Goals to prevent MOF
•Prevent ventilatory failure by early support, not allowing the lungs to fail and produce hypoxemia.
•Avoid fluid overload, maintaining a urine output of 25–50 ml/h and no more.
•Avoid excess sodium and sodium bicarbonate.
•Filter blood before transfusion.
•Insist on sighing and deep breathing during operation, during resuscitation, and afterward.
•Maintain adequate cardiac output by circulatory support using inotropic agents early such as isoproterenol, dopamine, and epinephrine.
•Empty the stomach, keep it empty and instill antacids after operation or injury.
•Continue controlled ventilation after operation if ventilatory problems are anticipated.
•Follow a sigh-suction-sit treatment program for ventilation.
•Prevent renal failure by maintaining renal blood flow and urine output.
•Use diuretics or dialysis early.
•Provide for early nutritional support of such patients.
•With tissue injury, use antibiotics before operation to reduce invasive sepsis.
•Drain septic foci and eliminate continuing peritoneal contamination.
Definitions
In the mid 1980s, after the recognition of sequential organ failure as a syndrome was recognized, multiple terms were used inconsistently by the medical community [11]. These disparate definitions attempting to describe the same physiologic phenomena led to the 1991 consensus conference. The societies of the American College of Chest Physicians (ACCP) and the Society of Critical Care Medicine (SCCM) were present. The goal of this conference was to establish a definition to describe what is now known as the spectrum of physiologic response to infection and/or inflammation. The term “systemic inflammatory response syndrome” (SIRS) was introduced at this conference. Additionally the terms sepsis, severe sepsis, septic shock and multiple organ dysfunction were defined as a result of this meeting (Table 7.2). The term “SIRS” was established to differentiate sepsis from a noninfectious, inflammatory state [12]. SIRS was defined as two or more of the following conditions:
Table 7.2
Definitions of SIRS, sepsis, severe sepsis, and multiple organ dysfunction
SIRS |
---|
•Two or more of the following conditions and can result from infectious or noninfectious causes: •Temperature >38°C or <36°C •Heart rate > than 90 beats per minute •Respiratory rate > than 20 breaths per minute or paCO2 < than 32 mmHg •White blood cell count >12,000 or <4,000, or >10% bands |
Sepsis |
•SIRS in conjunction with an infection is termed sepsis |
Severe sepsis |
•Sepsis associated with organ dysfunction •May include hypotension, elevated lactate, acute renal failure, liver failure, altered mental status, and/or hematologic abnormality |
Septic shock |
•Subset of severe sepsis with the addition of hypotension manifested by •Systolic blood pressure (SBP) <90 mmHg •Mean arterial pressure (MAP) <70 mmHg •Decrease in systolic blood pressure (SBP) >40 mmHg from baseline |
Multiple organ dysfunction (MODS) |
•Presence of altered organ function in an acutely ill patient such that homeostasis cannot be maintained without intervention |
Core body temperature >38°C or <36°C
Heart rate > than 90 beats per minute
Respiratory rate > than 20 breaths per minute
paCO2 <32 mmHg
White blood cell count >12,000 or <4,000, or >10% bands.
SIRS could represent the symptoms from an infectious or noninfectious source. Infection was described as the invasion of normally sterile tissue by organisms. The term “sepsis” was defined as SIRS in conjunction with a confirmed infection. “Severe sepsis” was defined as sepsis associated with organ dysfunction, hypotension or hypoperfusion as evidenced by: elevated lactate, acute renal failure, liver failure, altered mental status and/or hematalogic abnormalities. “Septic shock” was the term established as a subset of severe sepsis with the added additional clinical information of persistent hypotension, despite adequate fluid resuscitation. Hypotension was defined as systolic blood pressure (SBP) <90 mmHg, mean arterial pressure (MAP) <70 mmHg, or a decrease in SBP >40 mmHg from baseline.
MODS was defined as the presence of altered organ function in an acutely ill patient such that homeostasis cannot be maintained without intervention and is the culmination of septic shock and multiple end-organ failure [13]. The 2001 Consensus Conference further expanded on these definitions [14]. A problem similar to the disparate use of the word “sepsis” in the early 1980s remains a problem in regard to the definition of MOF. This is evidenced by a lack of consensus with regard to the innumerable scoring systems available to assess mortality.
Epidemiology
Sepsis, severe sepsis, septic shock, and MOF are commonplace in intensive care units and afflict 1.1 million people annually. Moreover, MOF results in 215,000 deaths in the United States alone. Mortality from the spectrum of sepsis is estimated to be 9.3% of all deaths in the United States [15]. The individual costs of treating a single patient with MOF can be upwards of $150,000 per patient [16]. In the United States alone the costs of treating sepsis and its related sequelae is approximately $24 billion annually [17]. Additionally, the cost of critical care can account for as much as 1% of the gross national product of some countries. The resultant morbidity from this disease and consequent loss of wages and quality of life are difficult to quantify. These costs illustrate the substantial financial and societal burden this disease process inflicts. The irony of MOF is that it emerged as a result of improvements in critical care but that it has remained a substantial encumbrance in terms of morbidity, mortality, and cost despite numerous improvements made in critical care in regard to resuscitation and supportive measures.
The overall mortality ranges between 40 and 60% for MOF in all patients and this mortality increases as more organ systems are affected [18, 19]. The incidence of any organ failure in all ICUs ranges from 30 to 60% [20]. In a 1985 study of intensive care patients by Knaus, single organ failure occurred in approximately one-third of all patients at some point during their ICU stay and MOF occurred in 15% of these patients [21]. MOF following septic shock remains the leading contributor to mortality in ICU patients. In a study by Mayr that looked at causes of death in 3,700 ICU patients, the most common cause of death in a single ICU was MOF (47%) [20]. Specifically regarding trauma patients, traumatic brain injury and uncontrolled hemorrhage remain the leading causes of early death after trauma. MOF is, however, the number one cause of late deaths in trauma patients [22]. Despite our improved understanding of the pathophysiology of this disease, the use of antibiotic agents, and more innovative therapies, there continues to be a high mortality rate for MOF.
Regarding the demographics of sepsis and organ failure, a study by Martin et al. in 2003 elucidated some important differences. This study revealed that men are more likely to have sepsis and are more frequently enrolled in clinical trials despite the predominance of women in the population of the United States. Additionally, African-American men had the youngest age of onset in this study as well as the highest mortality. The reason for these demographic differences is not known; however, genetic differences and socioeconomic factors most likely contribute to these disparities [23]. Recently, research has confirmed a lower overall incidence of MOF [24]. The incidence of early single organ dysfunction has not changed but there has been a decrease in early MOF from 22 to 7%. The incidence of MOF in 1992 was 1.8 times the incidence in 2002 [25, 26]. A similar study of trauma patients by Durham also revealed a lower overall mortality for single organ failure as well as a decrease in the overall incidence of MOF [27].
Risk Factors for the Development of Organ Failure
MOF resides at the most severe end of a spectrum of illness that includes SIRS, sepsis, severe sepsis and septic shock. Any point along this constellation of criteria puts the patient at risk for MOF. The risks of organ failure are multiple and due to lack of consensus regarding a scoring system, it is difficult to ascertain which risk factors are most specific. MOF was originally thought to be catalyzed by an infectious process. While the majority of patients with MOF will have an infectious source, it is also known that MOF occurs without an infection, per se, and can be solely due to unregulated inflammation, as occurs with severe pancreatitis, trauma or burns [28]. Immunosuppression, pneumonia, blood transfusions and bacteremia are all associated with increased risk for developing sepsis, severe sepsis, or septic shock and therefore also increases a patient’s risk for MOF [29, 30].
A demographic risk factor for MOF includes advanced age. Advanced age, defined as greater than 65, has likewise been associated with worse quality of life indicators in survivors of sepsis. These patients more often require extensive rehabilitation as well as skilled nursing facility admission upon their hospital discharge from their acute septic event [31]. In a multivariate analysis, adjusted for age, sex, and severe head injury, patients with MOF had four times greater odds of requiring assistance from others in activities of daily living more than 2 years after trauma as compared to trauma patients without organ failure. There was no statistically significant difference regarding self-care between patients who did not have a history of organ failure when compared with those patients who had a history of a single organ failure [32]. Obese patients, in general, have been found to have higher post-traumatic morbidity and mortality. Obesity is defined as body mass index (BMI) >30 kg/m and as the BMI goes up, the incidence of MOF increases as well [33]. Moreover, when age, injury severity score (ISS), and transfusions are adjusted for, obesity is associated with an 80% increased risk of MOF [22, 34]. This is likely associated to the pro-inflammatory state that obesity confers to patients [35]. Additionally, patients with nonoperative diagnoses—for example, patients admitted postacute myocardial infarction—have also been found to have a higher likelihood of developing MOF [21].
In trauma patients, Balk and colleagues aptly identified several major risk factors for the development of postinjury MOF. These included prolonged periods of hypotension, trauma, bowel infarction, hepatic insufficiency, advanced age, and alcohol abuse [36]. Additionally, ISS, number of units of packed red blood cells transfused, base deficit, and lactate levels are all associated with an increased risk of developing MOF [37, 38] (Table 7.3). Blood transfusions have independently been shown to be predictors of SIRS, MODS and mortality [39]. Furthermore, Durham et al. also validated that total blood products infused in the first 24 h after injury in addition to higher Acute Physiology and Chronic Health Evaluation (APACHE) III scores, amplified the risk for MOF occurrence [27].
Table 7.3
MOF risk factors
Risk factors for MOF |
---|
•Hypotension |
•Trauma •Ischemic bowel •Pancreatitis •Advanced age >65 •Shock •Infection •Obesity •Alcohol abuse •Transfusion of blood products •Injury severity score ISS >25 •Immunosuppression •Base deficit >8 •Genetic factors |
•Lactate >2.5 |
Genetic factors also play a role in determining the severity and progression of organ failure. Genetic variants, particularly single-nucleotide polymorphisms (SNPs), are critical determinants for individual differences in both inflammatory responses as well as clinical outcomes in trauma patients [40]. Individuals who possess specific genetic polymorphisms in genes controlling the synthesis of cytokines or toll like receptors (TLR) may be predisposed to excessive inflammatory response to sepsis which increases their risk for the development of MODS [41]. For example, toll-like receptor 9 (TLR9) signaling plays an important role in the innate immune response. Trauma patients with SNPs of TLR9 have been found to have a greater responsiveness of their peripheral blood leukocytes as well as a higher risk of sepsis and multiple organ dysfunction [42]. Henckaerts and colleagues furthermore showed that these functional polymorphisms involved in innate immunity predispose patients to severe infections and death. Further study and elucidation could contribute to formation of a risk model where patients could be stratified as to who could benefit from specific preventative or therapeutic options [43].
Scoring Systems
MOF does not have a consensus definition and there are a variety of scoring systems used to categorize the severity of organ dysfunction. Trending these scores during a patient’s hospital course enables physicians to prognosticate the patient’s risk of mortality [44]. There is also a direct relationship between the number of organ failures and ICU mortality. Moreover, improvements in cardiovascular, respiratory and renal function during an ICU course can predict a better survival [45].
Scoring systems like the Acute Physiology and Chronic Health Evaluation (APACHE) score are based on measured laboratory values that enable staging of the severity of organ dysfunction. One of the most commonly used scoring systems is the Sequential Organ Failure Assessment Score (SOFA) (Table 7.4). Clinical and laboratory variables in six organ systems (respiratory, hematologic, liver, cardiovascular, central nervous system, renal) are utilized to calculate a total score [46]. Patients with no organ failure defined by a SOFA score below or equal to two for each organ at admission have an ICU mortality rate of 6% compared to 65–100% for those with four or more organ failures [34]. The Denver MOF score is also a frequently used and well validated score. It is defined as two or more organ systems failing greater than 48 h after injury. The Denver score looks at dysfunction in the cardiac, respiratory, renal and hepatic systems [47] (Table 7.5). When comparing the Denver postinjury MOF score with the SOFA score, the SOFA score is very sensitive but not as specific as the Denver MOF score, whereas the Denver postinjury MOF score is more specific and less sensitive than the SOFA score when dealing with the trauma population. This distinction is important when analyzing epidemiologic data as more sensitive scores will have a higher incidence of MOF, while a more specific score will have a higher mortality rate [48–50]. Regardless of what score is used to evaluate the various physiologic and clinical parameters, it is an underlying theme in all organ failure scores, that as the number of organ systems that are affected increase, so does the mortality [51, 52]. Moreover, these scoring systems were developed to quantify the severity of illness and the risk of mortality in ICU patients. These prognostic scores will not tell how a patient will respond to therapy and are best utilized to predict outcomes in certain homogenous groups of patients. Additionally, these scores are unable to provide details regarding how a patient will respond to treatment. However, they can be repeatedly assessed to evaluate a patient’s progress and used to identify patients for enrollment and to assess morbidity in clinical trials [53].
Table 7.4
SOFA score. MOF is defined as a score ≥4 with involvement of ≥2 organ systems
SOFA score | |||||
---|---|---|---|---|---|
System | Grade 0 | Grade 1 | Grade 2 | Grade 3 | Grade 4 |
Respiratory PaO2/FiO2 | >400 | <400 | <300 | <200 with respiratory support | <100 with respiratory support |
Coagulation platelets (Ã-103/mm3) | >150 | <150 | <100 | <50 | <20 |
Liver bilirubin (mol/l) | <20 | 20–32 | 33–101 | 102–204 | >204 |
Cardiovascular | No hypotension | MAP <70 mmHg | Dopamine >5 or any dobutamine dose | Dopamine >5 or epi_0.1 | Dopamine >15 or epi >0.1 |
Renal creatinine (mol/l) | <110 | 110–170 | 171–299 | 300–440 | >440 |
Central nervous system Glasgow Coma Scale | 15 | 13–14 | 10–12 | 6–9 | <6 |
Table 7.5
Denver postinjury multiple organ failure score
Denver postinjury multiple organ failure score | ||||
---|---|---|---|---|
Dysfunction | Grade 0 | Grade 1 | Grade 2 | Grade 3 |
Pulmonary PaO2/FiO2 ratio | >250 | 250–200 | 200–100 | <100 |
Renal creatinine (mol/l) | <159 | 160–210 | 211–420 | >420 |
Hepatic total bilirubin (mol/l) | <34 | 34–68 | 68–137 | >137 |
Cardiac | No inotropes | Only 1 ionotrope at small dose | Any ionotrope at moderate dose or >1 agent at small dose | Any ionotrope at large dose or >2 agents at moderate doses |
Clinical Presentation, Evaluation, and Diagnosis
The common clinical manifestations leading to multiple organ dysfunction are included in the ACCP-SCCM guidelines and can fall anywhere within the continuum of SIRS to MOF. These most commonly include alterations in body temperature (hyper or hypothermia), tachypnea or hypocarbia, tachycardia, leukocytosis, leukopenia or bandemia, hypotension, thrombocytopenia or coagulopathy, and alterations in mental status [54]. Fever is the most common presenting symptom of sepsis and should be an impetus for further evaluation the patient as well as identification of a source. Elderly patients with sepsis or those that are immunosuppressed may not mount a febrile response or conversely may be hypothermic [55]. In sepsis, common sites of infection are the pulmonary, gastrointestinal, and urinary tract systems. Other nosocomial causes of sepsis are intravenous catheter infections, ventilator-associated pneumonia, and sinusitis. As approximately 20% of patients will not have an identifiable source, noninfectious etiologies for SIRS should be considered [56]. These may include surgery, trauma, hematoma, subarachnoid hemorrhage, venous thrombosis, pancreatitis, myocardial infarction, transplant rejection, thyroid storm, acute renal or adrenal insufficiency, lymphoma, tumor lysis syndrome, transfusion reaction, opiates, benzodiazepines, anesthetic related malignant hyperpyrexia, and neuroleptic malignant syndrome [57].
A thorough physical examination should include a head-to-toe exam as well as inspection of indwelling catheters, a rectal exam, and examination of all wounds, including those under casts/fixation devices. Potential atypical causes of sepsis should be given consideration when an obvious source is identified. These potential causes of sepsis include sinusitis, meningitis, septic joint, acalculous cholecystitis, septic thrombophlebitis, deep muscular abscess, or a viral infection. Corresponding laboratory values based on the suspected differential diagnoses should be obtained.
Infections leading to sepsis can also arise in surgical sites from the skin to the deep muscle layers. Physical examination should be repeated if no source is identified. An investigation of all organ systems should be thorough and systematic. Subtle findings of end organ hypoperfusion such as altered mental status, tachypnea, hypoxia, hypotension, oliguria may be missed if the physician does not have a high index of suspicion and an incomplete exam is performed; i.e., failure to remove a dressing to inspect a wound. Failure to investigate thoroughly can lead to a delay in diagnosis and increased morbidity and mortality. Physical examination should include a rapid review of the patient’s hemodynamic condition and should include continuous monitoring. Patients in shock should have arterial catheters placed for blood pressure monitoring. Persistent clinical signs of SIRS may suggest ongoing inflammation or infection. In addition to the patient’s hemodynamic status, clinical signs of poor end organ perfusion, such as change in mental status, low urine output, mottling, and poor capillary refill, should be taken into consideration and used to guide resuscitation [58]. Initiation of resuscitation should take place immediately upon recognition of SIRS or sepsis symptoms and should not wait for transport to the next level of care.
Laboratory Evaluation
While no laboratory value will diagnose sepsis or MOF, they may assist in narrowing the differential diagnosis, localizing the source and guiding appropriate antibiotic therapy. Laboratory studies should include a complete blood count with differential, chemistry profile, arterial blood gas with lactic acid, prothrombin time and partial thromboplastin time, fibrinogen, and urinalysis [59]. Utilizing lactic acid level trends to guide resuscitation has been shown to be helpful in septic patients. For prognostication purposes, resolution of lactic acidosis with resuscitation efforts is associated with improved outcomes [60].
Pan cultures of the urine, blood, and sputum should be collected. The SCCM guidelines recommend that one pair of blood cultures be obtained at the onset of symptoms and another set obtained again at 24 h [12]. When taking blood cultures, two sets of blood cultures should be drawn from peripheral sites. If this is not possible, then one set should be drawn peripherally and the other from a recently inserted central catheter after careful cleansing of the port site. Every effort must be made to draw the first cultures before the initiation of antimicrobial therapy. They can be drawn consecutively or simultaneously, unless there is suspicion of an endovascular infection, in which case separate peripheral blood draws separated by timed intervals can be drawn to demonstrate continuous bacteremia [61].
Based on physical exam, additional body fluids may be sampled if the patient exhibits localized symptoms of infection. For example, cerebrospinal fluid, pleural fluid, joint aspiration, and ascites can all be sampled to localize the source of infection and help guide antibiotic therapy. Radiographic images should be tailored to the most likely source. If plain films are nondiagnostic, CT scans can assist in elucidating a suspected source and used to guide therapy, for example abscess drainage.
Pathophysiology
The pathophysiology of MOF is at best a nebulous interaction of multiple inflammatory mediators. Our understanding of this process and the innumerable interactions is in its infancy. A complete discussion of the immunology of this process is beyond the scope of this chapter as entire books have been dedicated to this task [62–64]. This section highlights some salient points regarding the pathophysiology of MOF.
Initially, SIRS was thought to be an overwhelming, uncontrolled response to infection. While MOF frequently is the end point of the spectrum of SIRS and severe sepsis, severe inflammation is also a mitigating factor and can result in the same endpoint of organ failure. This indicates overlap in the pathophysiology between inflammation and infection. The progression to MOF from SIRS from either cause is likely the result of an unbalanced interaction between the pro and anti inflammatory mediators. In most patients, the initial SIRS response is physiologically followed by a compensatory anti-inflammatory response syndrome (CARS). This acts to limit the SIRS response so that it is not counterproductive. The subsequent balance between the pro-inflammatory (SIRS) and anti-inflammatory (CARS) response has been referred to as the mixed antagonistic response syndrome or MARS [36]. If the balance of these two systems is disturbed the inflammatory response becomes systemic and deregulated. The result is whole-body activation of the inflammatory response, with resultant disruption of normal cellular metabolism and microcirculatory perfusion. Both of these responses, if unchecked can result in complications, the former leading to MOF and the later secondary infections. At the site of injury, endothelial cells and leukocytes coordinate the local release of mediators of the inflammatory response, including cytokines interleukins, interferons, leukotrienes, prostaglandins, nitric oxide, reactive oxygen species, and products of the classic inflammation pathway. It is this usually functional biologic response that becomes unregulated and leads to MOF [65].
In 1996, Moore and colleagues recognized MOF is not necessarily related to an infectious process and follows a bimodal distribution. Early MOF is now defined as organ failure that develops within 72 h of the initial diagnosis of sepsis (Table 7.6). Late MOF was defined as organ failure that develops after 72 h after the initial diagnosis of sepsis [66]. When compared to the late MOF group, patients with early organ failure died sooner, had more cardiac dysfunction and had greater evidence of hyper inflammation. In contrast, patients with late MOF were older, had greater evidence of hepatic failure, and were more likely to have an infection as a “second hit” [67].
Table 7.6
Risk factors for early and late MODS
Risk factors for early MODS <72 h of injury | Risk factors late MODS >72 h after injury |
---|---|
•ISS > 24 | •Age > 55 |
•SBP < 90 | •>6 units of blood transfused within 12 h of injury |
•>6 units of blood transfused within 12 h of injury | •Base deficit >8 mEq/l within first 12 h of injury |
•Lactate > 2.5 | •Lactate > 2.5 mmol/l within 12–24 h of injury |
Multiple theories exist regarding the cause for MOF and it is likely that these pathways overlap to cause initially organ insufficiency that, unless reverses, ultimately leads to failure. Four overlapping categories have been proposed to the complex pathophysiology of MOF. These are the cytokine hypothesis, the microcirculatory hypotheses, the gut hypothesis and the two-hit hypothesis [63].
The Cytokine Hypothesis of MOF
In the cytokine hypothesis, the immune response to infection or inflammation results in excessive or prolonged activation or stimulation of mediators. These include interactions between polymorphonuclear neutrophils (PMNs), endothelial cells, and macrophages. PMN stimulation results in “priming” of the neutrophil and can lead to overzealous production, surface expression, and liberation of cytokines [68]. These mediators often have an exaggerated response and the products of these cascades exert damaging local and systemic effects. A temporal relationship between cytokine production and time of injury was recognized. Cytokines predictive of MOF in trauma patients include inducible protein (IP)-10, macrophage inflammatory protein (MIP)—1B, interleukin (IL) IL-10, IL-6, IL-1Ra, and eotaxin [69]. Several lines of evidence support the central role of inflammatory cells in the pathogenesis of lung and systemic organ injury. Tumor necrosis factor (TNF) has been considered one of the most potent pro-inflammatory cytokines identified in SIRS and sepsis. Administration of TNF to experimental animals creates the hemodynamic and metabolic observations consistent with SIRS. Analysis of cytokine serum biomarkers has shown that patients with MOF show a biphasic elevation of IL-6 and significantly higher soluble TNF receptor (sTNF-R) concentrations [70]. Activation of leucocytes and their subsequent inappropriate sequestration in organs appears to additionally be one of the key events in the development of early MOF. Once activated, leukocytes have the capacity to release their cytotoxic factors including nitric oxide and lysosomal granules, which aid in polymicrobial killing. These factors can cause necrosis and inflammation of organs such as the lung despite a lack of an infectious stimulus [71]. Additionally, PMN stimulation provokes endothelial and epithelial injury through up-regulation of adhesion molecules on these cells. This prompts changes in the cell wall, increased permeability cell swelling and culminates in cellular dysfunction. Neutrophil elastase is a key marker of severity of injury and has also been found to be a prognostic marker [72].
The Microcirculatory Hypothesis of MOF
The microcirculatory hypothesis proposes that organ injury is related to ischemia or vascular endothelial injury [73]. Some authors have speculated that even though adequate blood flow may reach the various tissue beds, there may be an inability of the mitochondria or cells to take up or use the delivered oxygen and substrate. Although prolonged tissue hypoperfusion and hypoxia leads to inadequate adenosine triphosphate (ATP) generation and potentially irreversible cell damage, this shock period is not long enough in most clinical conditions for that to occur. This damage is relieved by reperfusion and thus pro-inflammatory factors and oxygen radicals are introduced and lead to injury [74]. In vitro studies have found that nitric oxide (NO) up-regulates the production of pro-inflammatory cytokines (TNF-alpha, IL-8 and prostaglandins) and can lead injury of the lung, and intestine. Additionally, the superoxide anion and hydrogen peroxide can interact with NO and form peroxynitrite, which is toxic to cells [72]. During shock, these mediators, such as reactive oxygen species, are released to destroy the offending bacteria and to inactivate toxins. The unintended effects are that when unregulated, they also result in damaging the patient’s organ systems [75].
Gut Hypothesis of MOF
The gut is considered an immunologically active organ and a main in the burden of infection-induced systemic inflammation [76]. Gut barrier dysfunction can occur for a variety of reasons including trauma, shock, infection, and malnutrition. It is proposed that, as a result of the loss of the gut barrier function, intestinal bacteria and endotoxin cross the mucosal barrier and lead to exposure of the intestinal immune cells. The production of gut-derived toxins and inflammatory products reach the systemic circulation through the intestinal lymphatics, leading to SIRS, ARDS, and MOF [68]. These translocating bacteria are phagocytosed by intestinal immune cells and contribute to the intestinal inflammatory response. Some of these translocating bacteria or their toxic products are trapped in the intestinal lymph nodes, causing inflammatory reaction [72]. This hypothesis is supported by the demonstration of circulating levels of endotoxin in the peripheral blood of critically ill patients with sepsis and SIRS. Reports of endotoxemia in these critically ill patients, even without clinical or microbiologic evidence of infection with gram-negative organisms supports the potential role of translocation in the production of MODS/MOF [36]. The phenomenon of bacterial translocation, however, is not sufficient to explain the development of MODS in ICU patients. The development of MODS in these high-risk patients is likely due to intestinal injury and the resultant inflammatory cascade that reaches the systemic circulation via the intestinal lymphatics [77].
Two-Hit Phenomenon in MOF
The phrase “two-hit phenomenon in MOF” is used to describe the biologic phenomenon in which an initial insult primes the host such that on a second or subsequent insults, the host’s response is greatly amplified. Primers to the subsequent insult can be infection, shock, inflammation, or trauma. Despite the decreasing incidence of MOF, the rate of PMN priming has not changed. PMN priming increases elastase release, IL-8 production, L-selectin expression, and CD-18 expression, and delays apoptosis. This is evident by a lack of change in the incidence of early lung dysfunction postinjury, which is a surrogate marker of PMN priming [78]. The timing of the second hit phenomenon was shown in laboratory experiments evaluating abdominal compartment syndrome (ACS). If subjects had early decompressive laparotomy (<2 h) or late (>18 h), they had a lower mortality than those having a decompressive laparotomy at 8 h. This correlates with the clinically identified time frame of the development of postinjury ACS, which manifests 8–12 h window after trauma. Severely injured patients who develop ACS have a fourfold increase in their chance of developing MOF compared to the non-ACS patients with similar demographics, shock parameters and injury severity [24]. These insults prime the immune system to mount an exaggerated response when exposed to a second physiologic insult. Botha described the observation that the first hit primes and activates PMNs within 3–6 h after injury. This primer creates a vulnerable window during which a second insult activates excessive cytokine release. This second hit results in an elevated risk of developing MOF [79]. This exaggerated immune response then results in end organ injury [80]. In summary, MOF results from an excessive host response to an infectious or inflammatory stimulus. Any or all of the aforementioned hypotheses can coexist and each overlaps with the other. The cytokine, endovascular, and systemic storm that ensues thereafter, predisposes to additional infections and can lead to organ failure [45].
The temporal series of events in MOF is usually predictable and is independent of the etiology. Multiple studies have demonstrated that the respiratory system is usually the first to fail and is the most commonly affected [15]. This is typically followed by hepatic, intestinal, and renal failure, in that order. As the number of organ systems affected increases from 1 to 4, the mortality increased from 21 to 100% [81]. Hematologic and myocardial failures are usually later manifestations of MOF, whereas the onset of central nervous system alterations can occur either early or late [24]. Physiologically, these patients are hyper metabolic and they have a hyper dynamic circulation, which is characterized by an increased cardiac output and a decreased systemic vascular resistance. This classical sequential pattern of organ failure may be modified, however, by the presence of preexistent disease or by the nature of the precipitating clinical event. For example, renal failure may precede hepatic or even pulmonary failure in patients with intrinsic renal disease or in patients who have sustained prolonged periods of shock, whereas hepatic or myocardial failure may be an early or even the initial manifestation of this syndrome in the patient with cirrhosis or myocardial damage [82]. The exact sequence of organ failure, however, is not always predictable and can be influenced by the patient’s preexisting morbidities as well as their acute process. However, as the number of organs that fails increases from one to four, the mortality rate progressively increases from 30 to 100% [27].
Multiple Organ Failure by System
Pulmonary Dysfunction
The sequence of organ dysfunction is predictable and the lung is usually the first organ to show signs of failure. Initial pulmonary insufficiency and renal impairment are followed by circulatory failure and then metabolic dysfunction and liver failure. Respiratory failure can range from mild hypoxia and tachypnea to ARDS [83]. ARDS is defined as a PaO2/FIO2 ratio lower than 200 mmHg in association with bilateral fluffy pulmonary infiltrates and a pulmonary capillary wedge pressure lower than 18 mmHg [84]. Increased capillary permeability and neutrophil influx are the earliest pathologic events in ARDS. As the acute inflammatory process resolves, further lung injury results both from the process of repair, which involves fibrosis and the deposition of hyaline material, and from further lung trauma, resulting from positive pressure mechanical ventilation [85]. ARDS may occur within a few days of admission or after the development of SIRS and sepsis. Sepsis-induced ARDS is associated with the highest mortality rates. Additionally, the data suggests that approximately 40% of patients with severe sepsis develop ARDS. Historically, 10–12 ml/kg tidal volumes were commonplace and resulted in alveolar damage due to over distention. Parenchymal injury appears to be due primarily to oxidative damage from the activated neutrophils in the lung. Endotracheal intubation and a controlled mode of ventilation are the mainstays of support for respiratory failure. Lung protection ventilation strategies, with low tidal volumes (4–6 ml/kg) for patients with ARDS, are recommended and showed a decreased mortality from 40 to 31%. Due to the smaller tidal volumes, patients typically will have a rise in carbon dioxide [86]. This permissive hypercapnia has been shown to have a protective effect in critically ill patients [87]. Some patients with refractory hypoxemia may require alternative therapies such as extracorporeal membrane oxygenation (ECMO), high-frequency oscillation, or inhaled nitrous oxide.
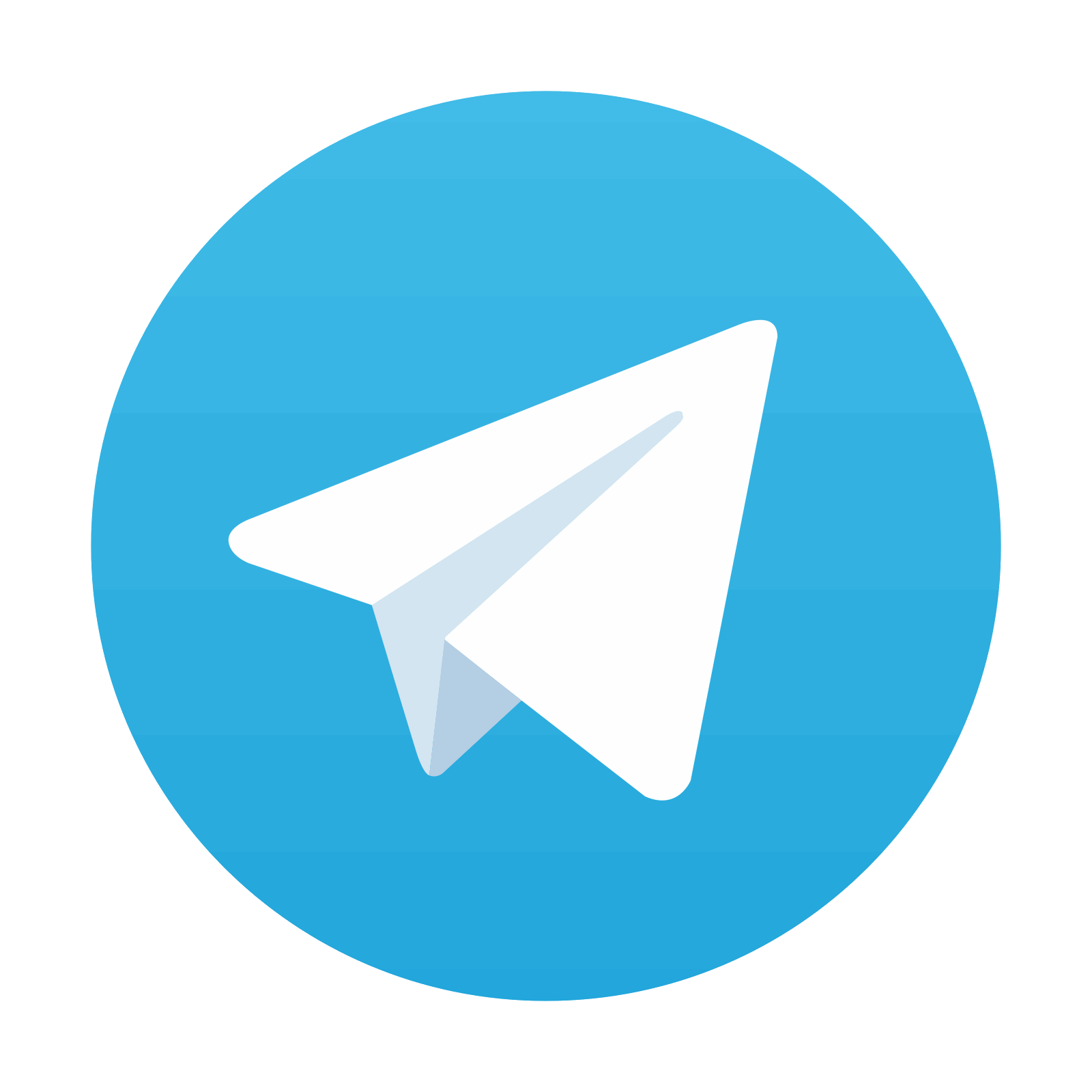
Stay updated, free articles. Join our Telegram channel

Full access? Get Clinical Tree
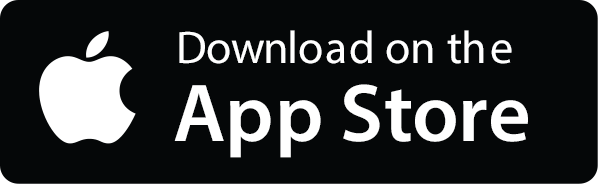
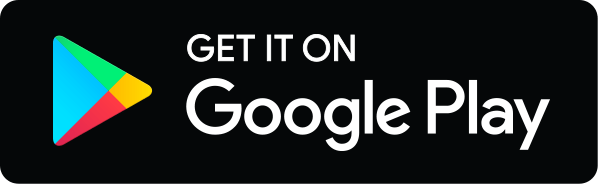