I. Pulmonary arterial hypertension
Idiopathic
Heritable
Drug and toxin induced
Associated with:
Connective tissue diseases
HIV infection
Portal hypertension
Congenital heart diseases
Schistosomiasis
Chronic hemolytic anemia
Persistent pulmonary hypertension of the newborn
I′. Pulmonary venoocclusive disease and/or pulmonary capillary hemangiomatosis
II. Pulmonary hypertension owing to left heart disease
III. Pulmonary hypertension owing to lung disease and/or hypoxia
IV. Chronic thromboembolic pulmonary hypertension
V. Pulmonary hypertension with unclear multifactorial mechanisms
Nitric Oxide
Class: Inhaled Selective Pulmonary Vasodilator
Nitric oxide (NO), previously termed endothelium-derived relaxing factor, is a potent endogenous vasodilator, as well as a valuable signaling molecule [6]. Originally described for its use in the treatment of persistent pulmonary hypertension of the newborn (PPHN) [7], inhaled nitric oxide (iNO) therapy has been extended to usage for right ventricular dysfunction with increased pulmonary vascular resistance following left ventricular assist device placement [8], orthotopic heart [9], lung and liver transplantation [10], and surgery for congenital heart disease [11].
Mechanism of Action
Nitric oxide is synthesized by nitric oxide synthase (NOS) from L-arginine to citrulline in pulmonary endothelial cells. Nitric oxide generated in the endothelium diffuses into adjacent vascular smooth muscle cells. The generation of NO influences guanylate cyclase to increase production of cyclic-guanine monophosphate (cGMP). cGMP decreases free intracellular calcium thereby causing vascular smooth muscle relaxation [12] (Fig. 17.1). Nitric oxide is absorbed into the blood where it binds to oxyhemoglobin forming methemoglobin or it combines with deoxyhemoglobin forming nitrosyl-hemoglobin, which is oxidized to methemoglobin with the release of nitrates [13]. Because of its rapid degradation and inhalational delivery, administration of NO has virtually no systemic vasodilatory effects.


Fig. 17.1
Nitric oxide (NO) and prostacyclin (PGI 2 ) signaling pathways in the regulation of pulmonary vascular tone. NO is synthesized by NO synthase (NOS) from the terminal nitrogen of L-arginine. NO stimulates soluble guanylate cyclase (sGC) to increase intracellular cGMP. PGI2 is an arachidonic acid (AA) metabolite formed by cyclooxygenase (COX-1) and prostacyclin synthase (PGIS) in the vascular endothelium. PGI2 stimulates adenylate cyclase in vascular smooth muscle cells, which increase intracellular cAMP. Both cGMP and cAMP indirectly decrease free cytosolic calcium, resulting in smooth muscle relaxation. Specific phosphodiesterases (PDE) hydrolyze cGMP and cAMP, thus regulating the intensity and duration of their vascular effects. Inhibition of these PDE with such agents as sildenafil and milrinone may enhance pulmonary vasodilation (From Steinhorn [27] and Porta and Steinhorn [12]; with permission)
Indications
Nitric oxide is used in the setting of acute increases in pulmonary vasoreactivity with associated right ventricular dysfunction, as well as therapy to decrease intrapulmonary shunt. It is usually administered with mechanical ventilation but can also be administered with continuous positive airway pressure (CPAP) or nasal cannula apparatus. The initial recommended starting dose is 20 parts per million (ppm). A higher dose of 40 ppm may be utilized if no response is readily noted within 20 min of initiating therapy; however, it should be noted that failure to respond at 20 ppm is rarely associated with a favorable response at a higher dose [7]. Due to its inhalational delivery, nitric oxide is delivered only to ventilated lung units thereby increasing perfusion to those selective areas decreasing intrapulmonary shunt. Inhaled NO therapy for PPHN has reduced the need for extracorporeal membrane oxygenation (ECMO) in infants >35 weeks’ estimated gestational age, yet it has not demonstrated a change in overall mortality [7]. Nitric oxide may also have a protective role in the inflammatory response as it has been shown to inhibit platelet aggregation, block monocyte adherence/migration, and inhibit vascular smooth muscle cell proliferation [6].
Inhaled NO is also used during diagnostic right heart catheterization to assess a patient’s response to acute vasodilator therapy. Those patients with pulmonary arterial hypertension who meet the defined criteria of a decrease in mean pulmonary artery pressure ≥10 mmHg to a value of ≤40 mmHg with an increase or unchanged cardiac output are termed “responders” and are initially treated with calcium-channel blockers for their disease [4]. However, the proportion of patients with a favorable response is <10 % [14]. Additional agents which may be used in this type of provocative testing are intravenous epoprostenol and adenosine [4].
Drug Interactions and Contraindications
The administration of high doses of nitric oxide and prolonged usage can result in high levels of nitric oxide, which can compete with oxygen. The use of iNO treatment requires monitoring for the formation of nitrogen dioxide (NO2) [6]. Cytotoxicity related to the formation of free oxygen radicals may worsen existing lung injury and interfere with mitochondrial respiration [6].
Prolonged administration and higher doses of nitric oxide increases the risk of developing significant methemoglobinemia. Methemoglobin levels should be measured frequently and kept <2.5 % [2]. The use of additional nitrate compounds such as nitroglycerin or sodium nitroprusside may have additive effects increasing the risk of developing methemoglobinemia.
Side Effects
Abrupt cessation in inhaled nitric oxide therapy may be followed by a severe rebound in pulmonary hypertension secondary to the downregulation of endogenous nitric oxide production. If deterioration occurs during weaning or after treatment has been discontinued, the dose should be increased to the previous level or inhaled NO therapy restarted [2]. The use of sildenafil prior to discontinuation of inhaled NO therapy has been shown to prevent rebound pulmonary hypertension [12].
Clinical Pearls
I.
Initial dose is 20 ppm.
II.
Requires specialized delivery system with monitoring for NO2.
III.
If difficulty is encountered with weaning, consider the addition of sildenafil and/or bosentan prior to reinitiating the weaning process.
Prostanoids
Prostacyclin is derived from the action of cyclooxygenase on arachidonic acid. Prostacyclin generated in pulmonary endothelial cells binds to the smooth muscle cell membrane-bound receptor stimulating adenylate cyclase to increase levels of cyclic adenosine monophosphate (cAMP) [12]. cAMP works to decrease intracellular calcium levels thereby causing vasodilatation [12] (Fig. 17.1). Prostacyclin is also a strong inhibitor of platelet aggregation, as well as an inhibitor of smooth muscle proliferation. Patients with pulmonary arterial hypertension are known to have decreased levels of prostacyclin causing an imbalance which favors vasoconstriction and pulmonary vascular remodeling [5].
Epoprostenol (Flolan®)
Class: Intravenous Synthetic Prostacyclin Analogue
Epoprostenol was approved by the FDA in 1995 for the treatment of pulmonary arterial hypertension. It is the only agent to date which has demonstrated survival benefit in this particular patient population [5].
Mechanism of Action
Epoprostenol functions like endogenous prostacyclin to increase cAMP levels which in turn decrease intracellular calcium levels thereby promoting pulmonary smooth muscle cell vasodilatation. Due to its short half-life of 3 min, epoprostenol must be administered as a continuous intravenous infusion requiring a dedicated central venous catheter [15]. The drug must be reconstituted in an alkaline solution, kept in a cool pack during administration, and be protected from light [16]. Once reconstituted, the solution is only good for 24 h. Initial dosing is started at 2 nanograms per kilogram per minute (ng/kg/min) and increased by increments of 2 ng/kg/min every 15 min based on response and any noted side effects. The maximum recommended dose is 40 ng/kg/min. Epoprostenol is rapidly hydrolyzed at neutral pH in the blood with its metabolites excreted in the urine.
Indications
Epoprostenol is considered worldwide to be the first line of therapy in patients with advanced disease (World Health Organization [WHO] functional classes III–IV) or those who are unresponsive to oral pulmonary vasodilator therapies [14] (Table 17.2).
Table 17.2
World Health Organization functional classifications of patients with pulmonary hypertension [26]
I. No limitation of usual physical activity; ordinary physical activity does not cause increased symptoms |
II. Mild limitation of usual physical activity; no discomfort at rest, but normal physical activity causes symptoms |
III. Marked limitation of physical activity; no discomfort at rest, although less than normal activity causes symptoms |
IV. Unable to perform any physical activity and may have signs of right ventricular failure; dyspnea and/or fatigue present at rest, and any physical activity causes symptoms |
Drug Interactions and Contraindications
Epoprostenol has both systemic and pulmonary vasodilatory effects. If used in combination with other antihypertensives, there is an increased risk for exaggerated decreases in systemic blood pressure. Epoprostenol also has antiplatelet aggregation properties, and if used concurrently with other antiplatelet agents or anticoagulants, the risk for bleeding is increased. It has also been shown to decrease the clearance of digoxin, which is important as digoxin is commonly used as part of conventional treatment for pulmonary arterial hypertension.
Side Effects
The most concerning side effect is systemic vasodilatation. A drop in systemic blood pressure may compromise coronary blood flow particularly to the right ventricle leading to worsening of its function [17]. Other reported systemic side effects which usually limit further epoprostenol dosage increases are headache, flushing, jaw pain, bone pain, nausea, diarrhea, and dizziness. Because epoprostenol does increase pulmonary perfusion, the presence of underventilated lung areas may lead to worsening of ventilation/perfusion mismatch in patients with existing lung disease.
Clinical Pearls
I.
Tachyphylaxis is quite common.
II.
The interruption of the continuous infusion can lead to severe rebound pulmonary hypertension; therefore, patients should always have a backup infusion pump as well as a spare drug cartridge readily available.
III.
Risk of central line catheter infection.
IV.
At higher doses, it may cause high-output cardiac failure.
Treprostinil (Remodulin®, Tyvaso®)
Class: Subcutaneous/Intravenous/Inhalational Synthetic Prostacyclin Analogue
Mechanism of Action
Being a prostacyclin analogue, treprostinil stimulates increased production of cAMP which decreases intracellular calcium in pulmonary vascular smooth muscle cells causing vasodilatation. Treprostinil has a longer half-life of 3–4 h in comparison to epoprostenol. This longer half-life allows treprostinil to be administered via a continuous subcutaneous route. Its neutral pH solution is stable at room temperature, making it much more convenient for patient care administration compared to epoprostenol [15]. For those patients who cannot tolerate the subcutaneous route, intravenous administration is an alternative option. However, intravenous administration requires central venous access with its attendant risks of infection and thrombosis. Initial dosing for both continuous subcutaneous and intravenous administration begins at 0.625–1.25 ng/kg/min with incremental increases of 1.25 ng/kg/min weekly for the first month. Afterward, further incremental increases are done at 2.5 ng/kg/min with a maximum recommended dosage of 40 ng/kg/min. Inhalational delivery dosage starts at three breaths which is equal to 18 micrograms (mcg) per treatment which is done four times daily while awake. Dosing is increased by three breaths every 1–2 weeks up to nine breaths per treatment (54 mcg) [15].
Indications
Treprostinil was initially approved by the FDA in 2002 for the treatment of patients with pulmonary arterial hypertension in WHO functional classes II–IV. The approval for intravenous administration followed in 2004 and subsequent approval for inhalation delivery in 2009.
Drug Interactions and Contraindications
Subcutaneous and intravenous administration of treprostinil during concurrent administration of antihypertensives may result in exaggerated decreases in systemic blood pressure. As with other prostanoids, treprostinil inhibits platelet aggregation and may increase the risk of bleeding in the presence of other antiplatelet agents and anticoagulants. Treprostinil is metabolized in the liver by cytochrome P450 (CYP) 2C8. CYP inhibitors like gemfibrozil decrease the clearance of treprostinil, while CYP inducers such as rifampin increase its clearance. Treprostinil itself however does not induce or inhibit CYP enzymes.
Side Effects
With the subcutaneous route of administration, the most common reported side effect is pain and erythema at the injection site. As with other prostanoids, systemic vasodilatation occurs with its associated symptomatology of headache, flushing, jaw pain, bone pain, nausea, and diarrhea and dizziness.
Clinical Pearls
I.
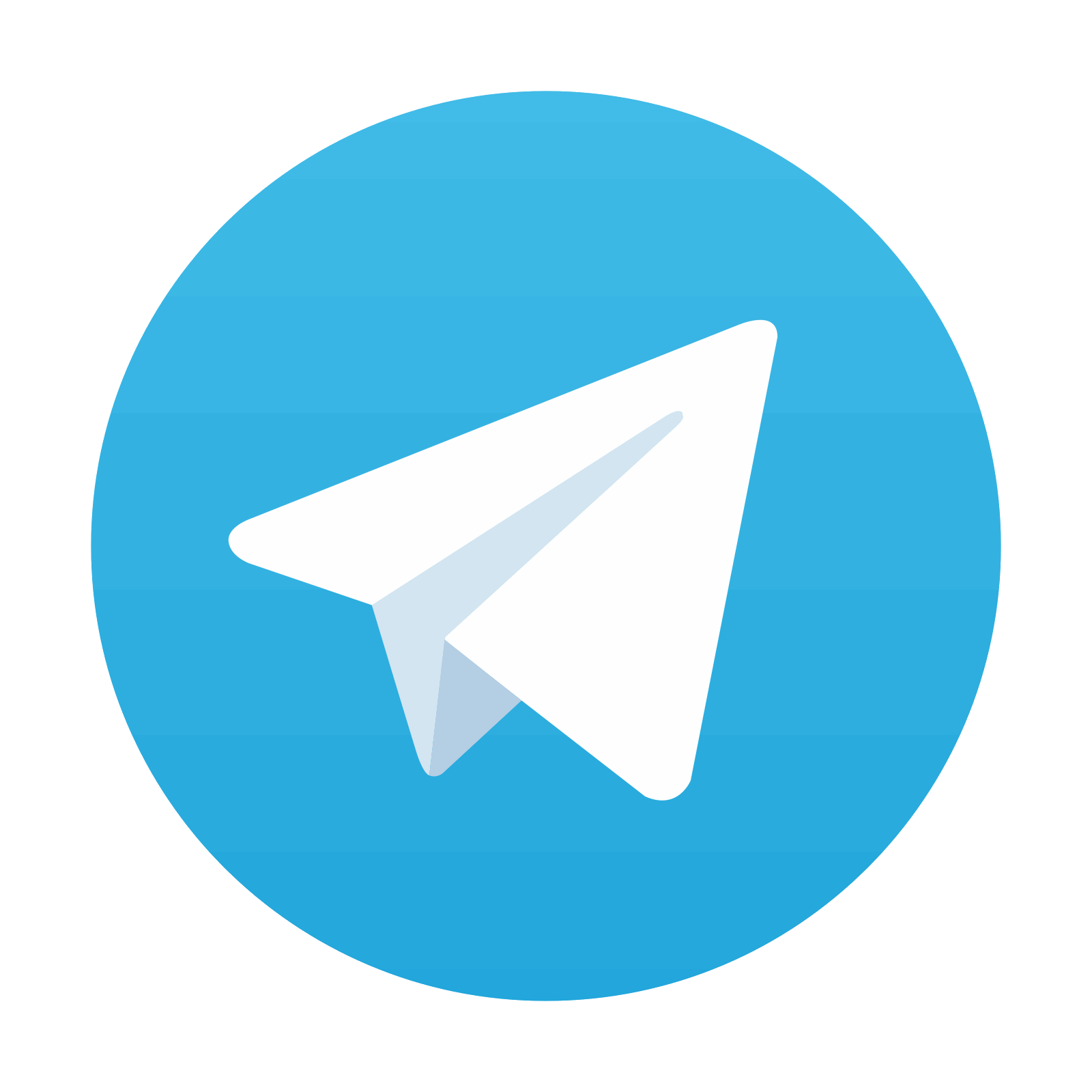
It is suggested with subcutaneous administration to change sites every 72 h.
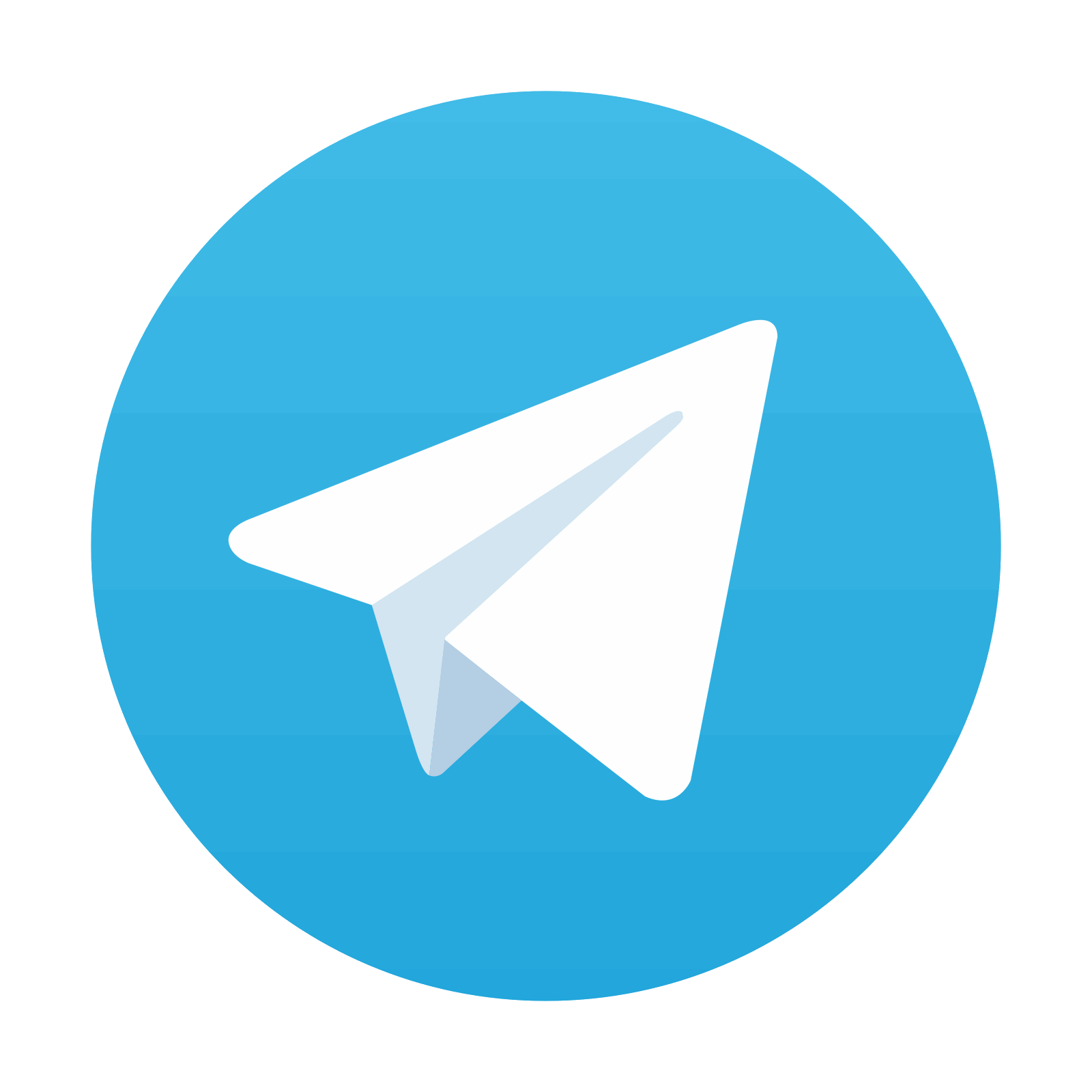
Stay updated, free articles. Join our Telegram channel

Full access? Get Clinical Tree
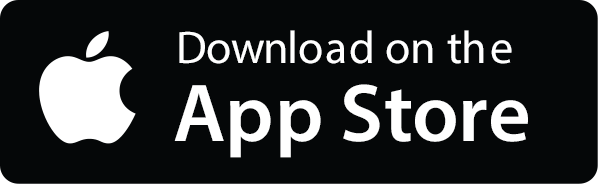
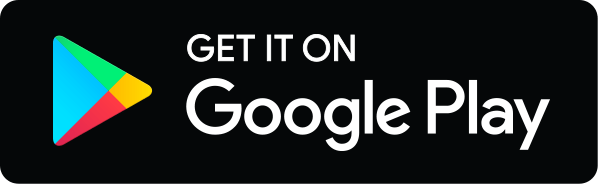
