Sudarshan Ramachandran This chapter will describe: • basic anatomy and physiology of the endocrine and renal systems • the role of biochemical laboratory testing in the preoperative phase • a clinical approach to preoperative optimisation of endocrine and renal disease • therapeutic treatment of endocrine and renal disease and implications for perioperative care. The purpose of this chapter is to help those carrying out preoperative assessment to use laboratory investigations in a rational manner to augment their clinical skills when faced with possible endocrine pathology. It must be clearly stated that laboratory guidelines must be applied in conjunction with clinical judgement rather than as a substitute, which would result in a deskilled process. The role of preoperative investigations is to add diagnostic and prognostic data that could influence surgical outcome. These investigations could be either related or unrelated to the surgery planned, but may lead to alteration in clinical management. It is necessary to have some basic understanding of the endocrine system before being able to diagnose endocrine pathology or to assess current function in a preoperative setting. We therefore offer an overview of commonly encountered endocrine diseases, including the more prevalent clinical features, some of the relevant hormones and the general principles of testing. Endocrine hormones can be defined as chemical agents that are secreted by specialised cells and are carried in the circulation to act on non-adjacent target tissues. This is in contrast to paracrine and autocrine actions where the effects, after secretion, are on neighbouring and secreting cells respectively. The word ‘hormone’ is derived from the Greek hormao meaning ‘arouse’. Hormones are synthesised from amino acids (protein or peptide hormones), cholesterol (steroid hormones) or phospholipids (prostaglandins and related compounds). The steroid hormones include vitamin D as well as the adrenal and sex hormones secreted by the adrenal cortex and reproductive system respectively. Chemical side chains and/or changes in their tertiary structure determine the specificity of protein/peptide and steroid hormones. Many of the hormones circulate in the body bound to binding proteins (synthesised in the liver). Thus measuring total hormone concentrations (protein bound and unbound) may be a less accurate index of biological activity, as this is generally considered to correlate with the free hormone fraction. The actions of protein and peptide hormones are mediated by cell surface receptors, because they are less able to cross the lipid cell membrane. They mainly bind to the extracellular aspect of the receptor complex and this in turn leads to dissociation (most hormones) or activation (e.g. insulin, growth hormone, etc) of intracellular components. Steroid and thyroid hormones can cross the cell membrane and bind to intracellular or nuclear receptors, which in turn act as transcription factors, acting on the cell’s DNA. Greater mechanistic details of hormone actions are beyond the scope of this chapter and will not be described further. It is essential to understand how control is exerted via negative and positive feedback on endocrine systems. Some endocrine systems (thyroid, adrenal cortex, sex organs etc), which are controlled by the anterior pituitary, are subject to feedback from the hormones secreted by the gland. For example, secretion of TSH by the anterior pituitary is suppressed by high concentrations of thyroid hormones (T3 and T4) and increased when concentrations are low (see Figure 8.1, page 144). In contrast, most of the other endocrine systems are controlled by the concentration of the substances that they regulate, e.g. insulin production is controlled by glucose concentration, parathyroid hormone by calcium concentration, etc The commonly encountered human hormones are classified by system and presented in Table 8.1. Table 8.2: Some commonly encountered hormones are classified by system and described. Figure 8.1 The hypothalamic-pituitary-thyroid axis illustrating negative feedback at the pituitary and hypothalamic levels We briefly describe a few selected endocrine disorders that should be considered, appropriately investigated and managed prior to surgery. The levels of thyroid hormones (triiodothyronine – T3 and thyroxine – T4) are under the control of the hypothalamic-pituitary axis. Thyrotrophin releasing hormone (TRH) released by cells in the hypothalamus stimulates thyrotrophs in the anterior pituitary to produce thyroid stimulating hormone (TSH). TSH synthesis is inhibited by somatostatin, dopamine and various cytokines. TSH binds to specific receptors found on the surface of follicular cells in the thyroid and triggers a series of cascades leading to synthesis and release of thyroid hormones. TSH concentration is also subject to negative feedback from thyroid hormones via alterations in the sensitivity of the pituitary thyrotrophs to TRH. This sensitivity to TRH (via TRH receptor numbers) depends on the pituitary intracellular concentration of T3 (80% of which is derived from intra-pituitary conversion of T4). TRH and TSH release is also affected by other physiological conditions (e.g. body temperature) and non-thyroidal hormone levels. The common causes and clinical manifestations of hypothyroidism and hyperthyroidism are widespread and are described in Table 8.2. Table 8.2:
Chapter 8 Preoperative testing and perioperative management of endocrine and renal disease
David Kennedy
Munirul Haque
SUMMARY
INTRODUCTION
A practical understanding of the function and regulation of hormones
Common endocrine pathology and appropriate investigations
Thyroid disorders
Common causes and clinical manifestations of hypo-and hyperthyroidism.
Hypothyroidism | Hyperthyroidism |
Causes | |
Autoimmune thyroid disease (Hashimoto’s disease) | Graves’ disease (antibodies stimulating the TSH receptor) |
Primary atrophic hypothyroidism | Toxic multinodular goitre |
Post radioactive treatment | Thyroid adenoma |
Post surgery | Thyroiditis |
Thyroiditis | TSH secreting tumour |
Over treatment with carbimazole/propylthiouracil | Hyperemesis gravidarum |
Some forms of thyroid hormone resistance | |
Secondary to hypopituitarism | |
Hypothyroidism | Hyperthyroidism |
Common clinical features | |
Fatigue | Anxiety |
Cold intolerance | Irritability |
Weight gain | Heat intolerance |
Constipation | Fatigue |
Voice changes | Weight loss |
Dryness of skin | Increased appetite |
Loss of hair | Tremor |
Bradycardia | Tachycardia/atrial fibrillation |
Anaemia | Increased sweating |
Goitre |
Disorders of the adrenal cortex
The adrenal cortex produces glucocorticoids (mainly cortisol), mineralocorticoids (mainly aldosterone) and sex hormones. Cholesterol (mainly acquired from circulating low density lipoprotein – LDL) is the precursor of all these hormones, with each group synthesised by specific enzymes differentially expressed in distinct regions of the adrenal cortex. Some of the causes of adrenocortical pathology (Cushing’s syndrome, hyperadrenalism and hypoadrenalism) and associated clinical features are shown in Table 8.3. Although many of these conditions are rare, the consequences of not detecting some of these conditions (e.g. Addison’s disease) in a preoperative setting can be dire.
Glucocorticoids
Cortisol, the principal glucocorticoid, is essential to a wide variety of metabolic and cellular functions, by affecting DNA transcription. The metabolic effects, inhibiting muscle and adipose tissue glucose uptake, increased insulin resistance, increasing gluconeogenesis and increasing lipolysis, all result in hyperglycaemia and hyperlipidaemia. Other effects include increased cardiac output and vascular tone resulting in hypertension and maintaining the extracellular fluid volume.
Mineralocorticoids
Aldosterone, the principal mineralocorticoid, stimulates the active reabsorption of sodium from the glomerular filtrate reaching the distal convoluted tubule in the kidney. Synthesis and release of aldosterone is under the control of the renin-angiotensin system. A decrease in renal perfusion, increased sympathetic activity or a reduction in sodium delivery to the macula densa stimulates the release of renin. Renin stimulates a cascade, culminating in the synthesis of aldosterone by the adrenal cortex. Aldosterone binds to receptors in the renal distal tubule, causing salt and water absorption with a consequent increase in extracellular volume.
Table 8.3: Causes of adrenocortical disease and associated clinical features
Hypoadrenalism | Cushing’s syndrome | Hyperadrenalism |
Causes | ||
Sudden discontinuation of steroid therapy Addison’s disease (due to autoimmune destruction, infection, haemorrhage, metastases, drugs) Hypopituitarism | Iatrogenic (exogenous steroids) Pituitary adenoma Ectopic ACTH secretion Adrenal adenoma | Secondary causes (Physiological (pregnancy), hypovolaemia, CCF, cirrhosis, nephrotic syndrome, salt losing states, renal artery stenosis, diuretic treatment) Primary causes (adenoma, hyperplasia) |
Common clinical features | ||
Postural hypotension, collapse Weakness Weight loss Pigmentation Hypoglycaemia Abdominal pain | Weight gain Central obesity Moon face appearance Striae/acne/bruising/hirsuitism Hypertension Diabetes/impaired glucose tolerance Proximal myopathy Osteoporosis | Hypertension Hypokalaemia Alkalosis |
Disorders of the adrenal medulla: Phaeochromocytoma
Chromaffin cells in the adrenal medulla (which comprises 20% of the adrenal gland and part of the autonomic nervous system) secrete catecholamines. Adrenaline forms about 80% of adrenal catecholamine synthesis whereas noradrenaline is the principal catecholamine produced by the sympathetic nervous system. Dopamine is a precursor of both.
Catecholamines cause either vasoconstriction (via alpha receptors) or increases in heart rate, blood pressure, sweating and metabolic changes (via beta receptors). The released catecholamines have a very short half-life, being rapidly metabolised within minutes by the enzyme monoamine oxidase.
Phaeochromocytoma is a rare endocrine tumour, which may result in paroxysmal symptoms, due to a sudden release of catecholamines. Although rare, it is essential to diagnose the presence of phaeochromocytoma, especially preoperatively to prevent potential mortality. Palpation of the tumour and certain therapeutic agents used in anaesthesia can precipitate sudden hormone release, which may be fatal. Clinical features that lead to suspicion are presented in Table 8.4.
Table 8.4: Some of the physiological effects of catecholamines and clinical features ofphaeochromocytoma
Effects of catecholamines | Clinical features of phaeochromocytoma |
Cardiovascular (increase in heart rate, increases peripheral resistance) Visceral (smooth muscle relaxation) Endocrine (increased secretion of glucagon, aldosterone) Metabolic (increased glycogenolysis and lipolysis) Renal (Decrease in glomerular filtration and sodium excretion) | Anxiety Increased sweating Palpitations Hypertension (paroxysmal or sustained) Tremor Headaches Abdominal pain Hyperglycaemia |
Calcium regulation and parathyroid disorders
Calcium is essential for life and has an important role in many physiological functions. Thus hormone regulation of the calcium concentration in the blood is vital. The hormones act via regulation of extracellular calcium (0.1% of total calcium), but total body calcium levels also depend on the same process.
The hormones regulating calcium also control phosphate homeostasis and so both must be considered together. Phosphate has an important physiological role; including being a component of DNA, RNA, phospholipids, ATP, enzymes and second messengers (c-AMP).
In plasma, calcium is present in three forms: protein bound (mainly to albumin), complexed (with citrate and phosphate) and a free fraction. Only the free fraction is considered physiologically active and is the component maintained by homeostatic mechanisms.
Total calcium concentration is usually the measure reported by the laboratory, varying between 2.2 and 2.6mmol/L. However, measurement of the free ionised calcium fraction is often available from blood gas analysers, and is approximately half that of total calcium, i.e. 1.0–1.30mmol/L. Both the free and the bound fractions are in equilibrium. Thus, protein concentrations and alkalosis or acidosis, which affect protein binding of calcium, all affect the measured total calcium level.
Calcium and phosphate levels are dependent on absorption from the gastrointestinal tract, loss via urine and faeces and bone turnover. Hormones such as parathyroid hormone (PTH) and vitamin D play a crucial part in calcium homeostasis.
Serum calcium concentration exerts an influence on PTH secretion via negative feedback, i.e. high calcium concentrations reduce PTH release. The half-life of PTH in the serum is a few minutes. PTH acts on bone, resulting in immediate osteolysis, thus increasing serum calcium whilst a more delayed resorption process leads to increased calcium and phosphate concentrations in the extracellular fluid. PTH also acts on renal tubular cells, where it inhibits renal phosphate reabsorption and increases renal calcium reabsorption. PTH also stimulates conversion of 25 hydroxy- vitamin D to the active 1, 25 dihydroxy- vitamin D, which results in increased active calcium and phosphate absorption in the gut.
Vitamin D prohormones are obtained principally from the skin (stimulated by UVB exposure) but also from the diet. The inactive prohormones are metabolised to 25 hydroxylated forms in the liver and further converted in the kidney to the 1,25 dihydroxylated compounds, which are physiologically active. Calcium, phosphate, PTH and 1,25 dihydroxy- vitamin D concentrations regulate 1-a hydroxylase enzyme activity in the kidney, thus providing a feedback loop.
Vitamin D stimulates calcium, phosphate and magnesium absorption in the gut. Its action on bone is more complex and still unclear. The clinical features of both hyper- and hypocalcaemia which suggest a disruption of normal calcium homeostasis are described in Table 8.5 (page 150).
Pituitary gland disorders
The posterior pituitary comprises of axons and nerve terminals of neuronal cells situated in the hypothalamus, which secrete vasopressin and oxytocin. Anterior pituitary hormone secretion is controlled by hormones released into the hypophyseal portal veins by the hypothalamus. In response, the anterior pituitary lobe releases either TSH, ACTH, or gonadotrophins (LH and FSH), which in turn control hormone secretion by the thyroid, adrenal gland and sex organs.
Table 8.5: Causes and clinical features of hyper- and hypocalcaemia
Hypercalcaemia | Hypocalcaemia |
Causes | |
Primary hyperparathyroidism (adenoma, hyperplasia, carcinoma) Malignancies Excess vitamin D (overtreatment, sarcoidosis, tuberculosis) Familial hypercalcuric hypercalcaemia | Post thyroidectomy/parathyroidectomy Vitamin D deficiency Disordered vitamin D metabolism e.g. CRF Hypomagnesaemia Hypoparathyroidism/pseudohypoparathyroidism |
Clinical features | |
Fatigue/lethargy Depression/confusion Polydipsia/polyuria Musculoskeletal symptoms Constipation Renal calculi/nephrocalcinosis Cardiac arrhythmias/hypertension Corneal and vascular calcification | Weakness Muscular e.g. cramps/tetany Behavioural disturbances/stupor Convulsions Cataracts (chronic) |
The anterior pituitary also secretes GH and prolactin, the former stimulates IGF-1 production by GH receptor proteins, the latter stimulating lactation. This network of hormonal control via endocrine axes is shown in Figure 8.2.
Figure 8.2 Hormonal control via endocrine axes
Diseases affecting the anterior pituitary can be divided into deficiency and hypersecretory states. The deficiency states can be either generalised or axis specific. Some of the causes of generalised or pan-hypopituitarism include non-functional pituitary adenomas, cranio-pharyngiomas, metastases, inflammatory diseases (granulomatous lesions, lymphocytic hypophysitis), haemorrhage and infarction. In a significant number of cases, hypopituitarism is evident following clinical and biochemical investigations without an underlying cause being identified and these are classified as ‘idiopathic’ hypopituitarism. Axis-specific deficiency states can be detected due to any of the above causes including ‘idiopathic’ hypopituitarism. The common clinical symptoms and signs of hypopituitarism are mainly due to deficiency of hormones produced by the end organs under its control.
Vasopressin is synthesised in the hypothalamus and thus pituitary adenomas are unlikely to lead to deficiency causing diabetes insipidus. More likely causes of vasopressin deficiency are craniopharyngiomas, inflammatory conditions and non-pituitary tumours. Familial diabetes insipidus of unknown aetiology has been described as presenting soon after birth. Biochemical investigations depending on the symptoms of deficiency states can be broken down into frontline tests such as TSH and free thyroxine (FT4), testosterone/oestrogens and LH, FSH, cortisol, prolactin and IGF-1. Should the hormone values suggest an abnormality, dynamic function tests, in addition to radiological investigations, should be performed. These are best carried out in consultation with the endocrine team and clinical biochemistry staff, as strict protocols have to be adhered to and often the interpretation of the results is not clear-cut.
Hypersecretory states are usually due to benign pituitary adenomas (rarely malignant) synthesising and secreting specific hormones. Clinical features are due to excess levels of these specific hormones and once again the relevant investigations are similar to those previously described for the deficiency states.
Diabetes mellitus
Diabetes is a disease characterised by hyperglycaemia caused by a relative deficiency of insulin secretion and/or insulin resistance. Current views on aetiology are beyond the scope of this chapter. An understanding of the actions of insulin will also not be dealt with for the same reason. It is essential that diabetes is detected in undiagnosed patients and glycaemia is controlled optimally to reduce surgery-related complications. For the purposes of this chapter we will define diabetes as Type 1 and Type 2; other types of diabetes (e.g. associated with single gene disorders, syndromes with severe insulin resistance and secondary causes) are rare. The clinical features of Type 1 and Type 2 diabetes are presented in Table 8.6. The presenting features (together with age and ketosis) often but not always differentiate Type 1 from Type 2 diabetes.
Table 8.6: Clinical features of Type I and Type 2 diabetes.
Clinical features | Type 1 diabetes | Type 2 diabetes |
polyuria/thirst | ++ | + |
fatigue/weakness | ++ | + |
weight loss | ++ | – |
vulvovaginitis/pruritis | + | ++ |
peripheral neuropathy | + | ++ |
nocturnal enuresis | ++ | – |
asymptomatic | – | ++ |
Type 1 diabetes is due to an autoimmune-driven process, leading to infiltration and destruction of the endocrine pancreas: the islets of Langerhans; and is associated with a loss of insulin secretion. There appear to be both genetic and environmental elements to the pathogenesis. Ketoacidosis is due to insulin deficiency and can be seen in type 1 diabetes. Insulin, an anabolic hormone, acts to inhibit lipolysis, with ketone bodies being the end products of this pathway. Thus a deficiency of insulin (or omission of exogenous insulin) promotes an increase in ketone body production and resulting acidosis. Therefore, it is essential that insulin is not completely discontinued even when the patient is ‘nil by mouth’ immediately prior to surgery.
Type 2 diabetes is also associated with genetic and environmental causative factors, but in contrast is thought to be due to insulin resistance causing the cells to fail as they become unable to provide adequate compensation.
Preoperative testing
Patients with endocrine disease presenting for anaesthesia and surgery can represent a significant clinical challenge. This section considers how appropriate and well targeted laboratory testing, following a detailed clinical history, can help uncover endocrine disease in patients with undiagnosed endocrine conditions, as well as aiding the management of those with established disease. Here we cover a range of endocrine conditions, highlighting information of particular relevance to perioperative care.
Patients with previously undiagnosed endocrine disease
Patients with undiagnosed endocrine disease can often have vague non-specific symptoms that can delay a positive diagnosis considerably. It is therefore a significant challenge to identify individuals at the preoperative stage with previously unknown endocrine conditions without causing unacceptable levels of inappropriate testing and delay due to the inevitably high false positive rate.
Routine, or generic, testing of asymptomatic individuals prior to surgery, even for common endocrine diseases such as diabetes mellitus, has now been clearly identified as inappropriate. However, a detailed clinical history prior to surgery should help identify key indicators that suggest endocrine disease may be present.
Clinical screening for endocrine disease
The clinical history should be detailed enough to allow identification of individuals with an increased likelihood of having an undiagnosed endocrine disease, particularly if facing major surgery. Further follow-up testing should only be requested where clinical suspicion is high and the suspected endocrine disorder is likely to impact on either response to anaesthetic agents and/or recovery. Some of the clinical features leading to the possibility of endocrine pathology are presented in Table 8.7 (page 154). As hypopituitarism is diagnosed by examining the function of all endocrine systems, we present a simplified investigation pathway in Figure 8.3.
Table 8.7 Key factors in the clinical history that may be linked to endocrine disease
General symptoms | Possible disorder(s) | Suggested initial test(s) |
Recent weight loss but no loss of appetite | Hypothyroidism | TSH and FT4 |
Recent weight loss with severe fatigue | Hypoadrenalism (primary or secondary), diabetes mellitus | Cortisol (9am if possible), plasma glucose, TSH (Synacthen test if 9am cortisol low) |
Specific symptoms | ||
Thirst and polyuria (± nocturia) | Diabetes mellitus, diabetes insipidus, hyperparathyroidism, hypoadrenalism | Urea and electrolytes, serum osmolality, cortisol, calcium and albumin, TSH, random plasma glucose (GTT if RPG ≥ 8.0) |
Hypertension with proximal muscle weakness (especially if unexplained low potassium) | Cushing’s syndrome, Conn’s syndrome | Urea and electrolytes, random plasma glucose, BP |
Excessive sweating (generalised) | Hyperthyroidism, diabetes, acromegaly, phaeochromocytoma, carcinoid syndrome | TSH and random plasma glucose. If other endocrine disorders suspected seek specialist advice |
Hypotension | Hypoadrenalism (primary or secondary) | Urea and electrolytes, serum cortisol (9am if possible), ACTH |
Recurrent fungal infection | Diabetes mellitus | Random plasma glucose |
Palpitations, with headaches and anxiety attacks | Hypoglycaemia, Phaeochromocytoma (especially if paroxysmal hypertension) | Fasting plasma glucose (if symptomatic serum must be frozen within 30 minutes for insulin). If phaeochromocytoma suspected seek specialist advice |
Goitre | Hypothyroidism or hyperthyroidism | TSH and FT4 |
Previous medical history | Possible disorder(s) | Suggested initial test(s) |
Renal stones | Hyperparathyroidism | Serum calcium and albumin (PTH if calcium high) |
Known hypotensive episodes or frequent dizzy spells | Hypoadrenalism (primary or secondary) | Urea and electrolytes, serum cortisol (9 am if possible), ACTH |
TB with recent hypotension and/or fatigue | Hypoadrenalism (primary) | Urea and electrolytes, serum cortisol (9 am if possible) |
Autoimmune disease, e.g. hypothyroidism, Addison’s disease, type 1 diabetes | Another coexisting autoimmune disease, e.g. hypothyroidism, Addison’s etc | Investigate selectively according to personal history and clinical signs |
Family history | Possible disorder(s) | Suggested initial test(s) |
Type 2 diabetes | Type 2 diabetes mellitus | Random plasma glucose if asymptomatic. If symptomatic then fasting plasma glucose (GTT if FPG ≥ 6) |
Autoimmune disease (incl. type 1 DM) | Another coexisting autoimmune disease, e.g. hypothyroidism, Addison’s etc | Investigate selectively according to personal history and clinical signs |
Multiple endocrine neoplasia syndrome (MEN) | Type 1 associated with hyperparathyroidism, pituitary hormone excess and pancreatic hormone excess. Type 2 associated with hyperparathyroidism, medullary carcinoma of thyroid and phaeochromocytoma | Type 1: calcium. Type 2: calcium and 24 hour urinary catecholamines |
Figure 8.3: A plan of investigation when hypopituitarism is suspected
Suggested investigations for the other endocrine diseases previously described are presented below.
Diabetes mellitus
As diabetes is much more common than many other endocrine disorders, affecting up to 5% of the population, the index of suspicion can be relatively low when judging whether to screen asymptomatic individuals. Evidence-based guidelines do not support routine screening, but it is common practice in many preadmission clinic units to screen for diabetes, particularly if the population served has a high preponderance of Asian, Afro-Caribbean and/or elderly patients.
Where screening is advocated, local protocols should be agreed. However, the use of random blood glucose testing is usually preferable to urine testing, as the latter can be poorly done unless the standard of staff training is high and dipstick readers are widely available.
A random plasma glucose ≥ 8mmol/L should be followed up with a fasting blood glucose (FBG). If other abnormal or equivocal blood glucose results have been obtained previously then an oral glucose tolerance test (OGTT) may be appropriate. If RBG is ≥ 11.1mmol/L or FBG is ≥ 7.0mmol/L then this is diagnostic for diabetes in a symptomatic patient. In an asymptomatic patient, a further test, e.g. a repeat FBG or RBG should be performed on a subsequent day to confirm the diagnosis.
Measurement of HbA1c can be useful in a preadmission clinic for patients with previously undiagnosed diabetes, as it enables identification of those requiring intervention to improve diabetes control prior to surgery, helping to reduce postoperative complications. An HbA1c <7.5% suggests reasonable diabetic control prior to surgery whereas above 7.5% active management is indicated. Perioperative requesting of HbA1c is unnecessary if the RBG is >13 mmol/L or FBG is > 7.8mmol/L as active management is indicated anyway.
Once a patient with previously undiagnosed diabetes has been identified, other risk factors such as hypertension or peripheral vascular disease should be assessed.
Thyroid disorders
For the purpose of preoperative screening, TSH alone is recommended. Only if the TSH is above or below the reference interval is follow-up testing of free T4 and possibly free T3 required. A normal TSH confirms euthyroid status. Uncontrolled hypothyroidism or hyperthyroidism both represent significant risks for anaesthesia and surgery. Therefore, where possible, surgery should be postponed until thyroid disease is better controlled.
Hypoadrenalism
If patients are suspected of adrenal hypofunction (either primary or secondary) this can be screened for by checking a random serum cortisol. A daytime cortisol result of ≥ 250nmol/L in a pre-admission clinic suggests adequate adrenal reserve for minor surgery, providing the patient is not acutely ill. If clinical suspicion is high, or suspicion low but major surgery is planned, then a Synacthen test may be required to confirm adequate adrenal function.
Hyperparathyroidism
A normal serum total calcium (or corrected calcium if calculated) and phosphate is adequate for screening. Although hyperparathyroidism can exist with normocalcaemia, this is not relevant in the context of preoperative screening. However, a high normal calcium and low phosphate should raise suspicion of possible gland overactivity. A high serum calcium should be followed up by measuring parathyroid hormone (PTH). It is recommended to contact the local laboratory to check the requirements before samples for PTH are taken. PTH can be unstable if the blood is collected into certain types of sample tube and may require faster processing than other routine samples.
Cushing’s syndrome
Non-iatrogenic Cushing’s syndrome (i.e. patients not on steroids) is rare and therefore specific follow-up testing is only appropriate after consultation with a specialist. However, electrolytes and plasma glucose should be checked in patients suspected of Cushing’s syndrome to exclude significant hypokalaemia or diabetes mellitus.
Conn’s syndrome
As with Cushing’s syndrome, this condition is rare and therefore does not justify specific follow-up testing during preoperative assessment other than to exclude hypokalaemia. In the event of unexplained hypokalaemia and hypertension (generally moderate, malignant hypertension is very rare) the endocrine and clinical biochemistry teams should be contacted to advise on further investigations, which include renin and aldosterone blood tests (lying and standing), suppression tests and radiology. The interpretation of these tests is often not clear cut.
Phaeochromocytoma
This is a rare disorder but poses a potentially highly significant problem for the anaesthetist and surgeon if present. Accidental palpation of an undiagnosed tumour or the use of certain therapeutic agents may precipitate a potentially fatal surge of catecholamines. Patients often present with a history of unexplained ‘palpitations’ or ‘panic attacks’ or unexplained tachyarrhythmias, with or without hypertensive episodes. Any patient for whom there is a clinical suspicion of the presence of a phaeochromocytoma should have a 24-hour urine sample collected for catecholamines (in a bottle containing acid preservative). Because of the episodic nature of catecholamine secretion by these tumours it is usual to request three separate collections to minimise the possibility of missing the secretion. However, in a preoperative setting, unless clinical suspicion is high, a single collection is probably sufficient. This test has a significant number of false positives, and certain drugs and foods can interfere. Thus any positive result will require follow-up testing and does not necessarily mean a tumour is present. Newer tests, e.g. plasma metanephrines, are not recommended for screening purposes.
Perioperative management in patients with endocrine disease
In this section we will cover the management of diabetes and thyroid disease in depth and the other endocrine disorders briefly as they are relatively rare; we would recommend that the medical endocrine team be consulted to optimise and advise on both pre- and perioperative management of these patients.
Diabetes mellitus
We will describe the management of diabetes in the perioperative setting by considering day case minor surgery separately from major surgery and Type 1 diabetes separately from Type 2 diabetes.
Day case surgery
Type 1 diabetes
Patients with Type 1 diabetes who are scheduled for surgery after 10am can be permitted to have their breakfast together with the morning insulin. A sliding scale of insulin replacement (please refer to local hospital guidelines) can be initiated an hour prior to surgery. Should the patient be scheduled first on the theatre list, the sliding scale could be started two to three hours prior to surgery.
Perioperatively the sliding scale is continued with intravenous fluids and close monitoring of blood sugar is recommended during and immediately after surgery. One strategy to avoid hypoglycaemia and hypovolaemia is to use the GKI protocol (10% dextrose, 20mL KCL and actrapid infused at 80mL per hour with an increase in infusion rate if hypoglycaemia develops).
Postoperatively if the patient is able to begin oral feeding the GKI protocol can be discontinued and the patient’s subcutaneous insulin regime can be recommenced. Should oral feeding not be possible or if vomiting complicates postoperative care, then the patient should be continued on the insulin sliding scale.
Type 2 diabetes
The management of Type 2 diabetic patients varies considerably from the above. Surgery can be permitted even in the presence of significant hyperglycaemia providing there is no ketosis. Although conversion to an insulin regime is often unnecessary, the surgeon or anaesthetist may insist on tighter glycaemic control and a sliding scale can be initiated as for the Type 1 diabetic patient.
In patients presenting with Type 2 diabetes controlled with diet, regular blood glucose monitoring (pre-breakfast and two hours after main meals while an inpatient) should be the only extra precaution in addition to those carried out for a non-diabetic patient. However, should complications arise that result in hyperglycaemia and ward admission interim, conversion to insulin therapy may be necessary.
Patients on metformin and/or a thiazolidinedione, as either monotherapy or part of the oral hypoglycaemic regime, should be treated with these agents on the morning of surgery, regardless of whether breakfast is given. Neither agent is associated with resulting hypoglycaemia, unlike sulphonylureas. When a sulphonylurea is part of the regime it should be omitted. Insulin is not generally required for these patients, even if hyperglycaemia occurs, and the patient can restart the entire preoperative treatment regime once oral feeding commences.
Major surgery
Management of hyperglycaemia is more critical when major surgery is scheduled. Preferably, Type 1 diabetic patients and insulin-treated Type 2 diabetic patients should be first on the surgical list. Oral intake is stopped from midnight and an insulin sliding scale and a GKI protocol can be initiated in the morning. However, should this not be possible or if the surgical list is scheduled in the afternoon a ‘nil by mouth’ restriction on intake should be placed four to six hours prior to surgery and an insulin sliding scale with fluids should be commenced. The sliding scale and fluids should be continued until oral intake is recommenced. At this point the Type 1 diabetic patient may return to the preoperative insulin regime, although the dose may have to be titrated depending on nutritional intake.
Patients with diet-controlled Type 2 diabetes need to have regular checks (every two to four hours) of blood glucose during and after major surgery. Insulin may not be needed if preoperative blood glucose remains consistently below 10mmol/L and close monitoring is essential. If the glucose concentration becomes erratic postoperatively, or the patient becomes unstable, then a sliding scale regime can be initiated. A similar management strategy applies to patients on metformin monotherapy (metformin should not be discontinued). However, if the patient is on sulphonylurea, it should be omitted with the discontinuation of oral feeding prior to surgery.
On perioperative checking if the glucose remains between 10 and 15mmol/L, then the patient must be monitored closely and the diabetes specialist nurses consulted. Should the glucose levels be persistently above 15mmol/L, insulin therapy should be initiated in the short term, again with management advice from the diabetes specialist nurses.
Thyroid disorders
When a treated thyrotoxic patient presents (clinically euthyroid) for day surgery, the carbimazole or propylthiouracil should be given as usual and continued thereafter. A similar approach is taken when preparing a patient for major surgery; however, perioperatively the patient should be monitored for signs of thyroid crisis. Intravenous propranolol can be used to control tachycardia.
If a patient presents for day surgery in a thyrotoxic state, the surgery should preferably be deferred, with the medical teams’ advice sought and appropriate treatment begun. When elective major surgery is planned for a patient who is clinically thyrotoxic, the risks of surgery should be balanced against those of not carrying out the procedure. If surgery is carried out then appropriate emergency care protocols must be followed.
If hypothyroidism is diagnosed when surgery is initially planned, thyroxine should be commenced (dose depends on the thyroid function tests and the severity of the symptoms and advice should be sought from the endocrine team). TSH and Free T4 should be checked, preferably a week before surgery, allowing further titration of the thyroxine replacement dose. As absorption is rapid, thyroxine can be given even on the morning of surgery.
Perioperative steroid cover for patients on long-term steroid treatment
Many patients undergoing surgical procedures may have abnormalities of the hypothalamicpituitary-adrenal (HPA) axis. This may be due to primary pituitary disease, e.g. hypopituitarism or following treatment for a pituitary tumour) or adrenal hypofunction (Addison’s disease). However, more commonly it is due to suppression of endogenous glucocorticoid production due to the administration of high doses of steroids.
The physiological stress of surgery is a potent activator of the HPA axis in normal subjects and failure to provide adequate steroid cover may precipitate potentially fatal acute adrenal insufficiency following surgery. Normal endogenous cortisol production is approximately 15–25mg per day. Patients receiving hydrocortisone replacement for primary pituitary or adrenal failure typically receive 20–30mg daily. See Table 8.8 for equivalent doses.
Table 8.8 Approximate equivalent doses
Hydrocortisone | 20mg | |
= Cortisone | 25mg | |
= Prednisolone | 5mg | i.e. 4 x potent c.f. hydrocortisone |
= Methyprednisolone | 4mg | i.e. 5 x |
= Dexamethasone | 0.5 mg | i.e. 40 x |
The following categories of patients require steroid cover:
• any patient with known past history of hypopituitarism or Addison’s disease who is on long-term replacement steroids
• any patients on therapeutic glucocorticoids with clinical features of Cushing’s syndrome
• patients who have received > 10mg prednisolone (or equivalent, see Table 8.8) daily for > 3 weeks continuously in the last 3 months
• patients taking high dose glucocorticoids (e.g. 4mg dexamethasone t.d.s.), even for short periods, who may have significant adrenal suppression for many months
• patients taking high dose inhaled steroids long-term (i.e. fluticasone ≥1000mcg daily or >1500mcg of other inhaled steroids).
Suggested replacement doses
Traditionally, large doses of IV hydrocortisone have been suggested, which were largely empirical and based on little evidence. Overtreatment with steroids perioperatively is of concern due to its possible effects on wound healing, immune function and glucose tolerance. The likelihood of a patient having HPA axis suppression depends on the dose of steroid received and duration of therapy.
For high dose steroid regimes, HPA axis suppression may last for several months. Therefore a detailed past medical and drug history is essential. In the majority of cases, testing for HPA axis suppression is unnecessary but a Synacthen test may be helpful in a minority of cases, e.g. inhaled steroids.
Table 8.9 Suggested steroid regimes1
Current steroid regime | Type of surgery | Perioperative treatment |
Low dose (< 10mg prednisolone or equivalent daily) | Assume normal HPA response | Additional steroid cover unnecessary |
Moderate dose (> 10mg Prednisolone or equivalent daily) | Minor surgery | 25mg hydrocortisone @ preop or induction. Resume normal dose postop |
Moderate surgery | Usual preop dose + 25mg hydrocortisone @ induction + 100 mg i.v. for 24 hours | |
Major surgery | Usual preop dose + 25mg hydrocortisone @ induction + 100 mg i.v. for 48–72 hours | |
Immunosuppressive doses | Give usual doses throughout perioperative period | |
Steroids stopped < 3 months | Treat as if on steroids | |
Steroids stopped > 3 months | No perioperative steroids necessary |
Renal impairment and preoperative management
As there are usually no specific symptoms, renal impairment is picked up in one of the following two ways:
1. routine biochemical testing (increased urea, creatinine and reduced estimated glomerular filtration rate (GFR))
2. patient presenting with features associated with renal dysfunction, e.g. hypertension, oedema, haematuria etc
Increasing numbers of patients are now picked up following the introduction of formula-based estimations of GFR (eGFR), which are now routinely provided by all clinical biochemistry laboratories using the abbreviated 4-variable MDRD study equation, as recommended in The National Service Framework for Renal Services.2
The initial approach should be to evaluate the cause and the severity of renal impairment. This includes:
1. estimation of disease duration (acute or chronic)
2. examination of the urine
3. estimation of the glomerular filtration rate (eGFR).
Acute renal failure (ARF) is worsening of renal function over hours/days, resulting in increasing urea and creatinine concentrations, often associated with coexisting medical or surgical conditions. The previous biochemistry is useful in making this distinction. Identification of normal urea and creatinine concentrations over the preceding weeks/months helps define acute renal failure. In the absence of previous records, the presence of anuria/oliguria, hypotension, sepsis, dehydration, and clinical evidence of fluid loss point at acute renal failure. It is important to examine all medications, as drugs can cause or exacerbate renal failure.
In contrast, chronic renal failure (CRF) results from increased loss of renal function over a longer period, a progressive rise in creatinine and a steady fall in eGFR. While anuria/oliguria is unusual, anaemia and small kidneys (on ultrasound examination) are more likely in chronic renal failure. Distinguishing acute and chronic renal failure is important for appropriate management, both immediate and long-term.
Chronic renal failure is currently graded according to the eGFR with actions dependent on the stage and progression. It must be kept in mind that eGFR will decrease with age. (see Table 8.10).
Table 8.10 Gradations of severity of renal impairement:
Grade 1 | Normal GFR + proteinuria | >90ml/min/1.73m2 |
Grade 2 | Mild CKD | 90–60ml/min/1.73m2 |
Grade 3 | Moderate CKD | 60–30ml/min/1.73m2 |
Grade 4 | Severe CKD | 30–15ml/min/1.73m2 |
Grade 5 | End stage renal disease | <15ml/min/1.73m2 |
While patients included in grades 1–3 are asymptomatic, in grade 4 non-specific symptoms are often evident. Figure 8.4 shows a treatment algorithm for investigating patients with reduced GFR.
The cause of the renal dysfunction can be further anatomically classified as pre-renal, intrinsic and post-renal (see Table 8.11, page 164).
Table 8.11 Causes of renal failure
Acute renal failure | ||
Pre-renal | Renal | Post-renal |
Hypovolaemia blood loss Dehydration diarrhoea, vomiting, etc Medications diuretics Ischaemia (renal artery) embolism, aortic dissection etc Renal vein thrombosis | Sepsis Medications NSAID, ACEI, ARB, contrast medium, lithium Rhabdomyolysis Myeloma Acute glomerular nephritis primary glomerular secondary – SLE, vasculitis, etc | Obstructive bladder outlet, ureter abdominal or pelvic mass prostatic pathology renal stones |
Chronic renal failure | ||
Pre-renal | Renal | Post-renal |
Acute or chronic similar causes as in ARF | Diabetic nephropathy Hypertensive nephropathy Chronic glomerulonephritis Polycystic kidneys Reflux nephropathy Renal stones | Obstructive similar causes as in ARF |
The following points must be remembered in the preoperative management of ARF.
• The fluid status of the patient must be estimated and appropriately corrected. This is essential, as prolonged hypotension can result in irreversible renal damage.
• Careful monitoring of both input and output of fluids is essential.
• All potentially nephrotoxic drugs must be discontinued. The advice of relevant specialists must be sought if possible if a new drug is substituted.
• The critical care team must be involved early as patient care may need to be optimised preoperatively.
• In the event of the patient remaining hypotensive despite adequate fluid replacement, ionotropic support may be required.
• In severe ARF, or in the event of mild to moderate renal impairment not significantly improving, or worsening, the renal team must be consulted; interim haemofiltration/dialysis may be required prior to surgery.
• Give smoking and weight advice
• Treat BP with target <130/80
• Suggest patient buys own BP meter
• Treat hyperlipidaemia
• If urine protein? + and blood ≥ +, culture MSU. If no significant growth, refer to nephrologist for exclusion of glomerulonephritis.
• If urine protein ≥ ++ and blood negative, refer to nephrologist for consideration of renal biopsy.
• Recheck GFR at 6–12 months If rate of decline >5ml/min/1.73m2/year refer to, or discuss with nephrologist.
Figure 8.4: Algorithm for the management of patients with reduced glomular filtration rate (GFR)
Patients diagnosed as CKD grades 1–3 need judicious fluid and electrolyte replacement with careful assessment to avoid dehydration leading to further deterioration of renal function or fluid overload. Patients classed as grade 4 CKD need to be reviewed and managed by a nephrologist (during the entire period of admission) ahead of surgery.
REFERENCES
1. G. Nicholson, J.M. Burrin and G.M. Hall (1998). Peri-operative steroid supplementation. Anaesthesia 53: 1091–104.
2. Department of Health (2004). The National Service Framework for Renal Services. London: HMSO.
FURTHER READING
British Association of Day Surgery Working Group (2004). Day Surgery and the Diabetic Patient: Guidelines for the assessment and management of diabetes in day case surgery patients. British Association of Day Surgery Handbook 2nd edn. London: BADS.
Royal College of Physicians of London (2006). Chronic Kidney Disease in Adults: UK Guidelines for Identification, Management and Referral. Available at: www.renal.org/CKDguide/full/UKCKDfullguide.pdf, accessed 08/06/08.
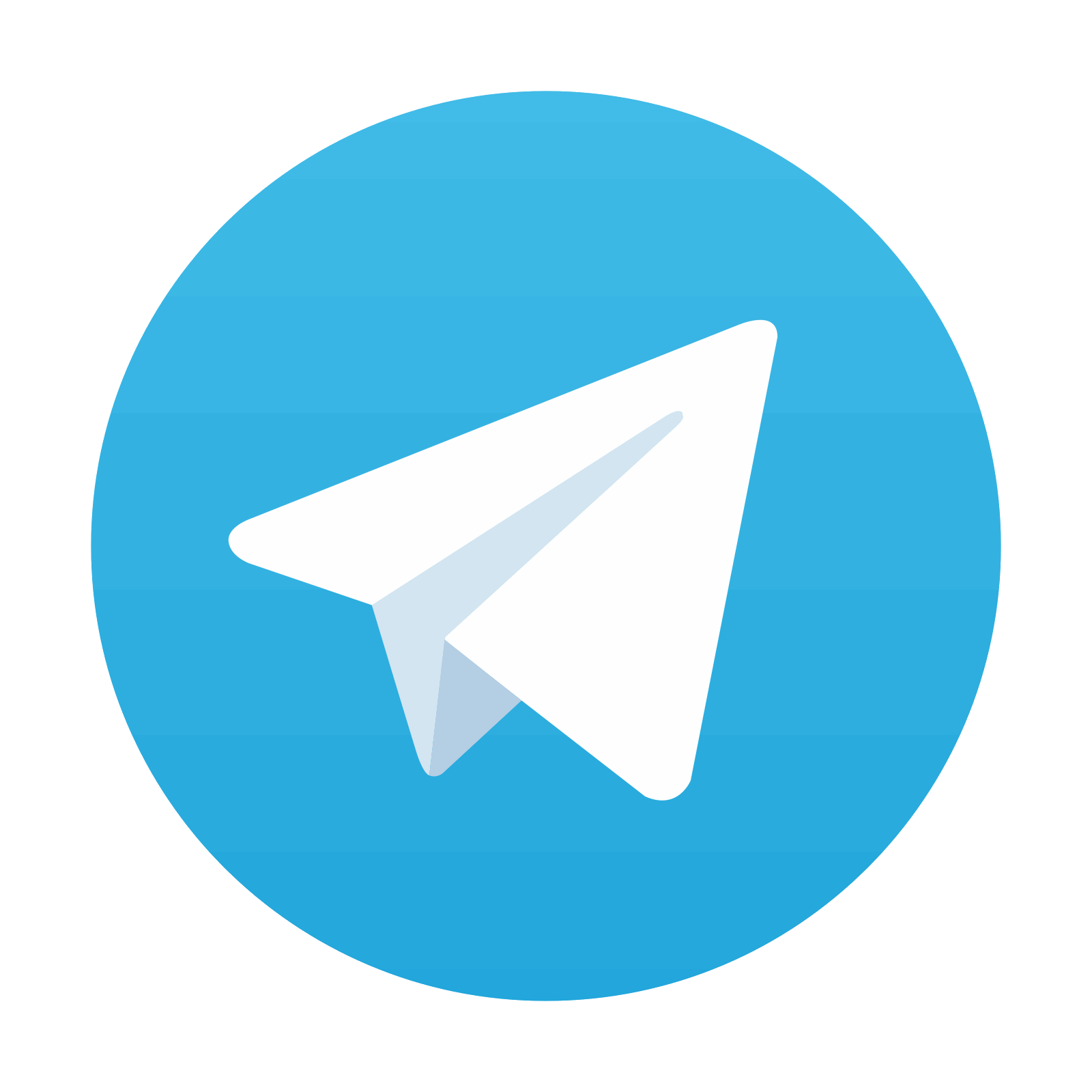
Stay updated, free articles. Join our Telegram channel

Full access? Get Clinical Tree
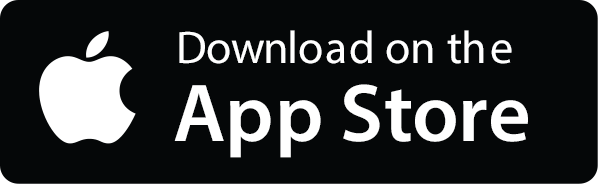
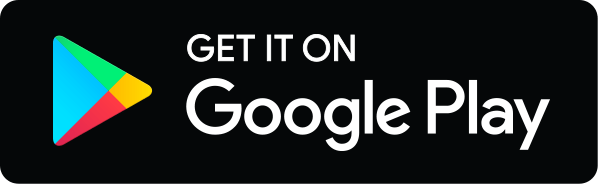