Negative-Pressure Ventilation: Introduction
Conventional mechanical ventilation via endotracheal intubation or tracheostomy in the treatment of acute respiratory failure (ARF) is a lifesaving procedure. Yet it exposes patients to severe complications, including upper airway trauma and nosocomial pneumonia, and may prolong the length of stay in the intensive care unit (ICU) and hospital because additional time may be necessary for weaning.1–4
Basic Principles of Negative-Pressure Ventilation
Negative-pressure ventilation (NPV) works by exposing the surface of the thorax to subatmospheric pressure during inspiration. This pressure causes thoracic expansion and a decrease in pleural and alveolar pressures, creating a pressure gradient for air to move from the airway opening into the alveoli. When the pressure surrounding the thorax increases and becomes atmospheric or greater, expiration occurs passively owing to the elastic recoil of the respiratory system. The inspiratory changes with NPV, in pleural and alveolar pressures, replicate those during spontaneous breathing. On the contrary, positive-pressure ventilation (PPV) causes an increase in intrathoracic pressures during inspiration (Fig. 16-1).
Figure 16-1
Airway and intrathoracic pressures during positive-pressure ventilation (upper panel) and during negative-pressure ventilation (bottom panel). Palv, Alveolar pressure; PCW, elastic recoil pressure of the chest wall; PL, elastic recoil pressure of the lung; Paw, airway pressure; Pmus, muscle pressure; Ppl, pleural pressure; Ptank, tank ventilator pressure.
All NPVs have two major components: an airtight, rigid chamber that encloses the rib cage and abdomen and a pump that generates pressure changes in the chamber.5,6
Tank ventilators enclose the body up to the neck. The advantage is that chest wall expansion is not limited by contact with the sides of the chamber, and only one airtight seal is required around the neck. Most modern tank ventilators are constructed of aluminium and plastic and are lighter than previously. The patient’s body rests on a thin mattress, and the head protrudes through a porthole at one end of the ventilator. A head and neck rest is provided in most designs to ensure comfort and to prevent upper airway collapse. Most tank ventilators have windows allowing patient observation and portholes for catheters and monitor leads and where procedures can be performed (Fig. 16-2).7
This ventilator is a windproof, water-permeable nylon parka suspended over a rigid grid that includes the rib cage and abdomen. It allows the application of negative pressure over the anterior portion of the chest wall.8 Airtight seals around the neck, arms, and hips are required to prevent air leakage. The jackets do not restrain expansion of the rib cage and abdomen, but are awkward for many patients to put on and often cold to wear because of air leaks. They are preferable to tank ventilators for home use but less efficient for treating patients with ARF. The tidal volume they develop at any given level of negative pressure is less than that of a tank ventilator, and the peak pressure that patients can tolerate also usually is slightly less.6
This consists of a rigid shell fitting firmly over the anterior portion of the chest. It applies negative pressure over a smaller surface area than either the iron lung or jacket and is the least efficient NPV.8 Its efficiency improves if the anterior abdominal wall is enclosed in the device and movement of the lateral aspect of the upper rib cage is not restrained. Proper fitting can be difficult, and tailor-made fiberglass shells often are necessary, particularly in patients with kyphoscoliosis.
Negative pressure is generated by bellows or rotary pumps that are separate or incorporated into the structure of the ventilator. Modern rotary pumps perform pressure-preset ventilation.9 After a breath is initiated, these pumps apply and maintain a targeted amount of subatmospheric pressure around the chest wall to meet a specified time-cycling criterion (Fig. 16-3). This mode is equivalent to pressure-controlled ventilation available with the latest PPVs. As with positive-pressure preset ventilation, tidal volume during NPV is a complex function of applied pressure and its rate of approach to target pressure, available inspiratory time, and the impedance to breathing (compliance of the respiratory system, airway resistance, and dynamic hyperinflation with intrinsic positive end-expiratory pressure [PEEP]).
Traditionally, NPV is controlled mechanical ventilation, and the device provides a fixed number of breaths per minute. If mechanical and spontaneous respiratory cycles do not match, the patient “fights” the ventilator, resulting in discomfort. Unlike PPV, during NPV, the airway opening is free; consequently, it is not possible to monitor airway pressure or flow continuously and to use these signals to trigger a mechanical breath. This patient-triggering inability may contribute to poor patient synchrony and induction of upper airway collapse secondary to the lack of coordinated activity between the upper airway and the inspiratory muscles.10 To overcome this limitation, some NPVs have incorporated patient-triggered modes using pressure change sensed via nasal prongs. There are data indicating that this technology is slow and relatively insensitive to patient inspiratory efforts.11 Recently, the performance of a prototype microprocessor-based iron lung capable of thermistor triggering was evaluated.12 The device used to trigger the iron lung was thermally sensitive and similar to that used in sleep studies, and it was activated by temperature changes caused by the onset of inspiratory airflow (Fig. 16-4). In normal subjects and patients with chronic obstructive pulmonary disease (COPD) recovering from ARF, we measured (a) the time delay between airflow onset and start of an assisted breath, (b) pressure-time product for the diaphragm per minute (PTPdi) during triggered breaths, and (c) nontriggering inspiratory efforts (Fig. 16-5). At maximum trigger sensitivity, the time delay was about 0.21 second in both groups. PTPdi was reduced markedly. Nontriggering inspiratory efforts were 1.1% and 2.3% of total breaths, respectively. Although the time delay of the thermistor trigger is longer than most recent flow-triggering and pressure-triggering systems of PPVs,13 the study suggests that this system permits the use of assisted NPV with an acceptable patient–ventilator interaction.
Figure 16-5
Recordings of flow, transdiaphragmatic pressure (Pdi), and tank pressure (Ptank) in a patient with chronic obstructive pulmonary disease receiving assist NPV. The continuous vertical line indicates the onset of inspiratory effort, the dashed vertical line indicates the start of inspiratory flow, and the dotted vertical line indicates the start of an assisted breath. The partitioning of the pressure-time product of the diaphragm is shown: effort required to overcome intrinsic positive end-expiratory pressure (PTPdiPEEPi), effort required to trigger the assisted breath (PTPdiTr), and effort exerted in the post-trigger phase (PTPdiPost). TDPEEPi and TDtr indicate the time delay between the onset of inspiratory effort and the start of inspiratory flow and the time delay between the onset of inspiratory flow and the start of assisted breath, respectively. (Used, with permission, from Gorini, et al. Effect of assist negative pressure ventilation by microprocessor based iron lung on breathing effort. Thorax. 2002;57:258–262.)
Presently, NPV can be delivered by five modes: (a) intermittent negative pressure, (b) negative/positive pressure, (c) continuous negative pressure (CNEP), (d) negative pressure/negative end-expiratory pressure (Fig. 16-6), and (e) external high-frequency oscillation.
Figure 16-6
Control panel of a microprocessor-based iron lung (Coppa CA 1001, Coppa, Biella, Italy) during intermittent negative pressure (upper-left panel), negative and/or positive pressure (upper-right panel), continuous negative extrathoracic pressure (lower-left panel), and negative pressure or negative extrathoracic end-expiratory pressure (lower-right panel) ventilation.
The ventilator generates targeted subatmospheric pressure for the selected inspiratory time. Pressure around the chest wall becomes atmospheric during expiration, which occurs passively owing to the elastic recoil of the respiratory system.
The ventilator generates the preset extrathoracic subatmospheric pressure during inspiration and preset extrathoracic positive pressure during expiration. In patients with chest wall disorders, this combination has been found to increase tidal volume more than intermittent negative pressure alone by reducing the end-expiratory volume of the respiratory system.14 A useful application of this option is to assist cough and promote clearance of sputum in patients with copious secretions i.e., cystic fibrosis and bronchiectasis.8
The ventilator provides a constant subatmospheric pressure throughout the respiratory cycle, and the patient breathes spontaneously.
The ventilator generates the preset subatmospheric pressure during inspiration and maintains a preset level of negative pressure throughout the expiration.
High-frequency ventilation using a jet system can be applied via a tracheal tube or a tracheotomy. Alternatively, a cuirass can be used to apply high-frequency oscillation externally.15 The Hayek oscillator includes a cuirass, power unit, and control unit. The power unit has (a) a diaphragmatic pump, which can operate over a wide range of frequencies to generate an oscillating pressure wave, and (b) a vacuum pump, which enables the oscillation to be superimposed on a negative-pressure baseline. Peak inspiratory (up to −70 cm H2O) and peak expiratory (up to 70 cm H2O) pressures, frequency (8 to 999 cycles per minute), and inspiratory-to-expiratory timing (I:E) ratio (1:6 to 6:1) can be set on the control unit.
Two studies suggest that external high-frequency oscillation can provide effective ventilation in healthy subjects15 and improve end-tidal carbon dioxide (CO2) and oxygen (O2) saturation (SaO2) in patients with severe stable COPD.16 In five patients with ARF, short-term application of external high-frequency oscillation improved oxygenation by 16% and reduced by 6%, compared with conventional PPV.15 In a randomized, controlled study, a 4-hour period of external high-frequency oscillation improved cardiac index and tissue perfusion in adult patients after coronary artery bypass grafting compared with conventional PPV.17
Physiologic Effects
Pioneering studies showed NPV, particularly that provided by tank ventilators, to be a highly effective form of mechanical ventilation, capable of maintaining normal arterial blood-gas tensions in patients with little or no spontaneous respiratory activity.18–20 Recently, it has been shown21 that CNEP, NPV, and NPV plus negative extrathoracic end-expiratory pressure provided by a microprocessor-based iron lung were able to improve ventilatory pattern, arterial blood gases, and pH significantly (Fig. 16-7).
Figure 16-7
Values for, PaO2, PaCO2 and pH in seven patients with acute exacerbation of chronic obstructive pulmonary disease during spontaneous breathing (SB), continuous negative extrathoracic pressure (CNEP), negative pressure ventilation (NPV), and negative extrathoracic end-expiratory pressure added to NPV (NPV-NEEP). (Reprinted with permission of the American Thoracic Society. Copyright © 2012 American Thoracic Society. Used, with permission, from Gorini, et al. Am J Respir Crit Care Med. 2001;163:1614–1618. Official Journal of the American Thoracic Society.)
Two studies22,23 reported that in patients with acute exacerbation of COPD, NPV was effective in improving respiratory muscle strength and in decreasing PaCO2. In patients with chronic respiratory failure secondary to COPD and restrictive diseases, NPV reduced electrical and mechanical activity of the inspiratory muscles.24–28 Rodenstein et al29 showed that controlled NPV provided by an old iron lung requires a short period of adaptation to obtain inspiratory muscle rest. Other studies show that when inspiratory and expiratory times are adjusted carefully to approximate the subject’s spontaneous timing components, NPV results in a substantial suppression of electromyographic activity of inspiratory muscles.25,26 Assist-control NPV provided by a cuirass is effective in relief of dyspnea induced experimentally in normal subjects by a combination of inspiratory resistive loading and hypercapnia, probably reducing inspiratory muscle workload.30
The effects of NPV provided by a microprocessor-based iron lung capable of providing CNEP and assist-control NPV on respiratory mechanics and inspiratory muscle effort in patients with COPD with ARF were evaluated by measuring the pressure-time product of the diaphragm and the electromyographic activity of parasternal muscles.21 Compared with spontaneous breathing, CNEP (−5 cm H2O) resulted in a significant decrease in dynamic intrinsic PEEP and pressure-time product of the diaphragm, whereas assist-control NPV caused a significant improvement in the pattern of breathing associated with a marked reduction in both pressure-time product of the diaphragm and electromyographic activity of the parasternal muscles. The application of −5 cm H2O of negative extrathoracic end-expiratory pressure during NPV further decreased the pressure-time product of the diaphragm slightly and improved patient–ventilator interaction by reducing dynamic intrinsic PEEP and nontriggering inspiratory effort (Fig. 16-8). Reduction in diaphragmatic effort obtained during assist-control NPV21 is similar to that measured in patients with an acute exacerbation of COPD with pressure-support ventilation.31,32
Figure 16-8
Recordings of volume, flow, pleural pressure (Ppl), gastric pressure (Pga), transdiaphragmatic pressure (Pdi), and tank pressure (Ptank) in a patient with an acute exacerbation of COPD during spontaneous breathing (SB), continuous negative extrathoracic pressure (CNEP), negative-pressure ventilation (NPV), and negative extrathoracic end-expiratory pressure added to NPV (NPV-NEEP). The dashed lines indicate the level of zero in flow, Ppl, Pga, and Pdi recordings. (Reprinted with permission of the American Thoracic Society. Copyright © 2012 American Thoracic Society. Used, with permission, from Gorini, et al. Am J Respir Crit Care Med. 163:1614–1618. Official Journal of the American Thoracic Society.)
The application of NPV during sleep in normal subjects,33 and in patients with chronic respiratory failure secondary to COPD10 and neuromuscular disorders,34,35 may result in the development of recurrent episodes of apnea and hypopnea, as well as altered sleep quality.10,34 However, a recent controlled study in patients with neuromuscular disorders showed that NPV resulted in a general improvement in sleep quality and oxygen saturation.36 It has been reported that in normal subjects during voluntary respiratory muscle relaxation, NPV caused a decrease in the caliber of the upper airway at the glottic or supraglottic level.37 In normal, awake subjects, the glottis width did not decrease with the increase in negative pressure applied to the chest wall.38 Consequently, the increasing level of NPV resulted in progressive increases in tidal volume and minute ventilation. On the contrary, control positive-pressure ventilation provided by mask caused inspiratory adduction of the vocal cords, which reduced the tidal volume effectively reaching the lungs.39,40
The mechanisms of upper airway obstruction observed with NPV at supraglottic level have not been elucidated fully. During spontaneous breathing, activation of the pharyngeal and laryngeal muscles precedes activation of the inspiratory muscles, resulting in stiffening of the upper airway walls. When NPV is applied during sleep or in completely relaxed subjects, this coordinated respiratory muscle activity may be abolished. Consequently, the subatmospheric pressure developed in the upper airway during inspiration may result in their collapse.5,10,34,37 Upper airway obstruction has been reported in two of ten patients with acute-on-chronic respiratory failure during treatment with NPV41 and was the reason for NPV failure in 16% of patients in a large prospective cohort study.42
NPV may induce a lower esophageal sphincter dysfunction in healthy subjects43 and in patients with COPD.44 This dysfunction may cause regurgitation of stomach contents and expose patients to the risk of aspiration. It can be prevented completely with metoclopramide.44
The hemodynamic effects of mechanical ventilation are complex and are the result of changes in intrathoracic pressure and lung volume, which independently can affect the determinants of cardiovascular performance.45–47 The increase in intrathoracic pressure caused by PPV decreases both venous return to the right ventricle and left-ventricular afterload. The net effect of this reduction depends on the cardiac function. When left-ventricular function is impaired, cardiac output increases in response to the rise in intrathoracic pressure because the decrease in left-ventricular afterload secondary to the reduction in transmural pressure has a greater effect than the decrease in venous return.48 When left-ventricular function is normal, the increase in intrathoracic pressure reduces cardiac output because the decrease in venous return has more effect than the decrease in left-ventricular afterload.49 In clinical situations such as hypovolemia, septic shock, and gas trapping associated with airflow obstruction, the reduction in cardiac output is more relevant.50
Although the hemodynamic effects of NPV have not been studied extensively,51 the effects are assumed to be the opposite of those of PPV, that is, more physiologic and more likely to maintain a normal cardiac output. The exposure of the entire body (except for the airway opening) to NPV by tank ventilators, however, results in the same hemodynamic effects as occurs with PPV.52 These effects occur because intrathoracic pressure actually is raised relative to body surface pressure, reducing the gradient for venous return. This consequence is not seen when NPV is confined to the thorax and upper abdomen using a cuirass or poncho-wrap.53,54 Unlike tank ventilators, these machines selectively decrease intrathoracic pressure so that right-atrial pressure becomes more negative relative to the rest of body, potentially enhancing the gradient for venous return. In an animal model of acute lung injury, NPV plus negative extrathoracic end-expiratory pressure, provided by poncho-wrap, resulted in a similar improvement in gas exchange and in higher cardiac output compared with PPV plus PEEP.53 In anaesthetized dogs, PPV plus PEEP and NPV plus negative extrathoracic end-expiratory pressure applied by iron lung had a similar effect on cardiac output, whereas the latter was higher with NPV plus negative extrathoracic end-expiratory pressure applied by poncho-wrap compared with the other two modes.54
NPV provided by cuirass does not induce adverse hemodynamic effects in stable patients with COPD,55 whereas a significant reduction in cardiac output has been reported with mask ventilation with PEEP in patients with COPD, both when stable56 and during acute exacerbations.57 Short-term studies have compared the effects of CNEP provided by cuirass58 or poncho-wrap59,60 with those of PEEP in intubated patients with acute lung injury receiving volume-controlled ventilation. CNEP was adjusted to obtain the same change in transpulmonary pressure60 or functional residual capacity58 as with PEEP. The combination of volume-controlled ventilation with CNEP, compared with volume-controlled ventilation with PEEP, resulted in significant increases in oxygen delivery and cardiac index, whereas arterial oxygen content and PaO2/FIO2 ratio did not differ between the two modes of ventilation.58–60 Further studies are required to define the feasibility of long-term clinical treatment with CNEP during controlled ventilation via endotracheal intubation and its effects on clinical outcomes. Children with congenital heart diseases, submitted to right-sided heart surgery with Fontan-type procedures, have unique cardiopulmonary physiology. In the absence of a right ventricle, pulmonary blood flow, the major determinant of cardiac output, is exquisitely sensitive to changes in intrathoracic pressure. In these patients, NPV, provided by cuirass, markedly increased pulmonary blood flow and cardiac output compared with volume-controlled PPV.61,62
Recently, the effects of CNEP applied to the chest through a cuirass during PPV in synchronized intermittent mandatory ventilation mode were evaluated in twenty patients with normal left-ventricular ejection fraction 2 hours after coronary artery bypass graft. With CNEP and synchronized intermittent mandatory ventilation, the stroke-volume index and cardiac index were significantly increased compared to ventilation with synchronized intermittent mandatory ventilation and PEEP. CNEP also reduced venous pressure and wedge pressure. Although the improvement in cardiac index and stroke volume was modest, the application of CNEP during PPV may have a greater benefit in patients with reduced ventricular function.63
Rationale, Advantages, and Limitations
NPV can be used to widen the field of application of noninvasive ventilator techniques. It is well known that compared with standard medical treatment, mask ventilation reduces the need for endotracheal intubation64–66 and reduces hospital mortality64–67 in selected patients with an acute exacerbation of COPD. A randomized study comparing mask with conventional mechanical ventilation in patients with exacerbations of COPD who failed medical treatment has shown that mask ventilation avoided endotracheal intubation in 48% of patients.68 Mask ventilation, however, is not without its problems, and failure rates of 7% to 50% are reported.69 Severe respiratory acidosis70–72 and illness at presentation,70,73 excessive airway secretions,73 and inability to minimize the amount of air leakage73 are major factors associated with failure of this technique.
In clinical studies, NPV has been used successfully in patients with severe respiratory acidosis or impaired level of consciousness.74–76 During NPV, the airway opening is free, unlike in PPV, and consequently, performing bronchial aspiration or fiberoptic bronchoscopy to remove excessive airway secretions is easy. Finally, NPV can be used in patients who cannot tolerate a mask because of facial deformity, or as a rescue therapy to avoid endotracheal intubation in those in whom mask ventilation fails. Routine implementation of noninvasive PPV in critically ill patients with acute exacerbations of COPD or severe cardiogenic pulmonary edema was associated with improved survival and reduction of nosocomial infection.77 For these reasons, mask ventilation is recommended as the standard method of ventilator support for exacerbations of COPD; invasive mechanical ventilation is regarded as second-line rescue therapy when mask ventilation fails.78
To verify the hypothesis that using both noninvasive mask and iron-lung ventilation should further reduce the need for endotracheal intubation in patients with acute on chronic respiratory failure, a prospective cohort study was carried out in 258 consecutive patients42
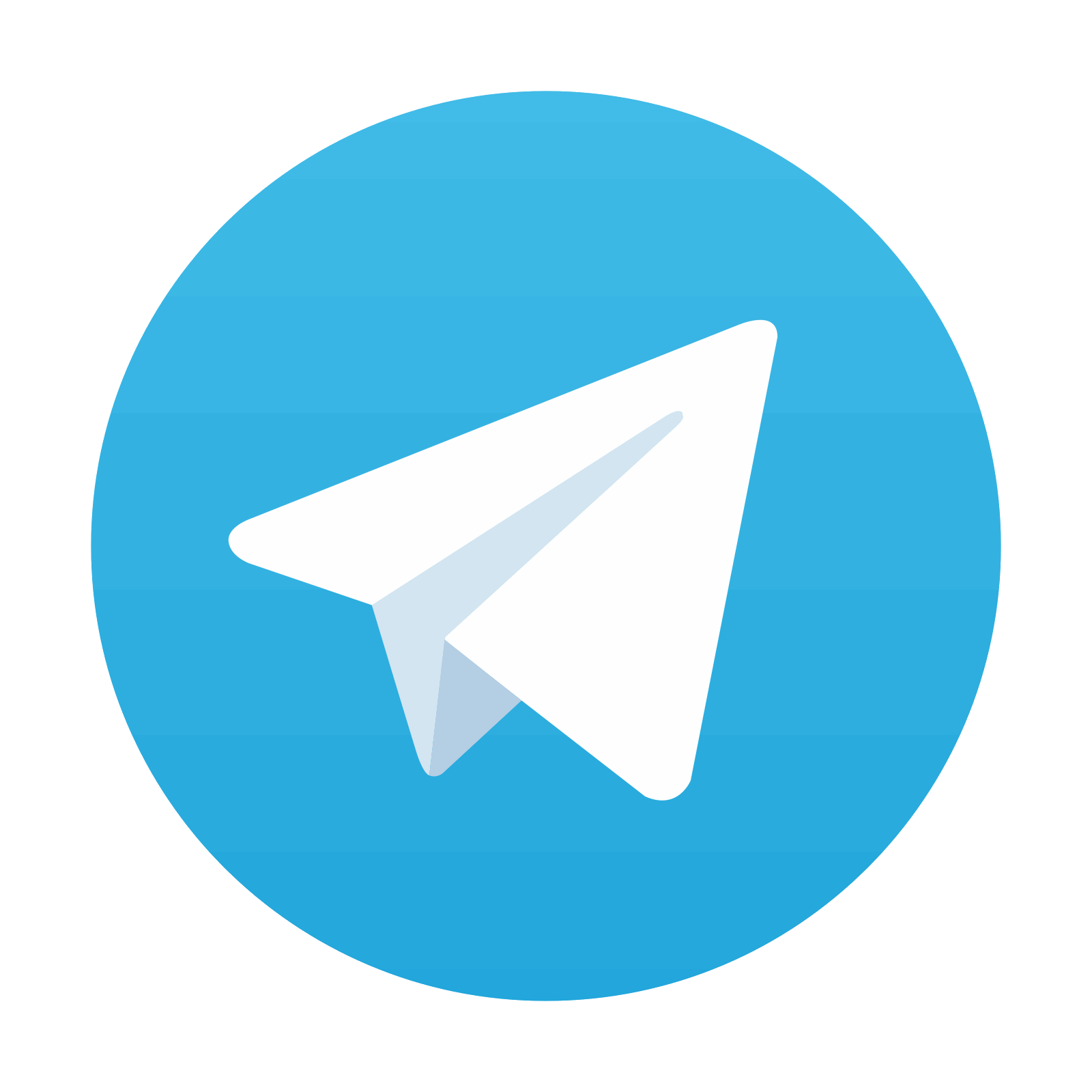
Stay updated, free articles. Join our Telegram channel

Full access? Get Clinical Tree
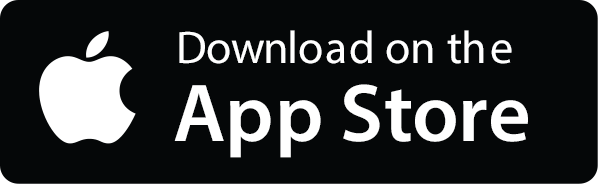
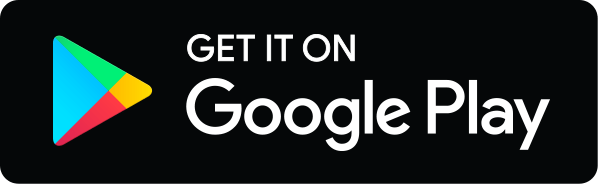
