Chapter 10 In absolute terms, cardiac arrest is not rare in pediatric patients; it occurs in 2 to 6% of children admitted to a pediatric intensive care unit (PICU) and about 16,000 children per year out of hospital in the United States (i.e., 8-20 per 100,000 children per year).1–4 From the perspective of the individual care provider in the prehospital or inpatient medical setting outside the intensive care unit (ICU), however, pediatric resuscitations constitute an infrequent occurrence.5–10 Significant disparity in favorable outcomes continues to exist between out-of-hospital and in-hospital pediatric cardiac arrest. Data from the National Heart, Lung, and Blood Institute (NHLBI)–funded Resuscitation Outcomes Consortium demonstrated an overall population-based incidence of nontraumatic pediatric out-of-hospital cardiac arrests (OOHCAs) of 8 per 100,000 pediatric person-years compared with 125 per 100,000 adult person-years; however, the rate among infants (73 per 100,000) was on the same order of magnitude as in adults. More important, pediatric patients were more likely to survive to discharge than adults (6.4% vs. 4.5%). Specifically, children and adolescents were twice as likely to survive to hospital discharge as infants and adults. The number needed to treat (NNT) of 13 to save a pediatric life compares favorably with NNT for other aggressive interventions considered quite effective, such as implantation of a cardioverter-defibrillator in patients with ventricular arrhythmias (NNT of 8) and immediate revascularization in patients with cardiogenic shock (NNT of 13). Furthermore, the potential years of life gained for pediatric survivors are much greater than for adult survivors.11 Among published studies of out-of-hospital pediatric cardiac arrest, the most common causes are sudden infant death syndrome (SIDS; 20-23%), trauma (15-20%), and submersion injury (8-12%).4,12 Prognosis after cardiac arrest varies considerably based on the underlying cause, with outcomes after SIDS and trauma being particularly dismal13–15 and outcomes after submersion including a higher prevalence of survival.3,16,17 Table 10-1 shows the prevalence of survival to discharge after out-of-hospital and in-hospital pediatric cardiac arrest as reported by multiple studies over the past three decades.4,11,18–22 It is noteworthy that survival after in-hospital cardiac arrest has improved substantially from less than 10% in the 1980s to greater than 25% in the first decade of the 21st century. By contrast, survival after OOHCA has changed very little in 30 years, with survival less than 10% in virtually all studies over that time period.4,11,12,23,24 The American Heart Association (AHA) National Registry of Cardiopulmonary Resuscitation (Get With The Guidelines—Resuscitation [GWTG-R], formerly known as NRCPR) database has yielded analyses demonstrating specific epidemiologic features of in-hospital pediatric arrest associated with improved survival, including young age and events occurring in hospitals with higher levels of pediatric staffing.25,26 Table 10-1 Outcomes after Pediatric Cardiac Arrest NR, data not reported; ROSC, return of spontaneous circulation. Cardiac arrest occurring in the emergency department (ED) (as distinct from out-of-hospital arrest with continued resuscitation in the ED) accounts for a minority (approximately 11-13%) of in-hospital events for children and adults.19,25,27 Historical outcomes for children who arrest while in the ED suggest that survival outcomes are less prevalent than in cases of in-hospital arrest but better than in out-of-hospital arrest.28 More recent controlled analyses from the GWTG-R demonstrate a significant discrepancy between outcomes among children who arrest in the ED, with an adjusted odds ratio of 0.39 for survival compared with other in-hospital locations, highlighting differences in pathophysiology of ED versus in-hospital cardiac arrest in children.25 These data stand in stark contrast to data in adults, in whom arrests in the ED were associated with significantly improved survival of 22% compared with 10-15% for events in the ICU or wards.29 Three common pathways to arrest have been identified: asphyxial, ischemic, and arrhythmogenic. Asphyxial cardiac arrests are precipitated by acute hypoxia or hypercarbia and are the most common in children.4,19,30 Ischemic arrests are precipitated by inadequate myocardial blood flow, in children most commonly as a result of shock from hypovolemia, sepsis, or myocardial dysfunction. Arrhythmogenic arrests are most often sudden, precipitated by ventricular fibrillation (VF) or ventricular tachycardia (VT). The immediate causes of arrest in two recent in-hospital studies were arrhythmogenic in 10%, asphyxial in 67%, and ischemic in 61% (many patients had both asphyxia and ischemia).19,30 The vast majority of out-of-hospital arrests are also either asphyxial or ischemic, and 5 to 20% are arrhythmogenic.3 The use of closed-chest cardiac massage to provide adequate circulation during cardiac arrest was initially demonstrated in small dogs with compliant chest walls.31 Therefore children were among the first patients successfully treated with this technique. The presumed mechanism of blood flow was direct compression of the heart between the sternum and the spine in children with compliant chest walls. Later investigations indicated that blood could also be circulated during CPR by the thoracic pump mechanism. That is, chest compression–induced increases in intrathoracic pressure can generate a gradient for blood to flow from the pulmonary vasculature, through the heart, and into the peripheral circulation.32,33 The triad of pulselessness, apnea, and unresponsiveness defines the clinical state of cardiac arrest. For decades, published guidelines by the International Liaison Committee on Resuscitation (ILCOR) on the assessment of patients suspected to be in cardiac arrest used the mnemonic ABC (airway, breathing, circulation) for the stepwise assessment and intervention sequence. This sequence included opening the airway, assessing respirations with the “look-listen-feel” technique for up to 10 seconds, providing two rescue breaths, and checking for a central pulse (brachial or femoral in children, carotid in adults). For the first time, in 2005, treatment recommendations published by ILCOR removed the pulse check as a necessary step for lay rescuers, based on data demonstrating poor specificity and sensitivity of a 10-second carotid pulse check by health care providers in anesthetized adults undergoing aortic cross-clamping.34,35 The implication for rescuers is that any patient who is unresponsive and apneic and appears hypoperfused by gross appearance (i.e., “appears dead”) should have chest compressions initiated immediately without a check for a pulse. This recommendation may have particular pertinence for children, given the high prevalence of bradycardia and hypoperfusion in the prearrest phase and the potential improvement in outcomes when CPR is provided for bradycardia (see later). Studies on the pulse check in pediatrics have predominantly focused on healthy children, where the data on the accuracy of brachial and femoral pulse checks are varied.36,37 A recent pediatric ICU study examined the accuracy of the pulse check by health care providers on children receiving extracorporeal circulatory support (i.e., either extracorporeal membrane oxygenation [ECMO] or a ventricular assist device [VAD]) in whom native pulsatile cardiac activity was variably diminished or absent; it was found that a 10-second femoral or brachial pulse check had a sensitivity of 86% and a specificity of 64%.38 In other words, making a decision to provide CPR based on the pulse check alone in this patient set would have resulted in chest compressions being given to 36% of patients in whom they were not indicated and compressions being withheld from 14% of children who were either pulseless or critically hypoperfused enough to require them. Based largely on this study, the 2010 ILCOR guidelines for pediatric resuscitation have removed the pulse check for lay rescuers. Health care providers may spend up to 10 seconds assessing a central pulse, but the empirical provision of chest compressions without a pulse check for the child who appears dead is appropriate. Prearrest Phase.: Because most out-of-hospital pediatric cardiac arrests are caused by progressive asphyxia or ischemia, they can often be prevented by avoiding the precipitating insult. For example, infant and child car seats and seat belts for older children can prevent cardiac arrests resulting from motor vehicle collisions. Similarly, fences around swimming pools with self-closing gates can prevent drowning. Both the BRESUS study in the United Kingdom and the AHA’s GWTG-R national registry data clearly demonstrate that most in-hospital cardiac arrests are asphyxial or ischemic rather than sudden arrhythmia-induced events.19,27,39 Most important, many of these arrests could be prevented by early recognition and treatment of respiratory failure and shock. These issues were appreciated by the founders of the Pediatric Advanced Life Support (PALS) course, which was therefore designed to prevent cardiac arrests by early recognition and treatment of respiratory failure and shock in children.40 No-Flow Phase (Untreated Cardiac Arrest).: Interventions during the no-flow phase of untreated pulseless cardiac arrest focus on early recognition of cardiac arrest and initiation of basic and advanced life support. Yet only a third of children with OOHCA are provided with bystander CPR. Low-Flow Phase (Resuscitation).: During untreated cardiac arrest, circulation has stopped (i.e., the no-flow phase). The low-flow phase begins once resuscitation measures (chest compressions) are implemented to generate blood flow. Specific considerations related to chest compressions and advanced life support medications are discussed later. Postresuscitation Phase.: The postarrest syndrome is a unique and complex combination of pathophysiologic processes that occurs after successful resuscitation. This postarrest syndrome includes (1) postarrest brain injury, (2) postarrest myocardial dysfunction, (3) systemic ischemia-reperfusion response, and (4) the unresolved pathologic process that caused the cardiac arrest. Clinical manifestations of postarrest brain injury include coma, seizures, myoclonus, varying degrees of neurocognitive dysfunction (ranging from memory deficits to persistent vegetative state), and brain death. Mild induced hypothermia is the best-established postresuscitation therapy for adult postarrest brain injury. Two seminal articles established that induced hypothermia (32-34° C) could improve outcome for comatose adults after resuscitation from VF cardiac arrest.41,42 In both randomized controlled trials, the inclusion criteria included patients older than 18 years who were persistently comatose after successful resuscitation from nontraumatic VF. Interpretation and extrapolation of these studies to children are difficult. Hyperthermia after resuscitation from cardiac arrest is common in children because of inflammatory mediators.43 It is reasonable to believe that mild induced systemic hypothermia may benefit children resuscitated from nontraumatic cardiac arrest. However, benefit from this treatment has not been rigorously studied and reported in children or in any patients with non-VF arrests. Multicenter trials of induced hypothermia in children after both in-hospital cardiac arrest and traumatic arrest are ongoing. Emerging neonatal trials of selective brain cooling and whole-body cooling show improved outcomes for this therapy in neonatal hypoxic-ischemic encephalopathy.44,44A Postarrest myocardial dysfunction and hypotensive shock are very common among pediatric survivors of cardiac arrest. It is interesting to note that postarrest myocardial dysfunction appears to be pathophysiologically similar to sepsis-related myocardial dysfunction, including increases in inflammatory mediator and nitric oxide production.45–47 Although the optimal management of postarrest hypotension and myocardial dysfunction have not been defined, data suggest that aggressive hemodynamic support may improve outcomes. Controlled trials in animal models have shown that dobutamine, milrinone, or levosimendan can effectively ameliorate postarrest myocardial dysfunction.48–51 In clinical observational studies, fluid resuscitation has been provided for pediatric patients with hypotension and concomitant low central venous pressure, and various vasoactive infusions, including epinephrine, dobutamine, and dopamine, have been provided for the myocardial dysfunction.* Adequate myocardial blood flow is necessary for return of spontaneous circulation. During CPR, myocardial blood flow depends on coronary perfusion pressure or the “driving pressure” of blood into the coronary arteries from the aorta (i.e., the difference between the aortic and right atrial pressures during the relaxation phase). If the coronary perfusion pressure falls below 15 mm Hg during CPR in adults, the likelihood for a return of spontaneous circulation is substantially decreased.54 Animal data suggest that outcomes improve as coronary perfusion pressure increases above 25 mm Hg.55 Moreover, even relatively brief interruptions to chest compressions (e.g., 4-second pauses for two rescue breaths) lead to substantial decreases in the aortic relaxation pressure and coronary perfusion pressure, thereby resulting in inadequate myocardial perfusion56,57 (Fig. 10-1). In animal models of cardiac arrest, circumferential CPR (e.g., vest or load-distributing band CPR, encircling hands and compression with two thumbs) provides better CPR hemodynamics than focal compressions (e.g., hands on chest, two-finger technique). In smaller infants, the recommended CPR technique for two rescuers is to encircle the chest with both hands and depress the sternum with the thumbs while compressing the thorax circumferentially (when the resuscitator’s hands are relatively large enough to do so58 (Fig. 10-2). This “two-thumb” circumferential compression technique results in higher systolic and diastolic blood pressures and a higher pulse pressure than traditional two-finger compression of the sternum.59 Although the optimal chest compression rate is unknown, data from large studies of animals have shown that coronary perfusion pressure, CO, and survival are substantially superior with chest compression rates of 100 per minute compared with rates of less than 80 per minute.28,29 In addition, clinical studies in adults have shown that end-tidal carbon dioxide levels, a marker of cardiac output during CPR, were significantly higher with chest compressions at 120 versus 80 per minute.60 Although restoration of coronary perfusion during CPR is critical for successful return of spontaneous circulation, adequate cerebral perfusion is important for mitigating the injurious effects of cerebral anoxia during cardiac arrest. Unlike myocardial blood flow, cerebral blood flow is generated during the compression phase of CPR. Forceful, uninterrupted chest compressions at a rate of 120 per minute compared with 60 per minute improves both cerebral and coronary perfusion pressures compared with less forceful compressions or compressions at a lower rate.61,62 In addition, vasoconstrictors, such as epinephrine or vasopressin, preferentially direct the CO during CPR to the coronary and cerebral circulations. Despite evidence-based guidelines, extensive provider training, and provider credentialing in resuscitation medicine, the quality of CPR is typically poor. Slow compression rates, inadequate depth of compression, and substantial pauses are the norm.63–65 Moreover, observed ventilation rates during professional rescuer CPR are often too high, potentially leading to deleterious effects on venous return and outcome.66 The resuscitation mantra is Push hard, push fast, minimize interruptions, allow full chest recoil, and do not overventilate. This approach can markedly improve myocardial, cerebral, and systemic perfusion and will likely improve outcomes.67 Ideal compression-to-ventilation ratios for pediatric patients are unknown. Physiologic estimates suggest that the amount of ventilation needed during CPR is much less than the amount needed during a normal perfusing rhythm because the CO (and therefore pulmonary blood flow) during CPR is only 10 to 25% of that during normal sinus rhythm.68 The best ratio of compressions to ventilations depends on many factors, including the compression rate, the tidal volume, the blood flow generated by compressions, and the time that compressions are interrupted to perform ventilations. In a manikin model of pediatric CPR, a chest compression-to-ventilation (CC:V) ratio of 15 : 2 delivered the same minute ventilation as CPR with a CC:V ratio of 5 : 1, but the number of chest compressions delivered was 48% higher with the 15 : 2 ratio.69,70 The benefits of positive pressure ventilation (increased arterial oxygen content and carbon dioxide elimination) are balanced against the adverse consequence of impeding circulation because of increases in intrathoracic pressure and venous return to the heart. For adults, mathematical models of oxygen delivery during CPR suggest that the optimal CC:V ratio is approximately 30 : 2 for two-rescuer health care provider CPR and is closer to 50 : 2 for single-lay rescuers.71 Similar mathematical models adjusted to the known physiologic variables in children indicate that CC:V ratios from 10 : 2 to 30 : 2 would be reasonable to optimize tissue oxygen delivery during CPR.72 The present recommendations for CC : V ratios during CPR are based on rational conjecture from animal, manikin, and mathematical models, as well as educational theory on the retention of skills in adult learners. In the 2010 AHA guidelines, a universal CC:V ratio of 30 : 2 is recommended for single-person bystander CPR. For two-rescuer CPR, a 15 : 2 ratio is recommended for neonates, infants, and children beyond the newly born (infant at the time of birth) period. For the newly born, a ratio of 3 : 1 is recommended, resulting in a greater number of ventilations per minute, but nearly the same number of compressions (100 vs. 90 per minute).58 This recommended ratio was arrived at by consensus to balance educational issues (i.e., the benefit to single-rescuer bystanders of remembering only one compression-to-ventilation ratio of 30 : 2) with what is known about the physiology of the cardiac and pulmonary circulations of children during cardiac arrest. In a large observational study of OOHCAs, incomplete release or “leaning” occurred in more than 10% of compressions and was observed in 16 of 173 (9%) CPR episodes.73,74 Observations during in-hospital pediatric CPR indicate that leaning is a common phenomenon, occurring in 23% of chest compressions.75 Leaning pressures of approximately 15% of body weight may affect intrathoracic pressure and impede the hemodynamics of CPR.75,76 In an effort to optimize CPR quality, new technology has been developed that monitors CPR through a force sensor and accelerometer on the chest. This information is transmitted to a defibrillator monitor to provide quantitative verbal feedback to the rescuer on the rate and force of compressions as well as the frequency and volume of ventilations. Recent studies document that poor-quality CPR, as analyzed by a feedback device, reduces the likelihood of defibrillation success,74 and rescuers can use this type of automated feedback to improve CPR quality and compliance with current guidelines.77 The optimal goals for aortic pressures during pediatric CPR are unknown. Animal data and adult data suggest that a reasonable goal for the aortic diastolic (or relaxation) pressure is greater than 20 to 30 mm Hg.54,55 Similarly, a reasonable goal for the aortic systolic (or compression) pressure is greater than 50 mm Hg for a newborn, 60 mm Hg for an infant, 70 to 80 mm Hg for a child, and 80 to 90 mm Hg for an adolescent. Multiple studies in adults with OOHCA have demonstrated that chest compressions alone (“hands-only” CPR) yielded survival outcomes that were as good if not better than standard CPR with ventilations included.78–80 Not surprising, animal studies of bystander CPR for asphyxia-precipitated cardiac arrests demonstrate that the addition of rescue breathing to compressions results in much better outcomes than chest compressions alone.81,82 Chest compressions alone, however, were superior to no CPR at all, even with hypoxia-induced cardiac arrest. These studies support the need for rescue breathing as a critical component of CPR for pediatric asphyxia-precipitated cardiac arrests. A large population-based study in Japan found that children with OOHCA of noncardiac origin (i.e., asphyxial or ischemic) had significantly improved survival when standard bystander CPR (with ventilation included) was provided when compared with compression-only CPR. This survival benefit, however, was not found to be present among children whose OOHCA was of primary cardiac origin.83 Thus, compression-only CPR is not recommended for the majority of pediatric cardiac arrests. For an older child with a sudden collapse from cardiac arrest (i.e., presumed VF or VT), compression-only CPR is a reasonable choice for bystander CPR. Figure 10-3 demonstrates a simplified algorithm for pediatric pulseless cardiac arrest. Table 10-3 lists medications commonly used during pediatric resuscitation including dosages and indications. Prospective and retrospective studies indicate that use of high-dose epinephrine in adults or children (0.05-0.2 mg/kg) does not improve survival and may be associated with a worse neurologic outcome.66–69 A randomized, blinded, controlled trial of rescue high-dose epinephrine versus standard-dose epinephrine after failed initial standard-dose epinephrine for pediatric in-hospital cardiac arrest demonstrated a worse 24-hour survival rate in the high-dose epinephrine group.84 High-dose epinephrine cannot be recommended for routine use during CPR. Calcium salts (calcium gluconate, calcium chloride) are commonly used in pediatric resuscitation for sepsis, transfusion-associated ionized hypocalcemia, specific toxidromes, and in newborns after cardiac surgery. Data on calcium salts in cardiac arrest, however, do not support its routine use. A controlled analysis of the GWTG-R database demonstrated significantly decreased survival to hospital discharge among children receiving calcium salts during CPR.85 Current recommendations for calcium salts during CPR are limited to cases of documented hypocalcemia, hyperkalemia, hypermagnesemia, or known or suspected intoxication with calcium channel blockers.86 Although asystole and pulseless electrical activity (PEA) are the most common rhythms seen with in-hospital pediatric cardiac arrest, VF and pulseless VT are not rare.19 VF/VT may occur as the primary inciting arrest rhythm (i.e., arrhythmogenic arrest), arising from a variety of underlying myocardial pathologies (e.g., acute infectious cardiomyopathies, congenital heart disease, Wolff-Parkinson-White syndrome, channelopathies, or electrolyte derangements. Of 1005 pediatric in-hospital cardiac arrests in the GWTG-R database, 25% of the patients had VF/VT at some point during the resuscitation, 10% as an initial rhythm and an additional 15% as subsequent VF/VT (i.e., some time later during the resuscitation effort).30 Asphyxia-associated VF (presumably subsequent VF) is also well documented in pediatric drowning patients.87 Traditionally, VF and VT have been considered “good” cardiac arrest rhythms, resulting in much better outcomes than after asystole and PEA. However, GWTG-R data establish that survival to discharge was more common among children with initial VF/VT than among children with subsequent VF/VT (35% vs. 11%; odds ratio 2.6, 95% confidence interval [CI] 1.2-5.8).30 Surprisingly, the subsequent VF/VT group had worse outcomes than children with asystole/PEA (11% vs. 27% survival). These data suggest that outcomes after initial VF/VT in children (an arrhythmogenic arrest) are “good,” but outcomes after subsequent VF/VT (i.e., VF/VT in the setting of an asphyxial or ischemic arrest) are worse, even compared with initial asystole/PEA without subsequent VF/VT. Defibrillation is necessary for successful resuscitation from VF cardiac arrest. When prompt defibrillation is provided soon after the induction of VF in a cardiac catheterization laboratory, the rates of successful defibrillation and survival approach 100%. In general, the mortality rate increases by 5 to 10% per minute of delay to defibrillation.88 Because pediatric cardiac arrests are commonly a result of progressive asphyxia or shock (or both), the initial treatment of choice is prompt CPR, not defibrillation. This emphasis is balanced against the increasing evidence that VF in children is not rare, that outcomes after arrhythmogenic VF arrests are superior to those after other types of cardiac arrests, and that early rhythm recognition is necessary for optimal care. Because of the increasing awareness that “shockable” rhythms are not uncommon in children, greater attention has been focused on the dose for pediatric defibrillation. The recommended shock dose is 2 to 4 joules (J)/kg, which is based on animal studies of short-duration VF and a single retrospective study of in-hospital (short-duration) VF with 91% (52 of 57) defibrillation success.89 More recent animal and pediatric data indicate that 2 J/kg is often ineffective at terminating fibrillation, and higher doses up to 10 J/kg may be needed to terminate VF.89,91 Thus recent recommendations suggest an initial dose of 2 to 4 J/kg followed by 4 J/kg if VF is not terminated; if VF continues, consider increasing the defibrillation dose up to 10 J/kg, not to exceed adult maximum doses. Bradycardia with hypoperfusion (without pulselessness) is a very common hemodynamic state for critically ill children during the prearrest phase. Early clinical studies of terminally ill children demonstrated almost ubiquitous prevalence of bradycardia before the onset of cardiac arrest.93 Animal models of asphyxia have demonstrated a predictable hemodynamic progression from tachycardia to bradycardia with hypotension, followed by PEA and asystole,94 and that CPR earlier in this continuum is associated with more favorable outcomes.82,95 Given that the majority of children with cardiac arrest experience respiratory insufficiency or circulatory insufficiency or both before the onset of pulselessness, a bradycardic child in shock should be considered to be in a prearrest state. Multiple reversible causes need to be considered, but immediate support of cardiovascular status is essential. Neonatal resuscitation algorithms have recommended escalation of respiratory and cardiac support for the neonate whose heart rate is less than 60 beats/min, including the provision of chest compressions if bradycardia does not resolve with effective ventilation and oxygenation. Multiple studies of pediatric patients from the GWTG-R database have shown that 18 to 54% of patients younger than age 18 who receive chest compressions in the hospital are in a state of bradycardia and hypoperfusion, as opposed to pulselessness, when CPR is initiated.19,25,96 A multivariate analysis from the NRCPR demonstrated an association between CPR for bradycardia (as opposed to pulseless arrest) and survival to discharge, even when controlling for age, diagnosis, and event characteristics.96 Current AHA guidelines recommend the consideration of immediate chest compressions for a child with a heart rate of less than 60 with obvious hypoperfusion.86 The use of extracorporeal cardiovascular support for cardiac arrest depends on the rapid availability of the resources, equipment, and personnel to establish mechanical circulatory support, most typically ECMO or, less commonly, VADs. Multiple case series have reported favorable outcomes in children placed on ECMO during cardiac arrest, with some demonstrating a relationship between shorter duration of CPR before ECMO support and survival.97–99 One retrospective series of 66 children placed on ECMO for cardiac arrest demonstrated an overall survival to hospital discharge of 45%; the median time of CPR before established ECMO circulation was 46 minutes.100 Another single-center study demonstrated 7 of 31 patients (22.5%) surviving to discharge after extracorporeal cardiopulmonary resuscitation (ECPR).101 Current consensus statements from the AHA state that there is insufficient evidence for a time-based threshold within which ECPR may be beneficial.86 Centers with resources for ECPR may consider its use for patients with other epidemiologic features known to be favorable (e.g., witnessed arrests, short CPR times).
Pediatric Resuscitation
Cardiac Arrest and Cardiopulmonary Resuscitation
Epidemiology
Pathophysiologic Principles
Pediatric Anatomy Relevant to Cardiopulmonary Resuscitation
Clinical Features
Four Phases of Cardiac Arrest
Management
Circumferential versus Focal Sternal Compressions
Chest Compression Rate
Chest Compression–Ventilation Ratios (Table 10-2)
Leaning
Real-Time Cardiopulmonary Resuscitation Feedback
Compression-Ventilation (Standard) versus Compression-Only (“Hands-Only”) Cardiopulmonary Resuscitation
Advanced Life Support Medications during the Low-Flow Phase of Cardiopulmonary Resuscitation
Pediatric Ventricular Fibrillation and Ventricular Tachycardia
Defibrillation
Cardiopulmonary Resuscitation in Pediatric Bradycardia
Extracorporeal Circulatory Support during Cardiac Arrest
Stay updated, free articles. Join our Telegram channel

Full access? Get Clinical Tree
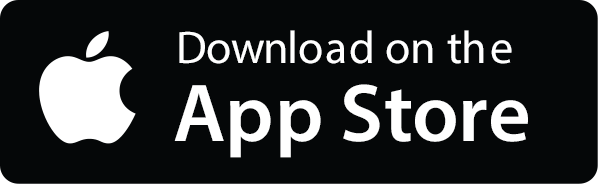
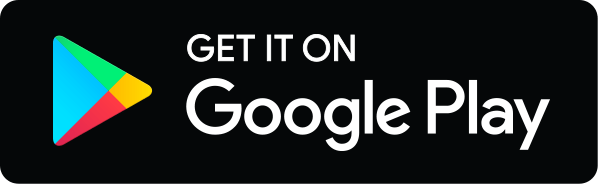