Fig. 10.1
(a) Cardiac tamponade in lung transplant recipient with oversized allografts (during expiratory phase of the ventilatory cycle). Transesophageal echocardiogram (TEE) image obtained at mid-esophageal level depicting the right atrium (RA) and right ventricle (RV) cavities. The image demonstrates the RA and RV normally open during diastole. This patient was intubated and mechanically ventilated. TEE was performed in order to evaluate the possible etiology of hemodynamic instability in the immediate postoperative period after bilateral lung transplantation. (b) Cardiac tamponade in lung transplant recipient with oversized allografts (during inspiratory phase of the ventilatory cycle). Transesophageal echocardiogram (TEE) image obtained at mid-esophageal level depicting the right atrium (RA) and right ventricle (RV) cavities. The image demonstrates the RA and RV are completely collapsed by extrinsic compression during diastole. TEE examination confirmed diagnosis of cardiac tamponade caused by increased intrathoracic pressure in the setting of positive pressure ventilation and oversized allografts
Acute Postoperative Respiratory Care of the Lung Transplant Patient
The goals for postoperative respiratory management are to provide adequate gas exchange that will meet the metabolic needs of the patient while limiting ongoing perioperative lung injury and minimizing ventilator-associated complications. Optimal respiratory management in the immediate postoperative period can also decrease the possibilities of both, acute and long-term complications. The mainstay interventions are the use of protective ventilatory strategies, early extubation, optimization of respiratory mechanics, and obtaining the best pain control possible.
In the immediate postoperative period, frequent assessment of gas exchange by arterial blood gas measurement is essential to determine stable lung function, to safely decrease inspired oxygen concentration (minimizing oxygen toxicity) and to help detect early life-threatening complications (e.g., hyperacute rejection, primary graft dysfunction, etc.). Additionally, periodic determination of mixed venous oxygen saturation allows evaluation of global balance between oxygen delivery and consumption; the use of a continuous oximetry pulmonary artery catheter is an effective alternative. Other indirect indicators of global perfusion can help to assess the adequacy of oxygen delivery (e.g., pH, base deficit, bicarbonate level, lactic acid determination). Optimizing the global balance between oxygen delivery and consumption requires an adequate cardiac index with appropriate hemoglobin content. In this patient population it can be challenging to achieve a normal cardiac output due to the combination of postoperative acute right and/or left ventricular dysfunction, perioperative vasodilatory shock, and hypovolemia. The benefit of improved oxygen carrying capacity should be balanced against the risks of red blood cells transfusion. It is unlikely that data extrapolated from other clinical areas, regarding transfusion thresholds are adequate or appropriate in this patient population. However, red blood cells transfusions following lung transplantation have been associated with worse survival and increased infections [22]; and judicious transfusion practices are likely warranted in these patients.
The use of protective ventilatory strategies aims to prevent or reduce the incidence of acute lung injury (ALI) . Such interventions include a combination of ventilator modes and procedures that intend to decrease inflammation and diffuse alveolar damage characteristic of ALI [23]. In this environment, protective ventilatory strategies can limit volu- and barotrauma, minimize pulmonary endothelial damage, and the so-called bio-trauma secondary to cytokine release, all factors associated with occurrence of ALI [24]. The use of protective ventilatory strategies have been extrapolated, from the evidence available for use of low tidal volume in ARDS patients, into the integral intraoperative management of pulmonary resection and perioperative management of thoracic surgical patients as standardized practice [25]. Those techniques include combination of the use of low-tidal volume (6–8 ml/Kg, ideal body weight in two lung ventilation), low peak inspiratory pressure (<20 cm H2O above PEEP level), low plateau airway pressure (<30 cm H2O), moderate PEEP (8–10 cm H2O), preferable use of pressure-controlled ventilation, and intermittent recruitment maneuvers [23]. The available evidence supporting the use of lung protective strategies (compared to traditional approaches) due to lower risk of mortality, earlier extubation, lower risk of respiratory failure, decrease evidence of ALI, improved ventilation-perfusion matching, suggest that their use in lung transplantation could be beneficial [26, 27]. Additionally, minimizing inhaled fraction of oxygen (FiO2) is believed to decrease the production of oxygen free radicals and subsequent PGD. It is important to balance the effects of recruitment maneuvers in preventing atelectasis and improving oxygenation with the potential barotrauma and hemodynamic instability in lung transplant patients. There is little clinical impetus to perform frequent recruitment maneuvers provided oxygenation and ventilation remain adequate.
A ventilation weaning protocol should be implemented as soon as patient hemodynamic status is improved and gas exchange is stable. Importantly, the inspired fraction of oxygen (FiO2) should be weaned as soon as possible in order to minimize oxygen toxicity. The goal of early extubation is paramount in order to avoid complications such as hospital-acquired pneumonia. The single most important intervention to accomplish early extubation is optimization of respiratory mechanics by achieving adequate pain control. The early institution of continuous thoracic epidural analgesia is safe and effective, and provides evidence of earlier extubation, better pain relief and decreased rates of re-intubation and postoperative respiratory complications, when compared with systemic intravenous analgesia [28]. The use of thoracic epidural analgesia is part of a multifaceted approach that includes minimization of long-acting sedation medications, avoidance of systemic opioids, avoidance of postoperative neuromuscular blockade and early recovery of neuromuscular function in anticipation of early extubation.
At our institution, in order to avoid issues with intraoperative anticoagulation, epidural analgesia is instituted postoperatively, when there is clear evidence of resolution of coagulopathy. We use a combination of low concentration bupivacaine and low dose hydromorphone, which provides synergistic neuroaxial effect and have minimal systemic absorption. It is important to wait until postoperative shock and hemodynamic instability have resolved before starting epidural analgesia. In our experience, it is safe to initiate at a low infusion rate institute when inotropic and vasopressor medications are being weaned.
Optimization of respiratory mechanics and early extubation can be achieved by a multidisciplinary strategy based on thoracic epidural analgesia for acute post-thoracotomy pain control that allows several additional interventions. Such interventions include, aggressive chest physiotherapy with periodic bronchodilators and postural drainage, early mobilization out of bed and ambulation, and most importantly complemented by an intensive exercise and physical therapy plan even if the patient is still intubated. Early tracheostomy in patients with suspected prolonged intubation will facilitate management of pulmonary secretions, increase early mobilization, and optimize patient comfort [9]. Early and liberal use of bronchoscopy is of vital importance not only to detect significant early complications and to assess the status of bronchial anastomosis, but also to maximize clearance of secretions increasing the possibilities of successful extubation.
Acute Respiratory Failure After Lung Transplantation
Acute respiratory failure is the most common complication following lung transplantation. It is also responsible for a 45 % mortality rate in patients who develop it. Similarly, it is a source of significant morbidity. In the acute postoperative period, PGD, acute rejection, and surgical technical complications are the most common causes of acute respiratory failure. After 3 months, the most prevalent causes are infections, acute rejection, and obliterative bronchiolitis [29]. The most important noninfectious entities responsible for the occurrence of acute respiratory failure in the immediate postoperative period will be reviewed in the following section.
Primary Graft Dysfunction/Severe Reperfusion Injury
The term “Primary Graft Dysfunction” (PGD) describes an entity that has received different names in the past (e.g. reperfusion injury, reperfusion edema, re-implantation response, re-implantation edema, primary graft failure, and early graft dysfunction) [30]. This complication occurs in up to 25 % of lung transplant recipients and represents the main cause of early mortality and morbidity [2]. In addition, this condition has other poor outcome consequences, including increased ICU and hospital length of stay, increased duration of mechanical ventilation and decreased exercise capacity [31]. PGD is a form of early ALI that leads to acute lung allograft failure. One of the leading mechanisms responsible for this form of ALI is the development of ischemia–reperfusion injury. The clinical syndrome consists of development of acute diffuse alveolar infiltrates consistent with pulmonary edema of noncardiogenic origin and secondary severe hypoxemia.
The ISHLT Working Group on PGD provided a standardized definition in their 2005 consensus statement. The definition includes the presence of “diffuse allograft alveolar infiltrates” by radiography within 72 h of reperfusion and hypoxemia based on partial pressure of oxygen (PaO2) to FiO2 ratio (PaO2/FiO2) (Table 10.1). The working group recommends inclusion of the timing of lung dysfunction (T 0—within 6 h of lung perfusion, T 24, T48, and T72—24, 48, 72 h after first blood gas) [30]. They also recommend mentioning certain subgroups in the description while defining PGD; pulmonary venous occlusion, left ventricular dysfunction, hyper-acute rejection, and infectious process. Importantly, patients who develop grade 3 PGD have increased long-term mortality, as well as increased incidence of chronic allograft rejection (or Bronchiolitis Obliterans syndrome) and decreased maximum allograft function [22, 32] The clinical risk factors identified for PGD are: transplantation of an organ with donor history of smoking, elevated FiO2 during allograft reperfusion, preoperative sarcoidosis or pulmonary arterial hypertension, use of CPB, single lung transplant, large-volume blood product transfusion, elevated postoperative pulmonary arterial pressures, and overweight or obese recipient body habitus [22]. Other previously associated factors included prolonged ischemic time, donor lung contusion or aspiration, and the use of high-potassium preservation solutions [33, 34]. The mechanisms involved are multiple but they involve a common path to endothelial and epithelial lung damage, which includes release of cytokines, cell damage by neutrophils and lymphocytes activation, complement activation, upregulation of multiple inflammatory cascade mediators, oxidative stress and reduction in endogenous NO production. The final result is increased cell death and apoptosis [35–37].
Table 10.1
Recommendations for grading of primary graft dysfunction severity
Grade | PaO2/FiO2 | Radiographic infiltrates consistent with pulmonary edema |
---|---|---|
0 | >300 | Absent |
1 | >300 | Present |
2 | 200–300 | Present |
3 | <200 | Present |
The above mentioned observations have led to the development of several strategies in order to prevent or reduce the incidence and severity of PGD with particular emphasis in modifiable clinical risk factors observed in a large prospective study (e.g., FiO2 on reperfusion, avoiding the use of CPB, and pretransplant recipient weight loss) [22]. Otherwise, suggested preventive interventions include standardizing enhanced procurement and preservation of donor lung techniques, controlled intraoperative reperfusion pressures, and leukocyte depletion of transfused blood products. Interestingly, there are reports about the use of NO added to the flush solution at time of harvest, as well as early use of iNO to attenuate the incidence and severity of PGD [38–40]. Inhaled NO is effective in reducing pulmonary arterial pressure, improving ventilation-perfusion matching and optimizing PaO2/FiO2 ratio, and has potential anti-inflammatory properties [9, 29, 40]. The use of this and other approaches deserve further investigation in order to address if they improve outcomes. However, neither iNO nor other pharmacologic interventions when formally studied have been shown to be clearly effective in preventing PGD following lung transplantation [41–43].
The mainstay of critical care management and treatment of patients with PGD is a multisystem approach that minimizes further injury by using low pressure protective ventilatory strategies, and by controlling existing pulmonary edema and minimizing further pulmonary edema formation. The use of veno-venous ECMO in cases of severe PGD intends to avoid the use of injurious mechanical ventilator settings, therefore avoiding additional pulmonary insult [44]. Otherwise, treatment and prevention of pulmonary edema requires the use of intravenous diuretics and a fluid management strategy that avoid pulmonary vascular congestion. In a recent study, a central venous pressure (CVP) > 7mmHg was associated with prolonged mechanical ventilation and also with a tendency to increased ICU and in-hospital mortality [45]. Nonetheless, it is important to highlight that is necessary to assume an strategy with careful hemodynamic monitoring and management relying in the use of inotropes, pulmonary vasodilators, and vasopressors; while minimizing intravascular volume resuscitation and maintaining hemodynamic stability and adequate global perfusion and oxygen delivery. Typically a pulmonary artery catheter is required to provide pertinent hemodynamic measurements and help guide resuscitative efforts.
Acute Rejection
The occurrence of hyperacute/humorally-mediated rejection is uncommon in the literature, but is more likely in patients with high levels of preformed anti-Human Leukocytes Antibodies (HLA) (i.e. panel reactive antibody (PRA)) [9]. The clinical syndromes of acute rejection , infection, and PGD can be difficult to differentiate. Patients with acute rejection present with inflammatory response-like syndrome (including low-grade fever) and dyspnea, along with hypoxemia and perihilar infiltrates. In many cases, patients receive empiric therapy for rejection after infectious etiologies are ruled out. In this regard, acute rejection findings are nonspecific and the diagnosis is made retrospectively. The gold standard for diagnosis of acute rejection is the pathologic evaluation of serial transbronchial biopsies. However, the use of transbronchial biopsies can underestimate the incidence of acute rejection when compared with open surgical biopsy [46]. The risk of open surgical biopsy must be weighed against the diagnostic yield in selected cases. Acute rejection is a common complication presenting in the first year following lung transplantation, with about 40–60 % of recipients having at least one episode of acute rejection during the first year [2]. This complication is characterized by airway-centered inflammation in the pathologic form of lymphocytic bronchitis / bronchiolitis. There is a clear association between the frequency and severity of acute rejection episodes and later development of chronic allograft dysfunction characterized by bronchiolitis obliterans . The treatment of acute rejection aims to address the acute episode and to decrease the possibilities of further events. The main therapy is the use of methylprednisolone 10–15 mg/kg for 3–5 days, followed by an oral steroid taper for 2–3 weeks, depending on the maintenance steroid dose. There is some controversy as to the need to treat clinically undetectable grade 1 acute rejection episodes that may be diagnosed by surveillance bronchoscopy. Much emphasis in the prevention of acute rejection is made on the use of induction protocols based on interleukin-2 receptor blockers (e.g. basiliximab) or antibody depletion with antithymocyte globulin.
Dynamic Lung Hyperinflation
This complication occurs in patients who have received single lung transplantation for emphysematous diseases. In the postoperative period, there is preferential gas flow to the highly compliant, diseased, native lung with its progressive over distension and potential mediastinal shift with hemodynamic instability in severe cases. The pathophysiology of this entity is caused by the difference of compliance between the donor allograft and the remaining lung, which causes differential gas flow with positive pressure ventilation. That process is aggravated by the occurrence of PGD in the allograft. In such cases, the donor lung becomes less complaint, causing further native lung over distension. As a result, the PVR in the native lung increases and causes increased shunting of blood to the dysfunctional lung allograft with subsequent worsening of ventilation-perfusion mismatch. Management of this situation requires use of ventilator strategies that avoid air trapping, including decreasing tidal volume, lower respiratory rates, low PEEP, and long expiratory times; or converting the patient to a spontaneous ventilatory mode whenever possible. The patient should also receive aggressive bronchodilator therapy and lateral decubitus ventilation with the transplanted side up in order to maximize gas flow to the allograft. This approach can cause a mild degree of hypoxemia and hypercarbia with respiratory acidosis that are usually transient and well tolerated. In cases of hemodynamic instability or severe hypoxemia and hypercarbia, the patient can be managed with independent / differential mechanical ventilation, after other causes of instability and hyperinflation, such as tension pneumothorax and allograft mucous plugging are ruled out. This later approach requires placement of a double lumen endotracheal tube and use of separate mechanical ventilators with settings appropriate for each lung. Limitations for the use of this technique are the specialized management required for endotracheal tube placement, and the challenge that represents maintenance of proper position. Dynamic hyperinflation improves when the compliance of the transplanted lung increases, usually after 24–72 h. Although there is not enough evidence of improved outcomes to support the routine use of this technique, there are reports of successful management in several patients [47, 48].
Noninfectious Airway Complications
There are multiple possible airway complications after lung transplantation including, bronchial anastomotic dehiscence, bronchial stenosis, obstructive granulomas, bronchomalacia, and bronchial fistula formation [49]. The incidence of central airway complications after lung transplantation ranges from 9 to 33 % with an associated mortality of 2–4 %. Importantly, among all airway complications, 9–13 % will require intervention [50]. The recognized risk factors for airway complications in lung transplantation are: ischemia of donor bronchi, surgical technique used, length of donor bronchi, presence of donor or recipient colonization or infection, donor/recipient bronchial size discrepancy, postoperative infection, postoperative mechanical ventilation, and use of immunosuppression [51, 52]. The improvement in surgical techniques as well as in donor and recipient management has greatly reduced the incidence of these complications. The most common airway complication is bronchial stenosis. This complication most commonly occurs between 2 and 9 months after transplantation. Such stenosis occurs after significant necrosis, dehiscence, and infections, particularly with Aspergillus species [50, 53, 54]. Another important complication is bronchial anastomosis dehiscence, which usually occurs within the first 5 weeks after transplantation, as a result of mucosal necrosis. This complication, thought quite uncommon is associated with high mortality and morbidity [55]. Although most airway complications are not fatal, they impose a high rate of morbidity and can require complex and multidisciplinary management that include interventional bronchoscopic airway treatment and airway stent placements. Ultimately, bronchomalacia and bronchial strictures can be the long-term result of anastomotic dehiscence and infections [49].
Acute Kidney Injury After Lung Transplantation
Acute kidney injury (AKI) is a common complication after lung transplantation. Data from the recent 2013 ISHLT registry report showed that 23.3 % of lung transplant patients developed AKI within 1 year, and 55.4 % within 5 years. More importantly, according to the registry, patients have a high incidence of severe renal dysfunction given the fact that 24 % of patients develop creatinine >2.5 mg/dl, dialysis or transplant requirement within 5 years after lung transplantation. In a similar fashion, 41 % of patients will experience any of the same complications within 10 years. More specifically, the need for chronic dialysis is 1.7 % within 1 year and increases to 3.2 % within 5 years [2]. In contrast, two recent studies of early AKI demonstrated a higher incidence of AKI by using RIFLE criteria (39 and 54 %, within 72 h and 30 days after lung transplantation, respectively). Both studies also concluded that AKI is more common after bilateral lung transplantation. Another factor associated with the development of AKI after lung transplant is high volume blood transfusion. Those findings suggest the influence of surgical and perioperative factors, as well as procedural complexity in the occurrence of AKI after lung transplantation [56]. This is in opposition to the traditional belief that the dominant etiology for AKI in transplant patients is nephrotoxicity from immunosuppressant medications, in particular cyclosporine and tacrolimus [56, 57].
It is also noteworthy that hypovolemia and vasopressors have not been identified as risk factors in contemporary studies [56]. The most important implication of AKI after lung transplant is its association with poor outcomes, including increased duration of mechanical ventilation and hospital length of stay [56]. Long-term, the main determinants of the progression of renal dysfunction remain pretransplant renal function and the severity of AKI in the early period after transplantation. Therefore, minimal GFR requirements (40–50 ml/min) should be established during the evaluation phase [58, 59]. Likewise, renal function after transplant requires close monitoring because the more severe the AKI after lung transplant, the more rapid the progression of renal dysfunction [60]. Other risk factors associated with long-term renal impairment are the presence of diastolic hypertension, and the use of cyclosporine instead of tacrolimus. Additional mechanisms implicated in the development of chronic kidney disease are the use of nephrotoxic medications other than calcineurin inhibitors, such as amphotericin and aminoglycosides. Identification of patients at risk allows for early detection of any degree of AKI, avoidance of secondary injury, adjustment of immunosuppression medication (e.g., lower doses, substitution therapy), and aggressive treatments of hypertension and diabetes, particularly with optimal blood glucose control.
Hematologic Complications After Lung Transplantation
Thrombotic Thrombocytopenic Purpura (TTP)/Hemolytic–Uremic Syndrome (HUS)
Both HUS and TTP are uncommon but potentially fatal causes of acute renal failure that occur as complications after solid organ transplantation. These syndromes are characterized by thrombotic microangiopathy due to platelet activation and microthrombi formation with consequent thrombocytopenia, hemolysis, and renal failure. Their occurrence is associated with the use of calcineurin inhibitors by poorly understood mechanisms. These syndromes occur early after lung transplantation, most commonly within 3 months. The mainstay of treatment is plasma exchange therapy , which can be combined with antiplatelet agent and glucocorticoids [61].
Heparin-Induced Thrombocytopenia/Thrombosis (HIT/T)
Thrombocytopenia remains a very common finding following lung transplantation. It is routinely a self-limiting state that reverts over the first few days after surgery. However, it can occasionally require platelet transfusion and can be associated with bleeding complications. Mechanical circulatory support, such as ECMO for PGD, commonly causes thrombocytopenia. Likewise, antimicrobial medications such as gancyclovir and voriconazole often lead to platelet consumption. Heparin-Induced Thrombocytopenia (HIT) occurs in up to 10–15 % of lung transplant recipients [62], as noted by presence of PF-4 antibodies or positive serotonin release assay. This can occasionally lead to a thrombotic phenotype (HITT) causing ubiquitous complications including deep venous thrombosis, pulmonary emboli, strokes, and ischemia. Treatment of this serious condition requires anticoagulation with pharmacologic agents alternatives to heparin, such as bivalirudin or argatroban, in order to minimize diffuse thrombotic events.
Venous Thromboembolic Disease, Including DVT and PE (VTE)
VTE occurs in up to a third of lung transplant recipients and can lead to symptomatic limb swelling, central venous occlusion, and pulmonary embolism. In addition to the usual risk factors of major surgery and immobilization, the placement of indwelling central lines during surgery, as well as long-term IV access such as with peripherally inserted central catheters (PICC) , can lead to lower or upper extremity DVTs . Sequential compression devices are routinely used on the lower extremities with uncertain benefits. However, early extubation, adequate pain control, and rapid mobilization are likely the best defenses against venous thrombus formation. The role of routine DVT prophylaxis with subcutaneous unfractionated or low molecular weight heparin (LMWH) is not firmly established in these patients. The bilateral thoracotomy incisions and extensive dissection of the hilar structures provide ample soft tissue for postoperative hemorrhage to occur and posttransplant decreases in GFR due to AKI may decrease LMWH clearance and increase the risk of bleeding. Although additional research is needed in this area, implementing routine DVT prophylaxis in our patients did not alter the rate of perioperative VTE complications, but was associated with an increase in bleeding complications [63].
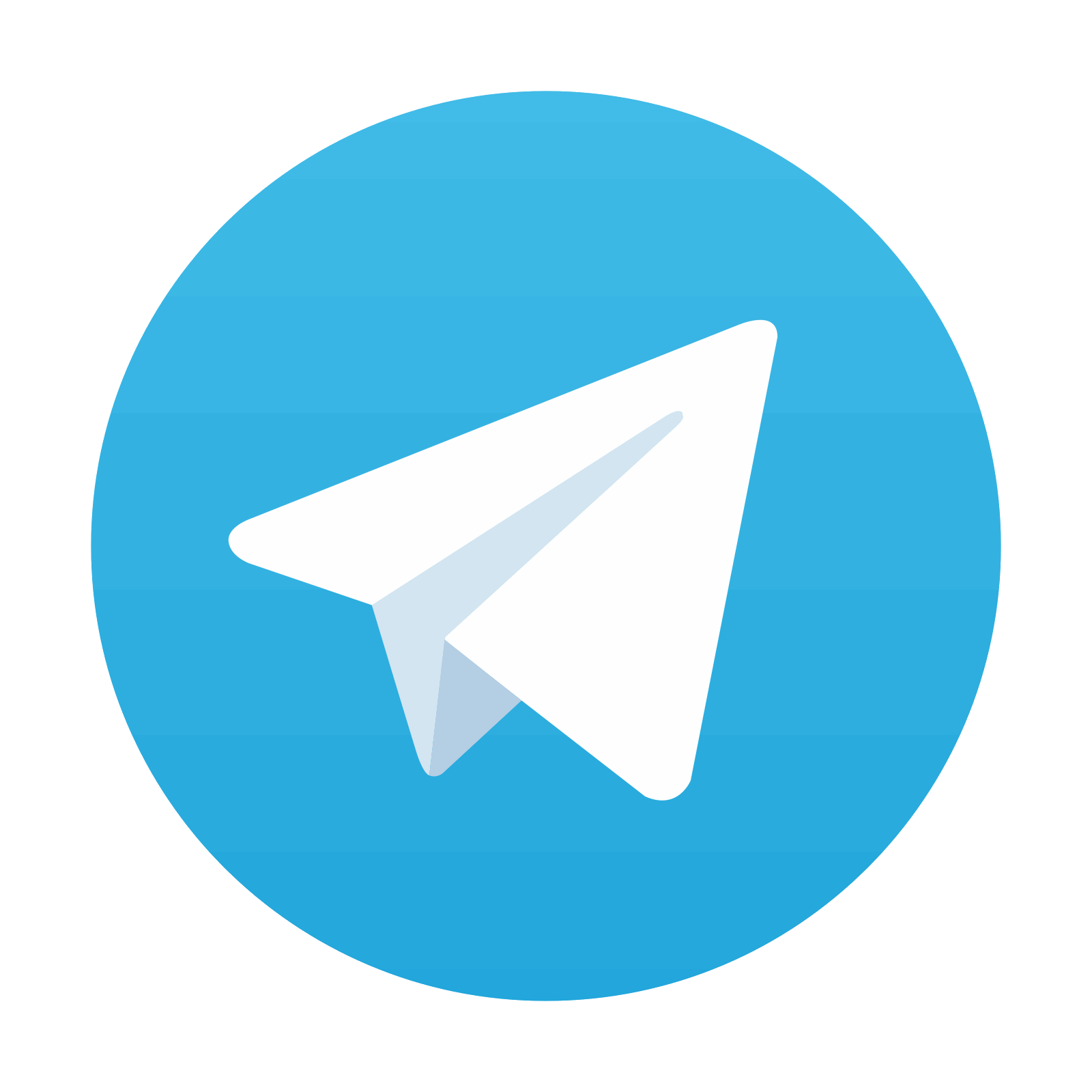
Stay updated, free articles. Join our Telegram channel

Full access? Get Clinical Tree
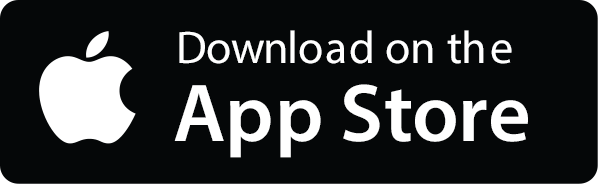
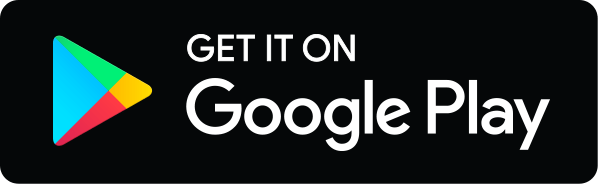