C. Lipid versus Protein Targets. Anesthetics might interact with several possible molecular targets to produce their effects on the function of ion channels and other proteins.
D. Lipid Theories of Anesthesia. The lipid theory of anesthesia postulates that anesthetics dissolve in the lipid bilayers of biological membranes and produce anesthesia when they reach a critical concentration in the membrane. Yet no lipid theory can plausibly explain all anesthetic pharmacology, and most investigators do not consider lipids as the most likely target of general anesthetics.
E. Protein Theories of Anesthesia. The Meyer-Overton rule could also be explained by the direct interaction of anesthetics with hydrophobic sites on proteins.
1. Direct interactions of anesthetic molecules with proteins not only satisfies the Meyer-Overton rule but would also provide the simplest explanation for compounds that deviate from this rule.
2. Protein-binding sites for anesthetics could also explain the convulsant effects of some polyhalogenated alkanes.
IV. HOW DO ANESTHETICS INTERFERE WITH THE ELECTROPHYSIOLOGIC FUNCTION OF THE NERVOUS SYSTEM? The functional unit of the central nervous system (CNS) is the neuron. Ultimately, general anesthetics must disrupt the function of neurons mediating behavior, consciousness, and memory. Anesthetics could accomplish this by altering the intrinsic firing rate of individual neurons (neuronal excitability) or by altering communication between neurons (synaptic transmission).
A. Neuronal Excitability. Anesthetics can hyperpolarize (create a more negative resting membrane potential) both spinal motor neurons and cortical neurons; this ability to hyperpolarize neurons correlates with anesthetic potency. Although current data still support the prevailing view that neuronal excitability is only slightly affected by general anesthetics, this small effect may nevertheless contribute significantly to the clinical actions of volatile anesthetics.
B. Synaptic Transmission. Synaptic transmission is widely considered to be the most likely subcellular site of general anesthetic action. Neurotransmission across both excitatory (not all excitatory synapses are equally sensitive to anesthetics) and inhibitory synapses is markedly altered by general anesthetics.
1. Presynaptic Effects. Neurotransmitter release from glutamatergic synapses has consistently been found to be inhibited by clinical concentrations of volatile anesthetics.
2. Postsynaptic Effects. Anesthetics alter the postsynaptic response to released neurotransmitters at a variety of synapses.
V. ANESTHETIC ACTIONS ON ION CHANNELS. Ion channels are a likely target of anesthetic action.
A. Anesthetic Effects on Voltage-Dependent Ion Channels. A variety of ion channels can sense a change in membrane potential and respond by either opening or closing their pores.
1. Voltage-dependent calcium channels serve to couple electrical activity to specific cellular functions (respond to action potentials by opening). This allows calcium to enter the cell, activating calcium-dependent secretion of neurotransmitter into the synaptic cleft.
2. Potassium channels are the most diverse of the ion channel types and include voltage-gated (background or leak channels) that open over a wide-range of voltages.
B. Anesthetic Effects on Ligand-Gated Ion Channels. Fast excitatory and inhibitory neurotransmission is mediated by the actions of ligand-gated ion channels. Synaptically released glutamate or GABA (γ-aminobutyric acid) diffuse across the synaptic cleft and bind to channel proteins that open as a consequence of neurotransmitter release. The ligand-gated ion channels provide a logical target for anesthetic action because selective effects on these channels could inhibit fast excitatory synaptic transmission or facilitate fast inhibitory synaptic transmission.
1. Glutamate-Activated Ion Channels (AMPA [AMPA (α-amino-3-hydroxy-5-methyl-4-isoxazolepropionic acid] receptors, kainate receptors, NMDA [N-methyl-D-aspartic acid] receptors)
a. AMPA and kainate receptors are relatively nonselective monovalent cation channels involved in fast excitatory synaptic transmission.
b. NMDA channels conduct not only Na+ and K+ but also Ca++ and are involved in long-term modulation of synaptic responses (long-term potentiation). NMDA-activated currents also appear to be sensitive to a subset of anesthetics.
c. Ketamine is a potent and selective inhibitor of NMDA-activated currents. Ketamine stereoselectively inhibits NMDA currents by binding to the phencyclidine site on the NMDA receptor protein.
d. NMDA receptors may also be an important target for nitrous oxide and xenon.
2. GABA-Activated Ion Channels. GABA is the most important inhibitory neurotransmitter in the mammalian CNS.
a. GABA-activated ion channels (GABAA receptors) mediate the postsynaptic response to synaptically released GABA by selectively allowing chloride ions to enter and thereby hyperpolarize neurons. GABAA receptors are multisubunit proteins consisting of various combinations of α, β, δ, and ε subunits, and there are many subtypes of each of these subunits.
b. The function of GABAA receptors is modulated by a wide variety of pharmacologic agents, including convulsants, anticonvulsants, sedatives, anxiolytics, and anesthetics.
c. Barbiturates, anesthetic steroids, benzodiazepines, propofol, etomidate, and the volatile anesthetics all modulate GABAA receptor function (potentiation, direct gating, inhibition).
d. Despite the similar effects of many anesthetics on GABAA receptor function, different anesthetics act on distinct subtypes of GABAA receptors (benzodiazepine sensitivity requires the presence of the γ 2 subunit subtype; sensitivity to etomidate requires the presence of a β 2 or β 3 subunit).
3. Other Ligand-Activated Ion Channels. Ligand-gated receptors structurally similar to the GABAA receptor, including the nicotinic acetylcholine receptors (muscle and neuronal types), glycine receptors, and 5-HT3 receptors, have been shown to be modulated by general anesthetics.
a. Glycine is an important inhibitory neurotransmitter, particularly in the spinal cord and brainstem (volatile anesthetics potentiate glycine-activated currents). The glycine receptor is a member of the ligand-activated channel superfamily that, similar to the GABAA receptor, is a chloride-selective ion channel.
b. The 5-HT3 receptors are also members of the genetically related super family of ligand-gated receptor channels (volatile anesthetics potentiate currents activated by 5-hydroxytryptamine). The 5-HT3 receptors may play some role in the anesthetic state produced by volatile anesthetics and may also contribute to some unpleasant anesthetic side effects such as nausea and vomiting.
VI. HOW ARE THE MOLECULAR EFFECTS OF ANESTHETICS LINKED TO ANESTHESIA IN THE INTACT ORGANISM?
A. Pharmacologic Approaches. An experimental paradigm frequently used to study anesthetic mechanisms is to administer a drug thought to act specifically at a putative anesthetic target (a receptor agonist or antagonist, an ion channel activator or antagonist) and then determine whether the drug has either increased or decreased the animal’s sensitivity to a given anesthetic. Development of specific antagonists for anesthetic agents would provide a major tool for linking anesthetic effects at the molecular level to anesthesia in an intact organism and might also be of significant clinical utility.
B. Genetic Approaches. An alternative approach to study the relationship between anesthetic effects observed in vitro and whole-animal anesthesia is to alter the structure or abundance of putative anesthetic targets and determine how this affects whole-animal anesthetic sensitivity.
1. There has been a focus on a novel presumptive cation channel, NCA-1/NCA-2, that controls halothane sensitivity in both Caenorhabditis elegans and the fruit fly Drosophila.
2. In mammals, the most powerful genetic model organism is mouse, in which techniques have been developed to alter or delete any gene of interest. The GABAA receptor has been extensively studied using mouse genetic techniques.
VII. WHERE IN THE CENTRAL NERVOUS SYSTEM DO ANESTHETICS WORK?
A. Immobility. Evidence indicates that the spinal cord is the main site at which anesthetics act to inhibit movement in response to noxious stimulation. This is the end point used in most measurements of anesthetic potency. MAC values for fluorinated volatile anesthetics are unaffected in rats by either decerebration or cervical spinal cord transection.
B. Autonomic Control. Anesthetics exert profound effects on homeostatic mechanisms through effects on autonomic centers in the brainstem. Cardiovascular perturbations are also mediated in part at autonomic centers. Hypothalamic thermoregulation is also ablated by anesthetics.
C. Amnesia. Although the neurobiological mechanisms underlying learning, memory consolidation, and memory storage remain unclear, the hippocampus and the amygdala are plausible anesthetic targets for suppressing memory formation.
FIGURE 5-2. Diagram of subcortical arousal circuitry implicated in anesthetic-induced unconsciousness. The tuberomammillary nucleus (TMN) and ventrolateral preoptic nucleus (VLPO) form a bistable sleep/wake switch. Orexin (Ox) release from the perifornical (PF) area stabilizes the VLPO–TMN sleep switch and plays a key role in modulating outputs from several areas. Ach, acetylcholine; DA, dopamine; His, histamine; GABA, γ -aminobutyric acid; LC, locus coeruleus; NMB, nucleus basalis of Meynert; nor, norepinephrine; VTA; ventral tegmental area.
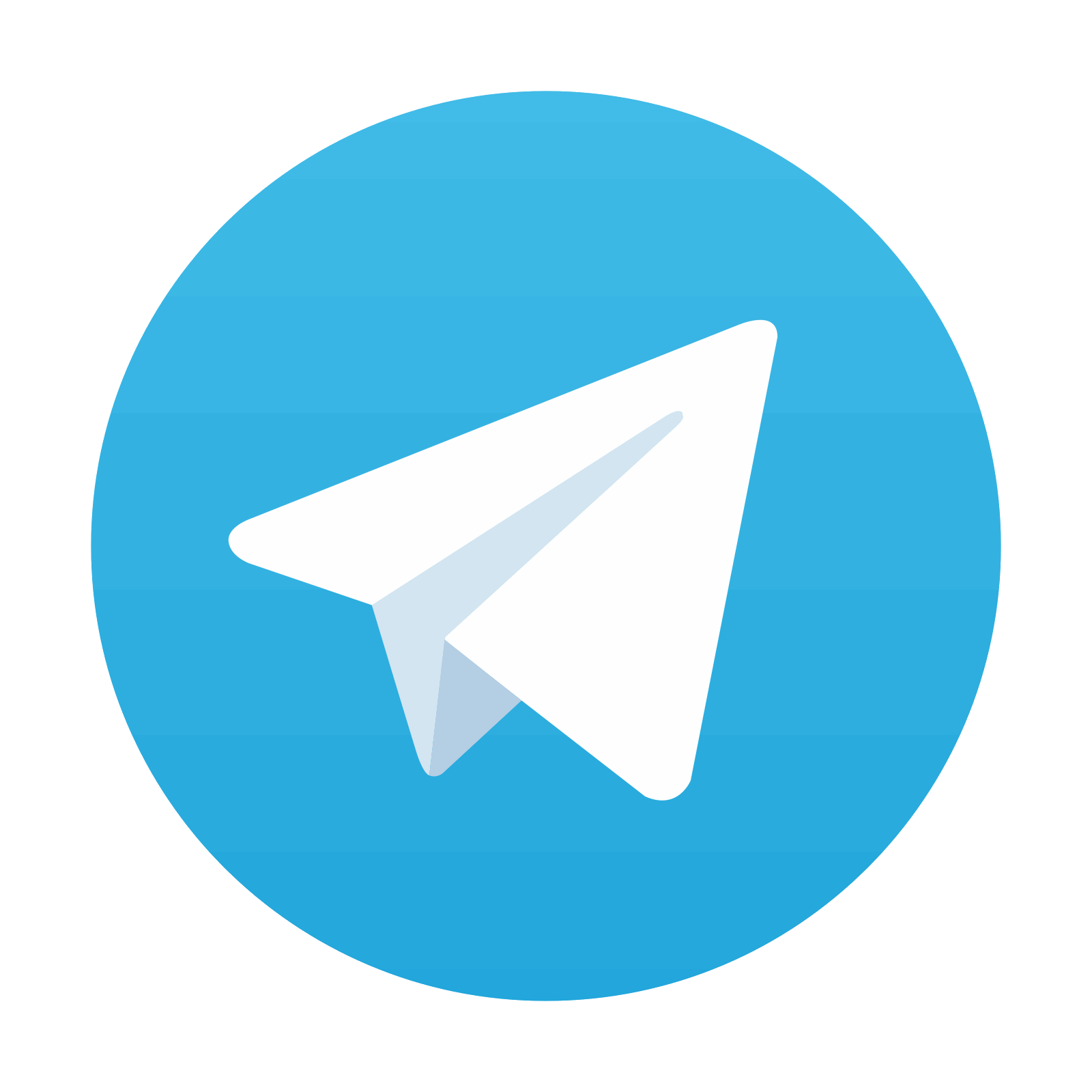
Stay updated, free articles. Join our Telegram channel

Full access? Get Clinical Tree
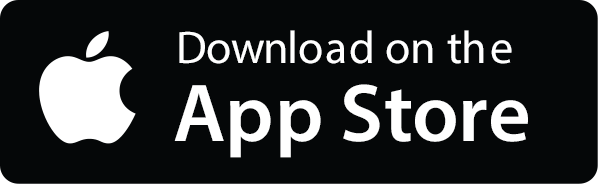
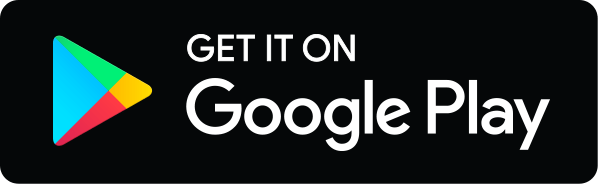