Risk stratification
Procedure examples
Vascular (reported cardiac risk often more than 5%)
Aortic and other major vascular surgery
Peripheral vascular surgery
Intermediate (reported cardiac risk generally 1–5%)
Intraperitoneal and intrathoracic surgery
Carotid endarterectomy
Head and neck surgery
Orthopedic surgery
Prostate surgery
Lowb (reported cardiac risk generally less than 1%)
Endoscopic procedures
Superficial procedure
Cataract surgery
Breast surgery
Ambulatory surgery
To minimize operative risk, the patient is ideally in optimal medical condition. In the acute care setting, however, the luxury of an elective preoperative cardiac evaluation is not often available. A careful history and physical exam in the emergency setting can alert the surgeon to opportunities to intervene and physiologically optimize the patient in order to decrease the risk of morbidity and mortality. Congestive heart failure, poorly controlled hypertension (diastolic blood pressure >110 mmHg), electrolyte imbalances, and hyperglycemia must be addressed prior to any operative intervention when possible. In general, cardiovascular medications should be continued through the perioperative period, as continuation of antihypertensive and beta-blocker therapy throughout the perioperative period does not typically contribute to postoperative hemodynamic instability. Discontinuation of antihypertensive therapy increases potential risks. Rebound hypertension may be precipitated if centrally acting α (alpha)-2 agonists such as clonidine are suddenly withheld. Congestive heart failure may recur or be exacerbated if angiotensin-converting enzyme inhibitors or angiotensin receptor blockades are stopped. β (beta)-blockade must be continued throughout the perioperative time period.
Patients who experience postoperative MI after noncardiac surgery have an estimated hospital mortality rate of 15–25%. Patients who have a cardiac arrest after noncardiac surgery have a hospital mortality rate of 65% [4]. Of these major vascular complications, MI is the most common [5]. The diagnosis of acute MI in the nonsurgical setting traditionally requires the presence of at least two of the following three elements: ischemic chest pain, evolutionary changes on the electrocardiogram (ECG), and rise and fall of cardiac biomarker levels (troponin). In the perioperative period, ischemic episodes are often silent (not associated with a patient complaint of chest pain) in up to 65% of patients with documented postoperative MI [2]. Additionally, many perioperative ECGs reflect nonspecific changes, and are therefore nondiagnostic. These nonspecific ECG changes, coupled with new onset dysrhythmias and noncardiac related hemodynamic instability, can further obscure the clinical picture of MI or acute coronary syndrome in the perioperative period.
In the perioperative setting, as in the nonoperative setting, an acute increase in troponin levels should be considered an acute MI. An increase in cardiac troponin is a marker of myocardial injury, and there is a good correlation between the duration of myocardial ischemia and the increase in cardiac-specific troponin [6, 7]. There is also a significant association between increased troponin levels and short- and long-term morbidity and mortality in surgical patients. This association exists for cardiac death, MI, myocardial ischemia, congestive heart failure, cardiac dysrhythmias, and cerebrovascular accident. Even relatively minor cardiovascular complications such as uncontrolled hypertension, palpitations, increased fatigue, or shortness of breath are correlated to increased levels of cardiac-specific troponins. An increase in troponin postoperatively, even in the absence of clear cardiovascular signs and symptoms, is an important finding that requires careful attention and further investigation and management.
Multiple physiologic triggers that have the potential to exacerbate underlying cardiac disease exist in the perioperative period (Fig. 3.1). Surgery, with its associated trauma, anesthesia, narcotics, intubation, extubation, pain, hypothermia, bleeding, and anemia is analogous to an extreme stress test. These variables all initiate inflammatory, hypercoagulable, stress, and hypoxic states, which are associated with perioperative elevations in troponin levels, arterial thrombosis, and morbidity/mortality [8–11].
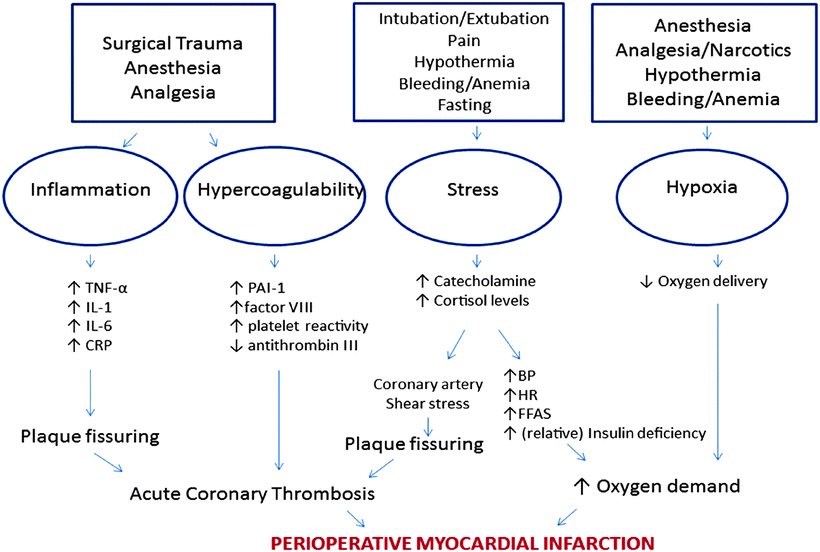
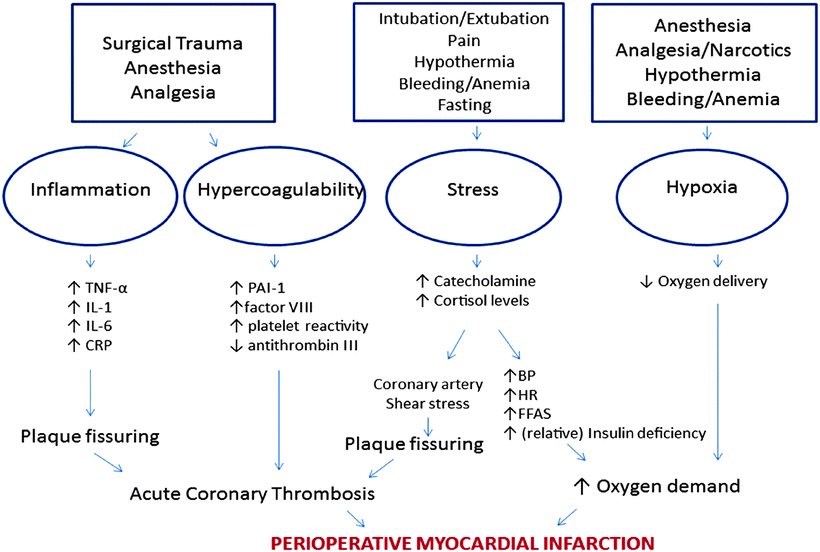
Fig. 3.1
Potential triggers of states associated with perioperative elevations in troponin levels, arterial thrombosis and fatal myocardial infarction. TNF-α (alpha) tumor necrosis factor α (alpha), IL interleukin, CRP C-reactive protein, PAI-1 plasminogen activator inhibitor-1, BP blood pressure, HR heart rate, FFAs free fatty acids. Adapted from [4]
Lee et al. derived and validated a “simple index” for the prediction of cardiac risk for stable patients undergoing major non cardiac surgery [12]. Although this study was done in the setting of nonurgent major noncardiac surgery, the following risk factors can and should be assessed in the setting of acute or emergent surgical intervention. Five independent risk correlates were identified:
1.
Ischemic heart disease (history of MI, history of positive treadmill test, use of nitroglycerin, current angina, or ECG with abnormal Q waves)
2.
Congestive heart failure (history of heart failure, pulmonary edema, paroxysmal nocturnal dyspnea, peripheral edema, bilateral rales)
3.
High-risk surgery (abdominal aortic aneurysm or other high-risk vascular, thoracic, abdominal, or orthopedic surgery—see Table 3.1)
4.
Preoperative insulin dependence for diabetes mellitus, and
5.
Preoperative creatinine greater than 2 mg/dL.
Increasing numbers of risk factors correlates with increased risk. This Revised Cardiac Risk Index has become one of the most widely used risk indices (Fig. 3.2).
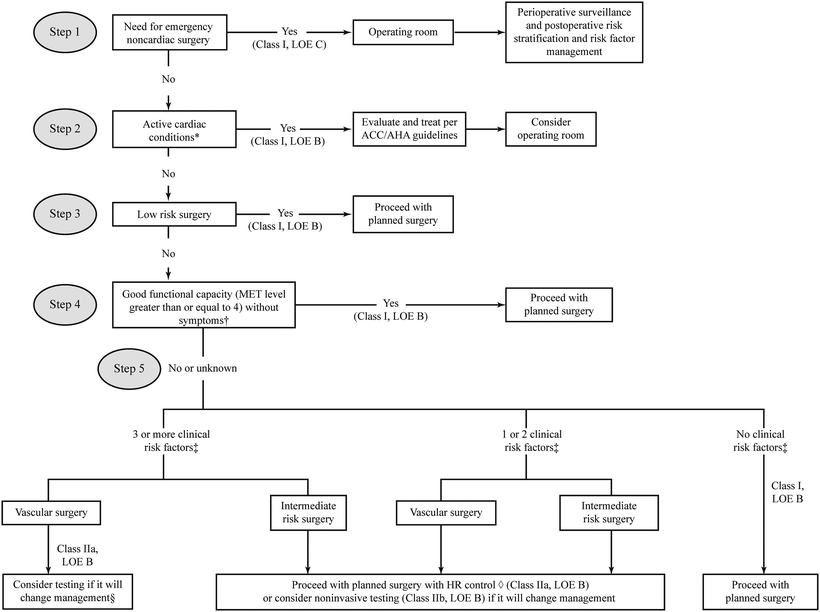
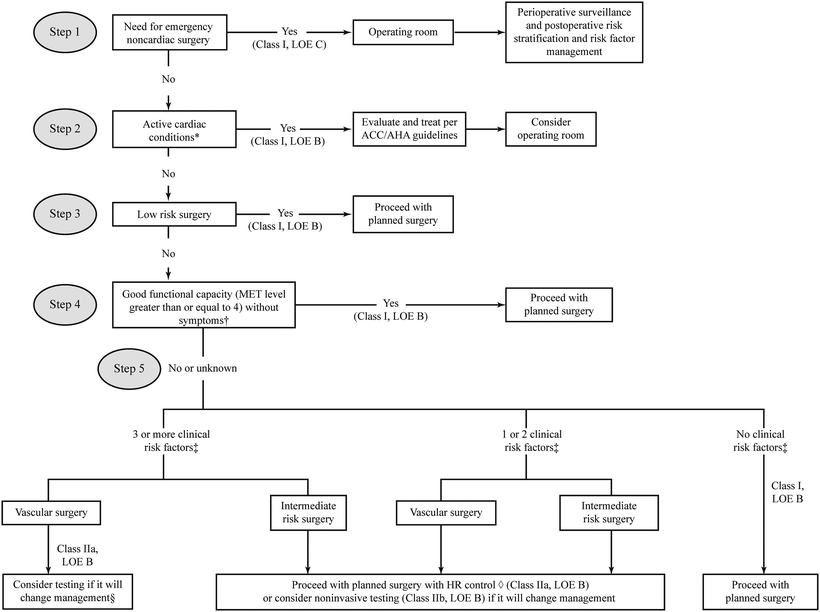
Fig. 3.2
Cardiac evaluation and care algorithm for noncardiac surgery. Asterisk Active clinical conditions: Unstable coronary syndromes, decompensated HF, significant arrhythmias, or severe valvular disease. Dagger Met 1 = Activities of Daily Living; Met 4 = heavy housework or climb a flight of stairs; Met 10 = strenuous exercise Section Noninvasive testing may be considered before surgery in specific patients with risk factors if it will change management. Double dagger Clinical risk factors include ischemic heart disease, compensated or prior heart failure, diabetes mellitus, renal insufficiency, and cerebrovascular disease. Open diamond Consider perioperative beta blockade. ACC/AHA American College of Cardiology/American Heart Association, HR heart rate, LOE level of evidence, MET metabolic equivalent. Modified with permission from Fleisher LA, Beckman JA, Brown KA, et al. ACC/AHA 2007 Guidelines on Perioperative Cardiovascular Evaluation and Care for Noncardiac Surgery: A Report of the American College of Cardiology/American Heart Association Task Force on Practice Guidelines. J Am Coll Cardiol. 2007;50(17): 1707–1732
Currently, there are no standard diagnostic criteria for perioperative MI in patients undergoing noncardiac surgery. The diagnosis of perioperative MI can be difficult as many are clinically silent, non transmural (non-Q-wave), and therefore have minimal accompanying ECG changes. On the basis of available literature, routine measurement of cardiac-specific troponin after surgery is more likely to identify patients without acute MI than with MI [13]. Additionally, studies of cardiac-specific troponin elevations neither consistently show associations with adverse cardiovascular outcomes at any time point nor provide insight into the effect of treatment on long-term or functional outcomes. Although it is known that patients with more extensive CAD are more likely to experience elevation in perioperative troponin levels, the role of revascularization in this population when no other manifestations of MI is unclear.
Additionally, revascularization in this setting may lead to postoperative complications such as bleeding due to initiation of antiplatelet therapy. Until the aforementioned issues are adequately studied and addressed, perioperative surveillance for acute coronary syndromes with routine ECG and cardiac serum biomarkers is unnecessary in clinically low-risk patients undergoing low risk procedures [14].
The presence of intraoperative and postoperative ST-segment changes has been associated with cardiac morbidity and mortality in high-risk patients undergoing noncardiac surgery. Numerous studies have demonstrated the limited ability of physicians to detect significant ST-segment changes compared with computerized analysis, and allows for trending of the data. Because the algorithms used to measure ST-segment shifts are proprietary, variability in accuracy between different monitors has been evaluated in several studies [15–17]. ST-segment trending monitors were found to have an average sensitivity of 74% and specificity of 73%, compared to offline Holter ECG recordings [16].
Retrospective data from multiple studies suggest that ST-segment depression is an independent predictor of perioperative cardiac events in high-risk noncardiac surgery patients. Changes of prolonged duration (greater than 30 min per episode or greater than 2 h cumulative duration) are particularly associated with increased risk [18–21]. Postoperative ST-segment changes, particularly of a prolonged duration, have been shown to predict worse long-term survival in high-risk patients [22, 23]. However, because intraoperative ST-segment changes may also be detected in the low-risk population, but are not associated with regional wall motion abnormality, ST-segment changes in this low-risk population may not be indicative of myocardial ischemia and CAD [24, 25]. Therefore, although there are data to support the use of ST-segment analysis to detect ischemia, no studies have yet addressed the issue of effect on patient outcome when therapy is based on these results alone. However, the general consensus of the American College of Cardiology (ACC) and the American Heart Association (AHA) is that early treatment, such as control of tachycardia, could lead to a reduction in cardiac morbidity [14].
Further evaluation regarding the optimal strategy for surveillance and diagnosis of perioperative MI is required. The current recommendations of the ACC/AHA, on the basis of current evidence, are for patients without documented coronary artery disease (CAD), surveillance should be restricted to those who develop perioperative signs of cardiovascular dysfunction. In patients with intermediate or high clinical risk who have known or suspected CAD and who are undergoing high- or intermediate-risk surgical procedures, the most cost effective strategy is to obtain a baseline (preoperative) ECG followed by an ECG in the immediate postoperative period and daily on the first and second postoperative day. If ECG changes are noted the use of cardiac-specific troponin measurements to supplement the diagnosis is warranted [15].
Once an intraoperative or postoperative MI has been correctly detected and diagnosed, it is important to recognize that the occurrence of a perioperative nonfatal MI carries a high risk for future cardiac events and cardiac related death [23, 26]. Patients who do sustain a perioperative MI should have evaluation of left ventricular function performed prior to hospital discharge, and standard post infarction medical therapy should be initiated. The use of pharmacological stress or dynamic exercise stress test should be obtained when feasible to assess risk stratification for possible coronary revascularization. In all cases, appropriate evaluation and management of complications and risk factors such as angina, heart failure, hypertension, hyperlipidemia, cigarette smoking, hyperglycemia or diabetes mellitus, and other cardiac abnormalities should occur prior to hospital discharge. Additionally, it is imperative to communicate these new observations and interventions to physician and nonphysician providers who will be responsible for the patient’s subsequent care and follow-up.
In summary, the basic clinical evaluation obtained by patient history, physical examination, and review of the ECG usually provides the surgeon with sufficient data to estimate cardiac risk. In each situation, the surgeon must determine the urgency of the proposed surgical procedure and balance this with the noted cardiac risk factors assessed in the history, physical exam, laboratory and radiographic data. In many instances, patient or surgery specific factors will dictate an obvious strategy (e.g., emergent surgery) that may not allow for further cardiac assessment or treatment. In these cases, the need for perioperative medical management and surveillance must be addressed. Selected postoperative risk stratification is often appropriate in patients with elevated coronary risk who have never undergone such assessment in the past. This should be initiated after the patient has recovered from any blood loss, deconditioning, and other postoperative complications that may confound interpretation of noninvasive test results, unless a perioperative MI has been diagnosed.
Preoperative Pulmonary Evaluation
Postoperative pulmonary complications (PPC) are equally prevalent as cardiac complications and contribute similarly to morbidity, mortality, and length of postoperative hospital stay. Late pulmonary complications are leading causes of morbidity and mortality after surgery, second only to cardiac complications. Pneumonia, respiratory failure, and atelectasis are the most commonly observed PPCs. However, multiple specific dysfunctions exist including laryngospasm, bronchospasm, airway obstructions, pulmonary embolism, reintubation or prolonged mechanical ventilation, pleural effusion, pneumothorax, and others.
PPCs are often multifactorial, and perioperative awareness and management of these factors can often help limit their occurrence. Procedural factors affecting pulmonary morbidity include upper abdominal and thoracic incisions, neurosurgical procedures, head and neck procedures, vascular procedures (particularly repair of abdominal aortic aneurysm), emergency operations, preoperative blood transfusion, use of nasogastric tubes, general anesthesia, and prolonged operative time (>3 h) [27–31]. Independent patient factors which contribute to postoperative pulmonary complications include older age (>60), severity of underlying pulmonary disease such as chronic obstructive pulmonary disease (COPD) or chronic bronchitis, American Society of Anesthesiologists (ASA) class II or greater (Table 3.2), functional dependence, alcohol abuse, cigarette smoking, and poor nutritional status (serum albumin <3.5 g/dL). Obesity and mild-to-moderate asthma have not been consistently shown to predict PPCs.
Table 3.2
American Society of Anesthesiologists (ASA) physical status classification
Classification | Description |
---|---|
1 | Normal healthy patient; no organic, biochemical, or psychiatric disease |
2 | Mild systemic disease with no functional limitation |
3 | Severe systemic disease with functional limitation |
4 | Severe systemic disease that is a constant threat to life |
5 | Moribund patient equally likely to die in next 24 h with or without operation |
6 | Brain dead patient; organs are being removed for donor purposes |
E | Emergency operation |
Until recently, no scoring systems existed for predicting PPCs similar to those used for cardiovascular risk stratification. Researchers with the Veterans’ Administration National Surgical Quality Improvement Project (NSQIP) have now developed and prospectively validated a scoring system for predicting postoperative pneumonia and respiratory failure [31]. This system includes many of the above predictors in a numeric scoring system (Tables 3.3 and 3.4). Table 3.3 displays the point values assigned to each preoperative predictor used to calculate the respiratory failure risk index score. Based on the predicted probability associated with various scores, the NSQIP researchers then categorized the patients into five risk classes. Table 3.4 displays the point values assigned to each preoperative predictor used in calculating the pneumonia risk index score. Table 3.5 displays the five risk classes with associated point values, and the predicted probability of postoperative respiratory failure or pneumonia.
Table 3.3
Respiratory Failure Risk Index
Preoperative predictor | Point value |
---|---|
Type of surgery | |
Abdominal aortic aneurysm Thoracic Neurosurgery Upper abdominal surgery Peripheral vascular Neck | 27 21 14 14 14 11 |
Emergency surgery | 11 |
Albumin <3.0 g/dL | 9 |
Blood urea nitrogen >3.0 mg/L | 8 |
Partially or fully dependent functional status | 7 |
History of chronic obstructive pulmonary disease | 6 |
Age (years) ≥70 | 6 |
Age (years) 60–69 | 4 |
Table 3.4
Risk index for postoperative pneumonia
Preoperative risk factor | Point value |
---|---|
Type of surgery | |
Abdominal aortic Thoracic Upper abdominal Neck Neurosurgery Vascular | 15 14 10 8 8 3 |
Age | |
>80 70–79 60–69 50–59 | 17 13 9 4 |
Functional status | |
Totally dependent Partially dependent | 10 6 |
Weight loss >10% in past 6 months | 7 |
History of chronic obstructive pulmonary disease | 5 |
General anesthesia | 4 |
Impaired sensorium | 4 |
History of cerebrovascular accident | 4 |
Blood urea nitrogen level | |
<8 mg/dL 22–30 mg/dL >30 mg/dL | 4 2 3 |
Transfusion >4 units | 3 |
Emergency surgery | 3 |
Steroid use for chronic condition | 3 |
Current smoker within 1 year | 3 |
Alcohol intake >two drinks/day in past 2 weeks | 2 |
Table 3.5
Risk categories for respiratory failure and pneumonia
Class | Postoperative respiratory failure risk index (point total) | Probability of respiratory failure (%) | Postoperative pneumonia risk index (point total) | Probability of pneumonia (%) |
---|---|---|---|---|
1 | 0–10 | 0.5 | 0–15 | 0.2 |
2 | 11–19 | 2.2 | 16–25 | 1.2 |
3 | 20–27 | 5.0 | 26–40 | 4.0 |
4 | 28–40 | 11.6 | 41–55 | 9.4 |
5 | >40 | 30.5 | >55 | 15.3 |
Decreases in functional residual capacity (FRC) and forced vital capacity (FVC) in the postoperative period can lead to atelectasis, decreased pulmonary compliance, increased work of breathing and tachypnea with low tidal volumes. Poor cough effort due to pain and impaired airway reflexes increase susceptibility to retained secretions, bacterial invasion and pneumonia. Aspiration of contaminated oropharyngeal secretions is thought to be a prominent mechanism leading to nosocomial and postoperative pneumonia [32]. Aspiration may occur during intubation, but undetected aspiration is probably frequent after surgery. Prolonged endotracheal intubation predisposes to aspiration of oropharyngeal material and puts the patient at risk for ventilator-associated pneumonia, a complication that doubles the risk for mortality [33]. Oropharyngeal and laryngeal protective mechanisms can be transiently decreased after surgery and may also predispose the nonintubated patient to pneumonia. Nasogastric intubation is likely to decrease airway protective mechanisms and predispose to occult aspiration. Residual subclinical muscle relaxation has been detected in patients who received long-acting muscle relaxants, and it was associated with an increased rate of pulmonary complications [34].
Perioperative fluid management and resuscitation in the acute surgical patient can impact cardiopulmonary function and may lead to PPCs. Because the acute surgical patient often presents in shock, overly aggressive resuscitation in the perioperative time frame may contribute to Acute Lung Injury (ALI) and PPCs. Large volume crystalloid resuscitation, particularly delayed resuscitation, may lead to undesirable extravascular pulmonary volume. Judicious resuscitation and ongoing clinical assessment by both the surgeon and anesthesia team is essential to reduce or minimize perioperative ALI.
The anesthetic and intraoperative ventilator strategy can influence the extent and course of perioperative lung injury. Kirkpatrick and Slinger recently examined the effects of perioperative mechanical ventilation, intraoperative lung protective ventilator strategies and their role in ventilator-induced lung injury [35]. The phenomenon of ventilator-induced lung injury (VILI) is well recognized. VILI involves a complex interaction of volutrauma, barotrauma, cyclic opening and closing of the alveoli (atelectotrauma), and inflammatory mediators (biotrauma). Although a degree of lung stretch is important for surfactant production, shear stress induces pro-inflammatory cytokines in endothelial, epithelial, and macrophage cells [35].
Atelectasis also plays a role in ALI. Atelectasis occurs frequently after open surgical procedures and in up to 90% of patients undergoing general anesthesia [36]. It is a pathologic state that has direct and indirect effects on the development or exacerbation of ALI. There is concern that the lower tidal volumes associated with lung protective ventilator strategies may predispose the lung to atelectasis and subsequent ALI. Unfortunately, there are conflicting data on the influence of tidal volume on atelectasis and recruitment [37, 38]. It is clear that the techniques to avoid or treat atelectasis, including recruitment maneuvers and appropriate application of positive end expiratory pressure (PEEP), are effective in the setting of low tidal volumes. Therefore despite the lack of randomized controlled trials thus far to optimally define appropriate intraoperative tidal volume, PEEP and the use of intraoperative lung recruitment, it is reasonable to apply protective ventilator strategies in the intraoperative period based on our current understanding of mechanical ventilation and ALI.
Intraoperative factors may significantly affect the risk for PPCs. There is some evidence that suggests performing laparoscopic surgery rather than open abdominal surgery is associated with decreased pulmonary complications [39, 40], similar to endovascular interventions as opposed to open procedures [41]. The duration of anesthesia and of the surgery is probably one of the strongest predictors of PPCs. This association has been detected by more than one study [42–44]; however, it is not clear whether the duration or the complexity and type of the procedure itself is the cause of PPCs. Regarding to anesthetic technique, conflicting data exist. In the NSQIP studies, general anesthesia was associated with higher risk for respiratory failure and pneumonia [31]. In other studies, however, the use of general anesthesia had no correlation with risk for PPCs [27, 45]. The assessment of the type of anesthesia as a risk factor for PPCs through retrospective or observational studies is difficult in that the effect of anesthesia is not easily distinguishable from the effect of the site or complexity of the surgery itself. Additionally, general anesthesia is more frequently used in surgeries already at increased risk for PPCs, such as thoracoabdominal procedures, and relatively less frequently selected for lower risk or extremity procedures.
The use of a perioperative gastric tube is another important risk factor for the development of PPCs. Several studies have reported that the perioperative use of nasogastric tubes is an independent predictor of pulmonary complications [27, 28, 46]. This correlation has been confirmed by multivariate analysis, which suggests that gastric suctioning itself, and not simply the use of a nasogastric tube in higher risk procedures, causes the pulmonary complications. The mechanism is likely related to decreased airway protection and aspiration of pharyngeal secretions.
Preoperative pulmonary testing is only useful if it provides data that cannot be obtained from the history and physical examination, and if it helps determine the probability of a complication in patients who are known to have risk factors. Several studies have shown that pulmonary function tests (PFTs) results have no significant correlation with PPCs [28, 47]. Other studies also suggest that pulmonary and nonpulmonary data collected through clinical evaluation contain most of the information necessary to make a risk prediction. The implication of these results is that no useful information is added by routinely performing PFTs as part of the clinical evaluation of patients undergoing nonthoracic surgery.
Arterial blood gas analysis has been used in the past for the preoperative evaluation of nonthoracic surgery patients despite the lack of evidence supporting its value. Hypercapnia with PaCO2 (partial pressure of carbon dioxide in arterial blood) greater than 45 mmHg and arterial hypoxemia (PO2 <60 mmHg) have in the past been considered important risk factors for PPCs and contraindications for surgery. These blood gas alterations may help in certain risk–benefit assessments for a given patient or procedure, as their presence is associated with a decreased life expectancy in patients with COPD. However, neither hypercapnia nor hypoxemia has been shown to be independent predictors of the risk for PPCs [27, 45].
Chest radiographs are still routinely performed preoperatively in older patients, in patients with known pulmonary disease, and in patients who smoke; however, there is little to no evidence that routine chest radiographs affect the perioperative management or outcomes in any way [48]. A reasonable use of chest radiology may be for patients with new or unexplained pulmonary symptoms, or for those with an acute process such as pneumonia or pneumothorax that will alter operative decision making and/or timing of surgery in the acute setting. Chest radiographs in asymptomatic patient are unlikely to add any information to the pulmonary risk stratification [48].
In summary, when assessing for pulmonary risk in the acute surgery setting, a thorough history and physical examination is essential, with particular attention to pulmonary and nonpulmonary factors that have been shown to be independent predictors of PPCs. Table 3.6 summarizes the aforementioned data and outlines risk reduction strategies for the prevention of PPCs. For patients with stable pulmonary symptoms who are undergoing a high-risk procedure (upper abdominal or thoracic surgery, major vascular surgery, duration longer than 3 h or the use of gastric suctioning), a further attempt at risk stratification using the postoperative pneumonia and respiratory failure risk indexes (Tables 3.3 and 3.4) can be performed. Those patients with a high score and therefore a high probability of PPCs can therefore be identified (Table 3.5), and the information used to guide not only preoperative counseling and consent but also in the implementation of risk-reduction strategies (Table 3.6), alternative surgical approaches, damage control procedure, or possibly nonoperative management. In patients with recent or ongoing respiratory infections, delay of surgery or nonsurgical management should be considered, if acceptable. Chest radiography with or without arterial blood gas measurement may be helpful in the acute setting to determine the presence, absence or severity of any active pulmonary disease or infection.
Table 3.6
Evidence-based strategies to reduce the risk of PPCs
Factor | Clinical strategies | Evidence grade |
---|---|---|
Demonstrated benefit Lung expansion modalities | Incentive spirometry, chest physiotherapy, continuous positive airway pressure | A |
Probable benefit Selective nasogastric decompression Shorter acting neuromuscular blockade | Use nasogastric tube only for postoperative nausea/vomiting, abdominal distention Use of vecuronium or atracurium vs. pancuronium | B B |
Possible benefit Laparoscopic vs. open operation | Choice of less invasive surgical approach | C |
Uncertain benefit Smoking cessationa Intraoperative regional block Postoperative epidural analgesia Immunonutrition | Long term vs. short term (48 h) Spinal or Epidural block Overall infections decreased; no data for PPCs | I I I I |
No benefit Pulmonary Artery Catheterization Routine use of parenteral nutrition Routine use of enteral nutrition | ? Exception for severely malnourished or prolonged decrease enteral intake Overall infections and complications were lower however no difference in PPCs | D Db Db |
Prophylaxis of Venous Thromboembolism
The morbidity and mortality of venous thromboembolism (VTE) makes consideration of prophylaxis mandatory for every major operation. In the acute care setting, multiple factors must be considered with regards to method and timing of initiation of chemical prophylaxis. Knowledge of risk factors for VTE in certain patient groups or in individual patients allows the most appropriate and cost effective use of prophylaxis. Multiple factors have been identified, including increasing age, prolonged immobility, obesity, prior VTE or history of pulmonary embolism, varicose veins, cancer, inflammatory bowel disease, nephrotic syndrome, pregnancy or estrogen use, indwelling central venous catheters, certain surgical procedures (in particular operations involving the abdomen, pelvis, or lower extremities), and trauma (especially pelvis or lower extremity fractures) [49–51]. These risk factors are often present in combination in hospitalized patients, and the risks are cumulative.
The 8th edition of the American College of Chest Physician (ACCP) guidelines (2008) recommends that every hospital develop a formal and active strategy to consistently identify medical and surgical patients at risk for VTE and to prevent VTE occurrence [52]. The Surgical Care Improvement Project (SCIP), a national partnership of organizations including the American Medical Association and the American College of Surgeons has been tasked with the goal to reduce surgical complications in the United States by 25% from 2005 to 2010. Two SCIP process measures have been developed in relation to improving VTE prophylaxis: (1) The proportion of surgical patients for whom recommended VTE prophylaxis is ordered, and (2) the proportion of surgical patients who actually receive appropriate VTE prophylaxis within 24 hours before or after surgery [53].
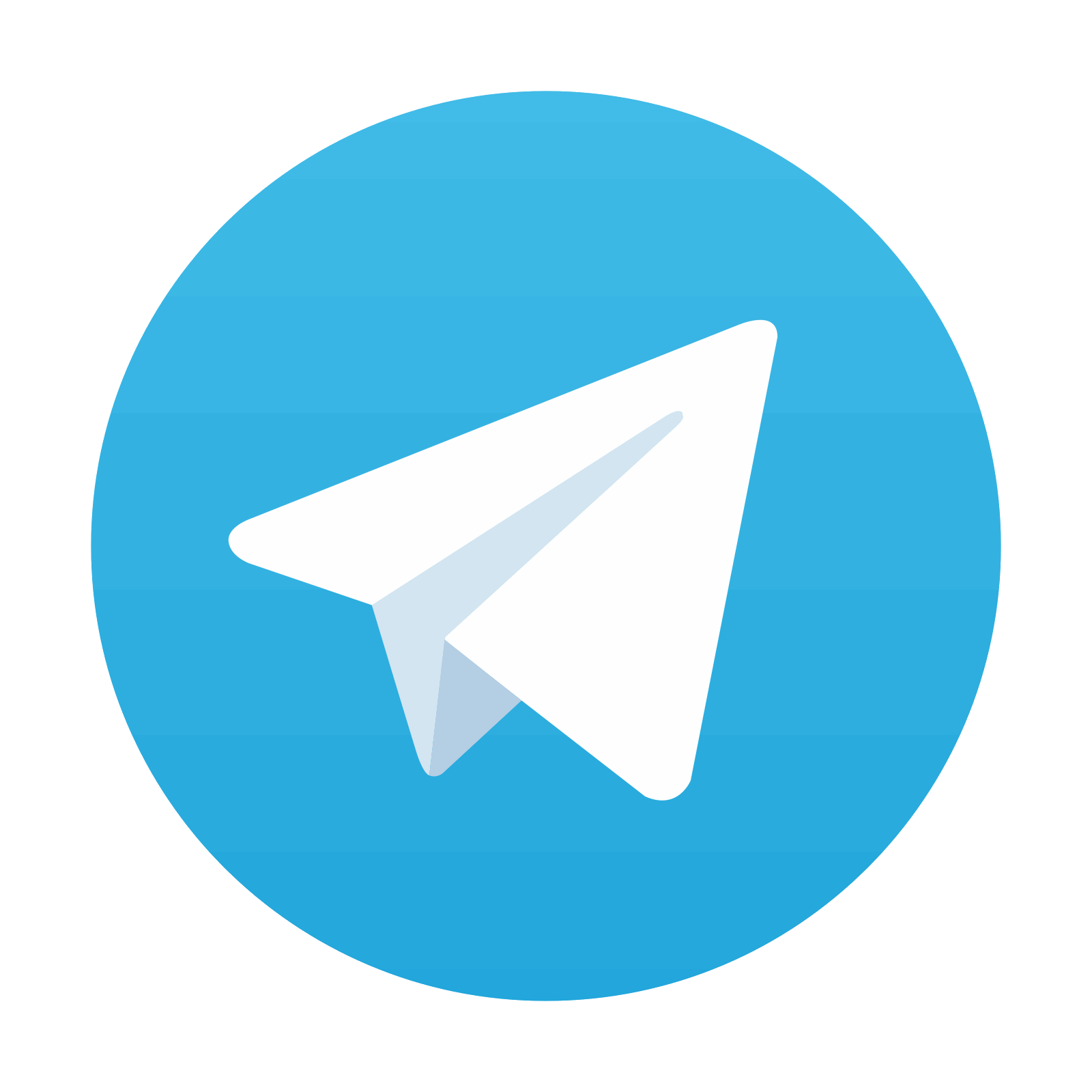
Stay updated, free articles. Join our Telegram channel

Full access? Get Clinical Tree
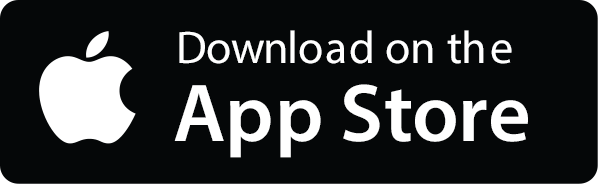
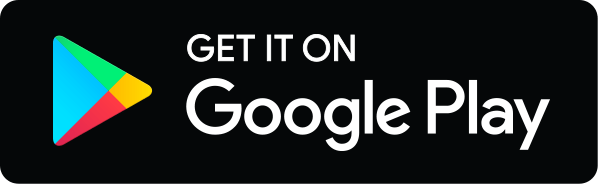