures should be minimized while a patient is receiving ECMO. If an intervention is necessary, anticoagulation may be temporarily discontinued; however, prolonged periods without anticoagulation should be avoided. Close attention must be paid to cannula positioning if the patient requires repositioning for a procedure. Ideally, patients should not be moved without the assistance or approval of the ECMO team.
Complications
Despite close monitoring, life-threatening complications do occur during all stages of ECMO, from initiation to weaning and decannulation. Therefore, ECMO should be instituted only when its benefits are believed to outweigh its risks. Bleeding complications may occur due to anticoagulation or as a result of vascular injury during cannulation. Additionally, cellular destruction and factor consumption by the circuit may lead to a clinical picture consistent with disseminated intravascular coagulation. ECMO cannulae are a nidus for thrombus formation, and inadequate anticoagulation may lead to thromboembolic complications. Air embolism has also been reported during cannulation. Adverse events related to the circuit and oxygenator, including oxygenator failure from thrombosis, have also been reported and require the vigilance of a trained ECMO specialist.34 Fortunately, complications have become considerably less frequent with recent improvements in ECMO technology.
CONCLUSION
The availability of extracorporeal support enables an ED to initiate early escalation of care in patients with severe respiratory or cardiac failure for whom the expectation of survival without such intervention is low. Emergency physicians have an opportunity to identify both patients who might benefit from ECMO support and those for whom it is inappropriate. At certain institutions, they may be responsible for initiating early consultation or regional referral to an ECMO center as well as providing essential ongoing management while the patient awaits transfer.
It should be emphasized that the initiation and management of ECMO is an involved process that requires a trained team and that inexperienced providers should never attempt extracorporeal cannulation or management without involvement from clinicians with ECMO expertise. That said, the ED’s role in providing extracorporeal support is likely to continue to expand as more centers include emergency physicians on ECMO teams to enable more efficient initiation of therapy.
LITERATURE TABLE
RCT, randomized controlled trial.
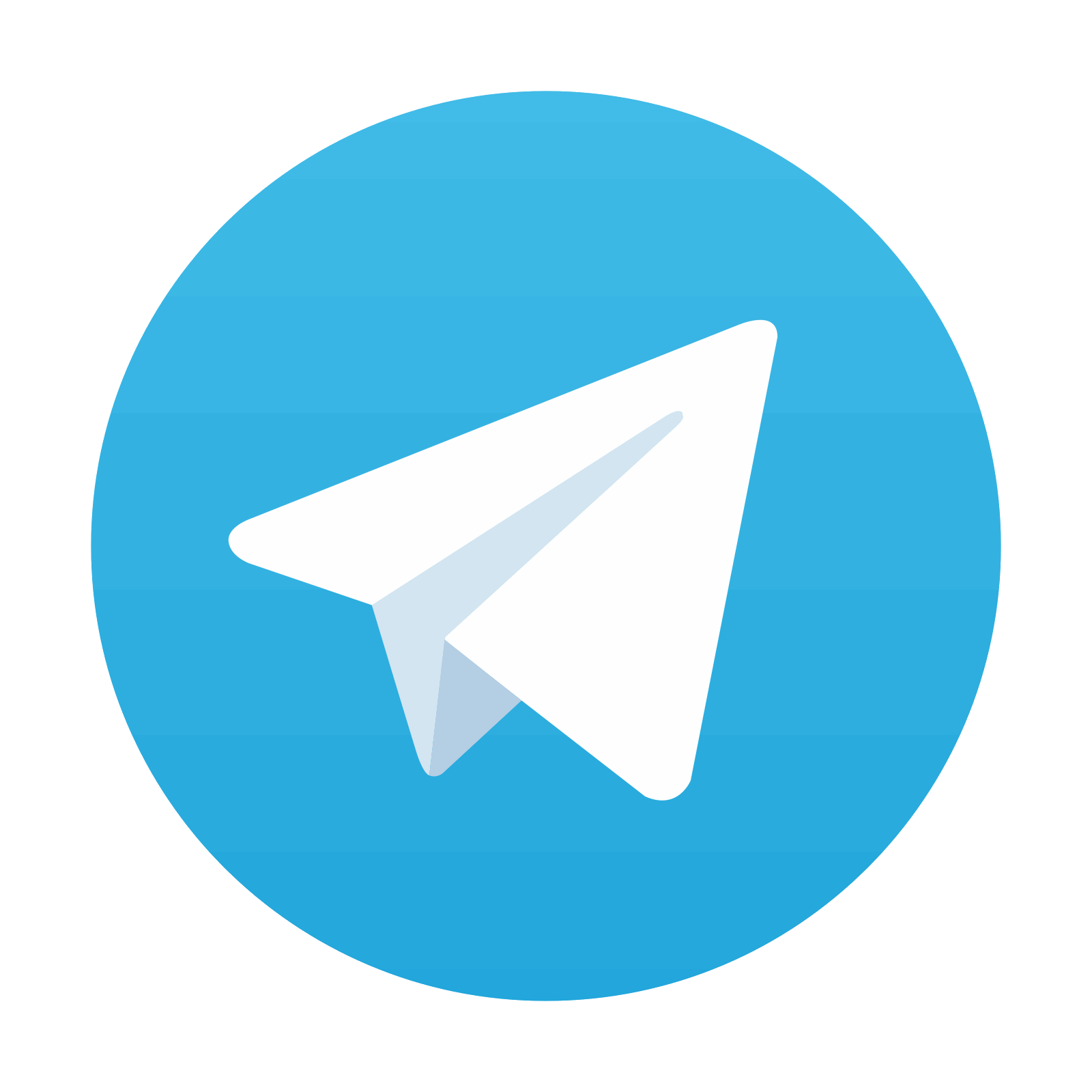
Stay updated, free articles. Join our Telegram channel

Full access? Get Clinical Tree
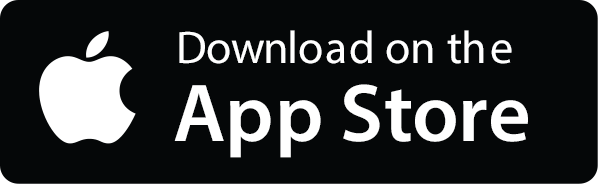
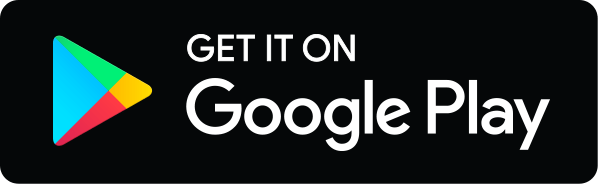