211 Management of the Brain Dead Organ Donor
Transplantation is an increasingly utilized treatment option for patients with organ failure. In 2009, 28,465 organs were transplanted in the United States, with over 100,000 patients on the waiting list.1 Despite advances in immunosuppression and postoperative management, utility of transplantation is dependent on the number of available organs. Expanding indications for transplantation have further widened the gap between supply and demand.
To address this problem, the U.S. Department of Health and Human Services launched the Organ Donation Breakthrough Collaboratives in 2003 with the intent to increase the number of donors, as well as number of organs transplanted per donor. Events in the pathway of organ donation are illustrated in Figure 211-1.2 Maintenance of allocated organs was identified as a major area for improvement. The majority of donor organs are cadaveric, of which 90% are from brain dead (BD) donors. An estimated 20% to 30% of organs are lost prior to procurement despite aggressive measures in BD donors.1 This number attests to the profound physiologic variations that occur at the time of brain death but can also be attributed to what can often be suboptimal unstandardized care.3 There is a large disparity between the intensive team-based management of the trauma or stroke victim and the singularity of the organ procurement organization (OPO) coordinator left at the bedside once brain death is declared. The intensivist can therefore have a profound impact on number and quality of organs salvaged.
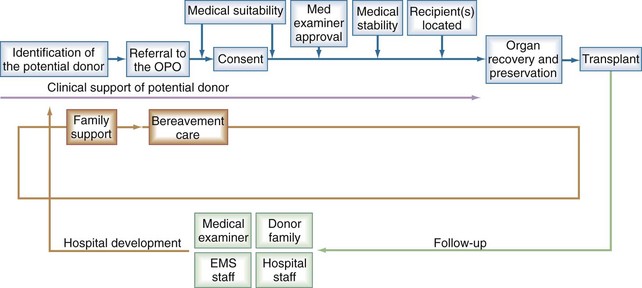
Figure 211-1 Events in the organ donation and consent process.
(From Organ Donation Breakthrough Collaborative best practices final report, September 2003. The Organ Donation Breakthrough Collaborative. Best practices final report; U.S. Department of Health and Human Services Health Resources and Services Administration; Office of Special Programs, Division of Transplantation Contract: 240-94-0037 Task Order No. 12, September 2003.)
Declaration of Brain Death
The initial process for organ donation requires heightened awareness on the part of the intensive care unit (ICU) team. Often, potential donors are excluded by the caregiver based on notions of donor criteria or concerns regarding conflict of care. Members of the local OPO are trained specifically to interact with families regarding donation issues in such a manner that the caregiver and OPO are not seen in mutual opposition. With the permission of the family, blood sampling to determine the suitability may be performed before brain death occurs.4 Once brain death is confirmed by standard criteria (see Chapter 219), the team needs to act quickly to stabilize the physiology of the donor and shorten time to transplantation.
Physiology of Brain Death
Brain injury resulting in herniation will follow a rostrocaudal progression of ischemia. Events leading up to brain death include hypertension with bradycardia (Cushing response) as the pons becomes ischemic. Further involvement of the medulla creates unopposed sympathetic stimuli, initiating a catecholamine “storm.” This surge of catecholamines damages end organs both from severe vasoconstriction and from the proinflammatory response elicited. Finally, spinal cord ischemia and loss of sympathetic denervation results in severe hypotension. Simultaneous ischemia to the pituitary and hypothalamus exacerbate this with loss of homeostatic control. These events occur in varying magnitude or velocity, making management even more difficult. The resulting physiology is characterized by hemodynamic instability with a host of secondary complications listed in Figure 211-2.
Initial Donor Resuscitation
Care of the BD donor requires multitasking and frequent reassessment. Donors often have associated traumatic injury and chronic health problems. To complicate matters, treatment strategies prior to brain death are directed toward maintaining cerebral perfusion, often to the detriment of other organs. Post-declaration management focuses on reversing this state and preventing further organ damage. Expeditious stabilization is paramount, as graft loss rapidly increases after 48 hours.5
Various organizations provide algorithms for standard management of the BD donor. Protocols may be organ specific, or target the donor as a whole.6–10 The United Network for Organ Sharing (UNOS) provides a sample standard pathway that includes initial workup as well as therapy (Figure 211-3). These algorithms help focus ongoing resuscitation, ensure provision of evidence-based therapy, and provide a platform for future research in the field.
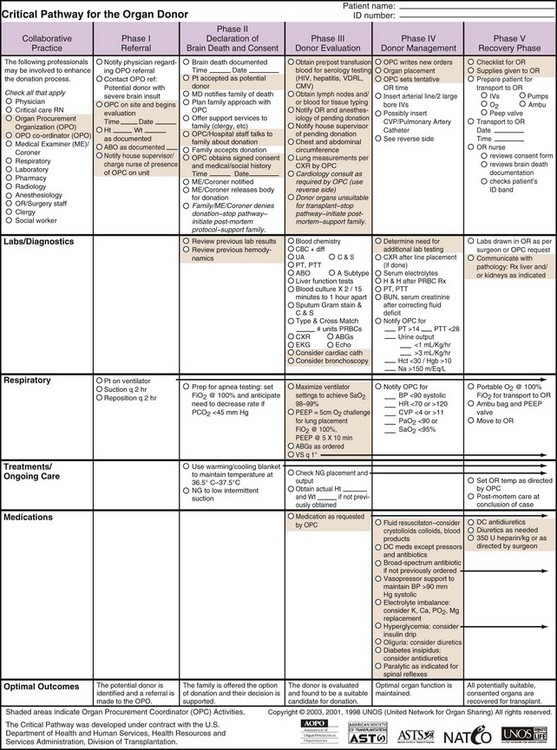
Figure 211-3 Critical pathway for organ donor.
(Reprinted with permission of UNOS, Richmond, Virginia. Access at http://www.unos.org/docs/cntical_Pathway.pdf.)
Immediate goals are establishing baseline organ function and stabilizing physiology. If not already in place, a central venous catheter and arterial catheter are inserted. Blood, urine, and bronchial cultures are obtained and baseline chemistries performed. Evaluation of the lung and heart begin with basic chest x-ray, echocardiogram, bronchoscopy, and coronary angiogram as indicated.7 Blood type and crossmatch are performed, and initial graft allocation efforts begun by the OPO coordinator.
Initial resuscitation includes crystalloid administration guided by central venous pressure or pulmonary artery pressure, although pulse pressure variation (PPV) may actually predict preload responsiveness more accurately.11 After adequate volume loading, vasopressors are often required to maintain perfusion pressure. Monitoring end organ perfusion may be achieved by measuring oxygen delivery or central venous oxygen saturation.12 Other endpoints of resuscitation are listed in Box 211-1.
Standard ICU protocols should be employed to prevent further complications. Gastrointestinal and deep vein thrombosis (DVT) prophylaxis should be continued appropriately, blood products administered for anemia or coagulopathy, aspiration precautions upheld, and electrolytes and acidosis corrected to avoid arrhythmias. Insulin therapy should be given, as it has antiinflammatory properties that may be particularly beneficial in the BD donor.13,14 A multidisciplinary approach greatly helps coordinate care.
Specific Considerations and Controversies
Cardiovascular
Cardiovascular management after brain death is paramount to maintaining perfusion and preserving the heart for donation. Hemodynamic collapse occurs more from loss of afterload than primary nonfunction of the heart.15 Nevertheless, the catecholamine surge during herniation can incite considerable myocardial damage.16 Right ventricle strain is common secondary to increased pulmonary capillary perfusion and pulmonary overflow injury from increased vascular resistance.17 Contractility must be frequently reassessed and quantified with echocardiography, as regional wall abnormalities will often resolve.18 Most important to cardiac function is coronary perfusion pressure, which can be affected by loss of autoregulatory reserve after brain death.19–21
Protocols based on traditional volume and vasopressor management have increased the number of donor hearts.8,22 These protocols included moderate crystalloid resuscitation followed by catecholamine use for hypotension and hormone treatment when cardiac dysfunction was diagnosed (Figure 211-4).23 Since their development, certain details have been debated, primarily hormone treatment (discussed later) and choice of vasopressor.
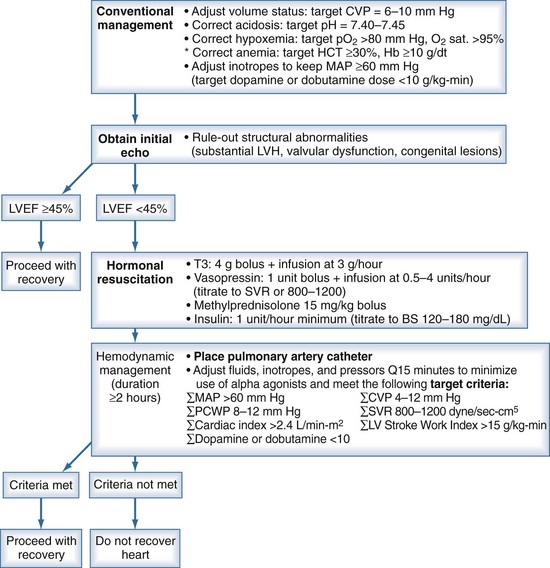
Figure 211-4 Recommendations for cardiac donor management.
(Adapted from Rosengard BR, Feng S, Alfrey EJ, Zaroff JG, Emond JC, Henry ML et al. Report of the Crystal City meeting to maximize the use of organs recovered from the cadaver donor. Am J Transplant 2002;2:701-11.)
Types of vasopressors advocated include dopamine, epinephrine, and norepinephrine as well as vasopressin.7,24–27 Immunomodulatory function of the catecholamines makes them attractive in the context of the donor’s proinflammatory condition.28–30 Dopamine-stimulated induction of heme-oxygenase-1 makes kidneys more resistant to ischemic-reperfusion injury in donor models.31,32 Dopamine may suppress anterior pituitary hormones, however, and is likewise notorious for inducing tachyarrhythmias.33,34 Norepinephrine and epinephrine have been related to cardiac and kidney graft nonfunction.35–37 Vasopressin makes sense in the face of posterior pituitary ischemia and can reduce the dose of catecholamines administered.38,39
Pulmonary
Like the heart, lung function in the donor can be affected by physiologic changes with brain death, in addition to underlying pulmonary disease. Pulmonary edema after brain death results from elevated afterload from the catecholamine surge combined with increased venous return and decreased left ventricular function.40 The sympathetic discharge also up-regulates inflammation in the lung parenchyma and capillaries, leading to further edema and failure.41 These effects are significant because pulmonary edema and inflammation reduce lung donation rates to less than 20%.42
Standard criteria for lung donation include a clear chest x-ray and PaO2/FIO2 above 300, although with expanded donor criteria, these parameters are viewed as too strict.43,44 Findings such as edema and atelectasis can be reversed with adequate diuresis and recruitment maneuvers.45,46 Global oxygenation does not represent unilateral oxygenation, and the single-lung donor pool can therefore be expanded by obtaining unilateral pulmonary vein gases instead of relying on PaO2.47,48
Once a suitable donor is identified, aggressive management with a lung-specific focus is pursued.6 Most protocols use frequent chest physiotherapy and bronchoscopy, diuretics, strict aspiration precautions, empirical antibiotics, and steroid administration.49–51 Lung recruitment maneuvers and frequent bronchoscopy increase oxygenation and lung utilization.52 Diuretics are given to decrease central venous pressure (CVP) in an effort to decrease alveolar-arterial oxygen gradient, although restricting CVP does not necessarily increase lung utilization.53–55
Empirical antibiotics are generally administered based on chest x-ray findings. Although culture obtained from bronchoalveolar lavage (BAL) is the gold standard, results often are not rapid enough for specific antibiotic tapering.56 Even when bronchial culture is obtained, there is poor correlation between culture data and posttransplant pneumonia development, with an 8% transmission rate despite appropriate antibiotics.57 Steroids are also widely employed in lung donors in an effort to decrease lung water accumulation and enhance alveolar fluid clearance.58,59
Whereas there are few studies about ventilator mode, pressure-cycled modes are being used more frequently for donors.60 Lung protective strategies using low tidal volumes and moderate positive end-expiratory pressure (PEEP) can prevent further barotrauma.61,62 For the donor population specifically, acute lung injury is more common in those treated with increased tidal volumes, and sustained recruitment maneuvers should be used with caution.63 Excessive oxygen administration should likewise be avoided, as this can induce the inflammatory cascade and apoptosis.64
Renal
BD donors are typically volume depleted secondary to aggressive mannitol use and diabetes insipidus. Strategies for preventing further renal injury include avoidance of nephrotoxic agents and maintaining hydration. Larger amounts of volume administration can improve kidney and liver graft function by correction of hypernatremia.36,65,66 By contrast, hypervolemia is also deleterious, inducing right heart strain and lung dysfunction.45 Restricting CVP to improve lung function, however, did not adversely affect kidney graft function in a recent well-designed study.55
Crystalloids are primarily used for initial resuscitation. Some societies advocate colloids to avoid lung water accumulation, but data to support this are limited.53 Hydroxyethylstarch (HES) can generate nephrosis-like lesions and impair graft function in kidneys, although newer, less osmotic formulations do not demonstrate the same detrimental effects.67–69 Hypertonic saline may modulate inflammation and shows promise for donor resuscitation, although sodium levels should be monitored closely.70,71
Endocrine
One of the more debated aspects of donor management is the use of hormonal therapy. The donor suffers from a variable panhypopituitary state secondary to ischemia.72 Dysfunction of the posterior pituitary is common (90%), with resultant low to nil vasopressin levels.73 Administering desmopressin treats the subsequent diabetes insipidus that can further complicate fluid management. Dysfunction of the anterior pituitary is less consistent, with variable effects of hormones given to counteract the loss of corticotropin (ACTH) and thyroid-stimulating hormone (TSH).74 In animal models, levels of triiodothyronine (T3), cortisol, and insulin are all markedly decreased.75 Humans, however, exhibit near-normal levels of cortisol and insulin, with nonuniform decreases in T3.73 Hypophyseal blood flow may be maintained by branches off of the external carotid and could explain some of these variable hormone alterations.
Initial enthusiasm for hormone replacement therapy (HRT) was based on non-randomized data showing increased organ yield when a cocktail of T3, steroids, insulin, and vasopressin was administered.9,22,76,77 Hormone cocktails therefore became part of the UNOS protocol for cardiac donor management. Using this protocol, animal models demonstrated beneficial reduction in vasopressors when given HRT.78 With more rigorous examination, however, combination hormone therapy has not been supported. A recent randomized control trial using HRT in a protocol to increase lung donors was unable to demonstrate increased organ yield in those receiving the cocktail.79 Criticism of HRT focuses primarily on the thyroid and steroid components of therapy, and thus will be examined more closely.
Thyroid
Thyroid hormone replacement for donors originated with baboon studies in the late 1980s. Novitsky et al. observed reversal of cardiac dysfunction after administration of T3.72,80 Some societies therefore advocated T3 if donor heart dysfunction was encountered.7,9,76,78 However, further animal studies could not demonstrate benefit to T3.81,82 Numerous human studies were likewise unable to show correlation between T3, cardiac function, inotropic support, or improved organ yield.83–91
Thyroid hormone replacement with thyroxine has also yielded conflicting results.92,93 Revised UNOS recommendations concede that DDAVP (1-deamino-8-D-arginine-vasopressin), diuretics, and steroids rather than thyroid hormone administration increase organ yield.94 Reasons for the conflicting data may be the pattern of thyroid dysfunction in brain death. Characterized by normal thyroxine levels, elevated reverse T3, and low TSH, this matches the “sick euthyroid” state which has likewise failed to demonstrate benefit from hormone replacement.95–97 In cases of prolonged donor management or excess catecholamine administration, thyroid hormones may have some role; however, they also may be harmful and therefore cannot be advocated in all donors.88,98
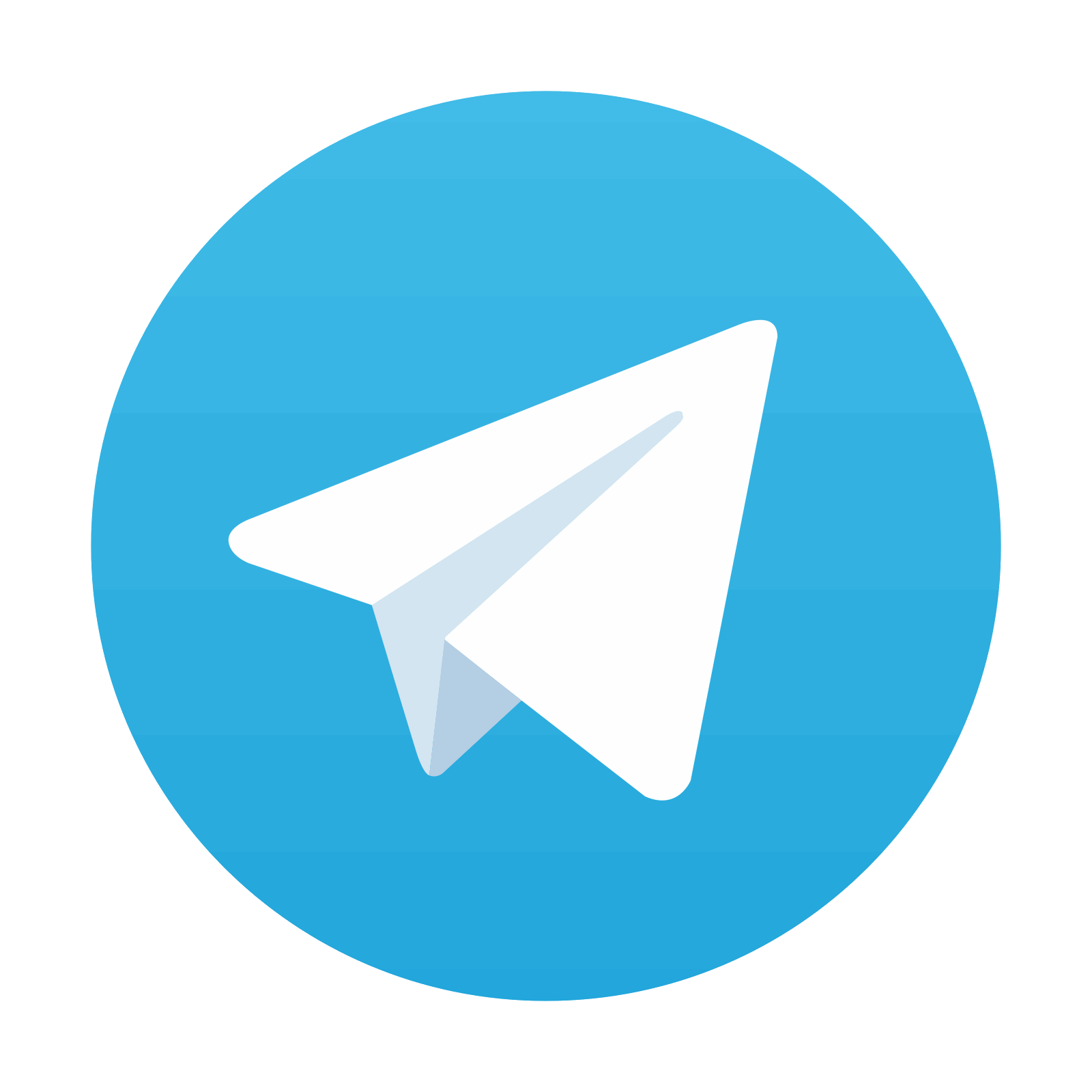
Stay updated, free articles. Join our Telegram channel

Full access? Get Clinical Tree
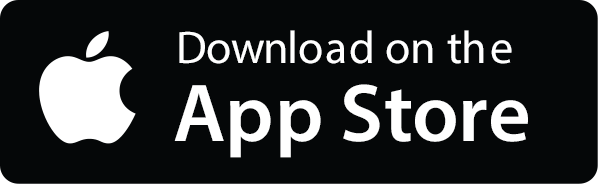
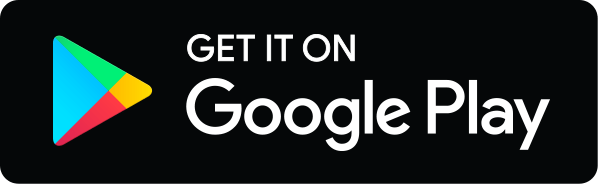