Key Concepts
Drug molecules obey the law of mass action. When the plasma concentration exceeds the tissue concentration, the drug moves from the plasma into tissue. When the plasma concentration is less than the tissue concentration, the drug moves from the tissue back to plasma.
Most drugs that readily cross the blood-brain barrier (eg, lipophilic drugs like hypnotics and opioids) are avidly taken up in body fat.
Biotransformation is the chemical process by which the drug molecule is altered in the body. The liver is the primary organ of metabolism for drugs.
Small unbound molecules freely pass from plasma into the glomerular filtrate. The nonionized (uncharged) fraction of drug is reabsorbed in the renal tubules, whereas the ionized (charged) portion is excreted in urine.
Elimination half-life is the time required for the drug concentration to fall by 50%. For drugs described by multicompartment pharmacokinetics (eg, all drugs used in anesthesia), there are multiple elimination half-lives.
The offset of a drug’s effect cannot be predicted from half-lives. The context-sensitive half-time is a clinically useful concept to describe the rate of decrease in drug concentration and should be used instead of half-lives to compare the pharmacokinetic properties of intravenous drugs used in anesthesia.
Pharmacological Principles: Introduction
The clinical practice of anesthesiology is connected more directly than any other specialty to the science of clinical pharmacology. One would think, therefore, that the study of pharmacokinetics and pharmacodynamics would receive attention comparable to that given to airway assessment, choice of inhalation anesthetic for ambulatory surgery, or neuromuscular blockade in anesthesiology curricula and examinations. The frequent misidentification or misuse of pharmacokinetic principles and measurements suggests that this is not the case.
Pharmacokinetics
Pharmacokinetics defines the relationships among drug dosing, drug concentration in body fluids and tissues, and time. It consists of four linked processes: absorption, distribution, biotransformation, and excretion.
Absorption defines the processes by which a drug moves from the site of administration to the bloodstream. There are many possible routes of drug administration: oral, sublingual, rectal, inhalational, transdermal, transmucosal, subcutaneous, intramuscular, and intravenous. Absorption is influenced by the physical characteristics of the drug (solubility, pKa, diluents, binders, and formulation), dose, and the site of absorption (eg, gut, lung, skin, muscle). Bioavailability is the fraction of the administered dose reaching the systemic circulation. For example, nitroglycerin is well absorbed by the gastrointestinal tract but has low bioavailability when administered orally. The reason is that nitroglycerin undergoes extensive first-pass hepatic metabolism as it transits the liver before reaching the systemic circulation.
Oral drug administration is convenient, inexpensive, and relatively tolerant of dosing errors. However, it requires cooperation of the patient, exposes the drug to first-pass hepatic metabolism, and permits gastric pH, enzymes, motility, food, and other drugs to potentially reduce the predictability of systemic drug delivery.
Nonionized (uncharged) drugs are more readily absorbed than ionized (charged) forms. Therefore, an acidic environment (stomach) favors the absorption of acidic drugs (A− + H+ → AH), whereas a more alkaline environment (intestine) favors basic drugs (BH+ → H+ + B). Most drugs are largely absorbed from the intestine rather than the stomach because of the greater surface area of the small intestine and longer transit duration.
All venous drainage from the stomach and small intestine flows to the liver. As a result, the bioavailability of highly metabolized drugs may be significantly reduced by first-pass hepatic metabolism. Because the venous drainage from the mouth and esophagus flows into the superior vena cava rather than into the portal system, sublingual or buccal drug absorption bypasses the liver and first-pass metabolism. Rectal administration partly bypasses the portal system, and represents an alternative route in small children or patients who are unable to tolerate oral ingestion. However, rectal absorption can be erratic, and many drugs irritate the rectal mucosa.
Transdermal drug administration can provide prolonged continuous administration for some drugs. However, the stratum corneum is an effective barrier to all but small, lipid-soluble drugs (eg, clonidine, nitroglycerin, scopolamine, fentanyl, and free-base local anesthetics [EMLA]).
Parenteral routes of drug administration include subcutaneous, intramuscular, and intravenous injection. Subcutaneous and intramuscular absorption depend on drug diffusion from the site of injection to the bloodstream. The rate at which a drug enters the bloodstream depends on both blood flow to the injected tissue and the injectate formulation. Drugs dissolved in solution are absorbed faster than those present in suspensions. Irritating preparations can cause pain and tissue necrosis (eg, intramuscular diazepam). Intravenous injections completely bypass the process of absorption.
Once absorbed, a drug is distributed by the bloodstream throughout the body. Highly perfused organs (the so-called vessel-rich group) receive a disproportionate fraction of the cardiac output (Table 7-1). Therefore, these tissues receive a disproportionate amount of drug in the first minutes following drug administration. These tissues approach equilibration with the plasma concentration more quickly than less well perfused tissues due to the differences in blood flow. However, less well perfused tissues such as fat and skin may have enormous capacity to absorb lipophilic drugs, resulting in a large reservoir of drug following long infusions.
Tissue Group | Composition | Body Mass (%) | Cardiac Output (%) |
---|---|---|---|
Vessel-rich | Brain, heart, liver, kidney, endocrine glands | 10 | 75 |
Muscle | Muscle, skin | 50 | 19 |
Fat | Fat | 20 | 6 |
Vessel-poor | Bone, ligament, cartilage | 20 | 0 |
Drug molecules obey the law of mass action. When the plasma concentration exceeds the concentration in tissue, the drug moves from the plasma into tissue. When the plasma concentration is less than the concentration in tissue, the drug moves from the tissue back to plasma.
Distribution is a major determinant of end-organ drug concentration. The rate of rise in drug concentration in an organ is determined by that organ’s perfusion and the relative drug solubility in the organ compared with blood. The equilibrium concentration in an organ relative to blood depends only on the relative solubility of the drug in the organ relative to blood, unless the organ is capable of metabolizing the drug.
Molecules in blood are either free or bound to plasma proteins and lipids. The free concentration equilibrates between organs and tissues. However, the equilibration between bound and unbound molecules is instantaneous. As unbound molecules of drug diffuse into tissue, they are instantly replaced by previously bound molecules. Plasma protein binding does not affect the rate of transfer directly, but it does affect relative solubility of the drug in blood and tissue. If the drug is highly bound in tissues, and unbound in plasma, then the relative solubility favors drug transfer into tissue. Put another way, a drug that is highly bound in tissue, but not in blood, will have a very large free drug concentration gradient driving drug into the tissue. Conversely, if the drug is highly bound in plasma and has few binding sites in the tissue, then transfer of a small amount of drug may be enough to bring the free drug concentration into equilibrium between blood and tissue. Thus, high levels of binding in blood relative to tissues increase the rate of onset of drug effect, because fewer molecules need to transfer into the tissue to produce an effective free drug concentration.
Albumin binds many acidic drugs (eg, barbiturates), whereas α1-acid glycoprotein (AAG) binds basic drugs (local anesthetics). If the concentrations of these proteins are diminished or (typically less important) if the protein-binding sites are occupied by other drugs, then the relative solubility of the drugs in blood is decreased, increasing tissue uptake. Kidney disease, liver disease, chronic congestive heart failure, and malignancies decrease albumin production. Trauma (including surgery), infection, myocardial infarction, and chronic pain increase AAG levels. Pregnancy is associated with reduced AAG concentrations. Note that these changes will have very little effect on propofol, which is administered with its own binding molecules (the lipid in the emulsion).
Lipophilic molecules can readily transfer between the blood and organs. Charged molecules are able to pass in small quantities into most organs. However, the blood-brain barrier is a special case. Permeation of the central nervous system by ionized drugs is limited by pericapillary glial cells and endothelial cell tight junctions. Most drugs that readily cross the blood-brain barrier (eg, lipophilic drugs like hypnotics and opioids) are avidly taken up in body fat.
The time course of distribution of drugs into peripheral tissues is complex and can only be assessed with computer models. Following intravenous bolus administration, rapid distribution of drug from the plasma into peripheral tissues accounts for the profound decrease in plasma concentration observed in the first few minutes. For each tissue, there is a point in time at which the apparent concentration in the tissue is the same as the concentration in the plasma. The redistribution phase (for each tissue) follows this moment of equilibration. During redistribution, drug returns from peripheral tissues back into the plasma. This return of drug back to the plasma slows the rate of decline in plasma drug concentration.
Distribution generally contributes to rapid emergence by removing drug from the plasma for many minutes following administration of a bolus infusion. Following prolonged infusions, redistribution generally delays emergence as drug returns from tissue reservoirs to the plasma for many hours.
The complex process of drug distribution into and out of tissues is one reason that half-lives are clinically useless. The offset of a drug’s clinical actions are best predicted by computer models using the context-sensitive half-time or decrement times. The context-sensitive half-time is the time required for a 50% decrease in plasma drug concentration to occur following a pseudo steady-state infusion (in other words, an infusion that has continued long enough to yield nearly steady-state concentrations). Here the “context” is the duration of the infusion. The context-sensitive decrement time is a more generalized concept referring to any clinically relevant decreased concentration in any tissue, particularly the brain or effect site.
The volume of distribution, Vd, is the apparent volume into which a drug has “distributed” (ie, mixed). This volume is calculated by dividing a bolus dose of drug by the plasma concentration at time 0. In practice, the concentration used to define the Vd is often obtained by extrapolating subsequent concentrations back to “0 time” when the drug was injected, as follows:
The concept of a single Vd
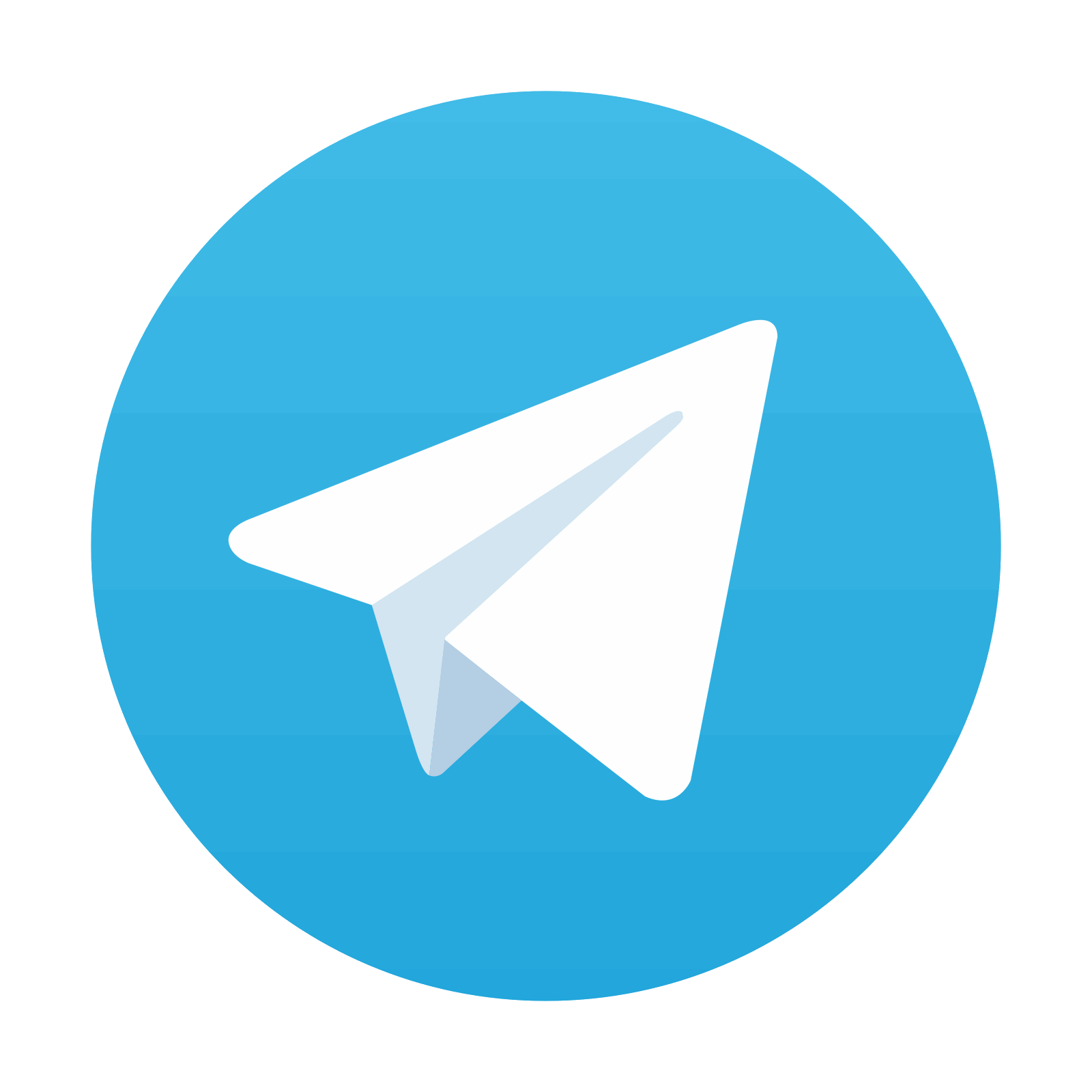
Stay updated, free articles. Join our Telegram channel

Full access? Get Clinical Tree
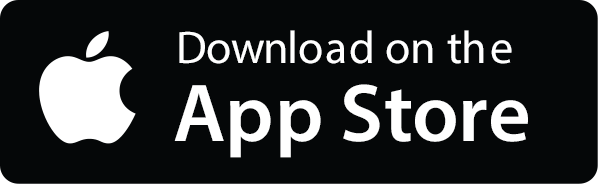
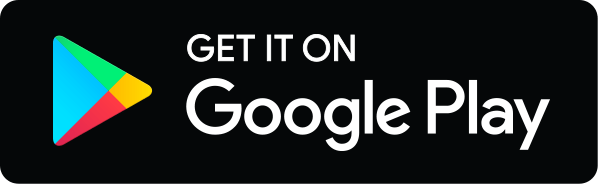
