195 Management of Patients After Heart, Heart-Lung, or Lung Transplantation
Lung Transplantation
Lung transplantation offers hope for improved survival and quality of life for selected patients with end-stage lung disease. The availability of suitable donor organs and preservation injury remain the initial limiting factors to successful transplantation. Like other transplants, rejection and infection as well as organ system dysfunction associated with the perioperative course remain challenges. However, experience over 40 years has led to substantial improvements in early outcome. This experience has been reflected in changes in various aspects of the field, including a different allocation system where priority is given based on medical urgency and expected outcome,1,2 donor and recipient assessments,3 innovative surgical techniques, better understanding of early complications, and the development of newer immunosuppressive medications. Nevertheless, obliterative bronchiolitis (OB) resulting from chronic rejection and non-cytomegalovirus (CMV) infections limit the long-term quality of life and is largely responsible for the 47% 5-year mortality rate for lung transplantation.4
Diagnoses for which adults receive lung transplantation include chronic obstructive pulmonary disease (COPD)/emphysema (35.8%), idiopathic pulmonary fibrosis (20.8%), cystic fibrosis (15.9%), α1-antitrypsin deficiency (7.1%), idiopathic pulmonary arterial hypertension (3.3%), and others including sarcoidosis, congenital heart diseases, and connective tissue disease complicated by advanced lung disease. These diagnoses have remained relatively unchanged, with the exception of procedures offered to patients with idiopathic pulmonary fibrosis going from 15% of all procedures in 2000 to 27% in 2007, and procedures offered to patients with idiopathic pulmonary arterial hypertension (previously called primary pulmonary hypertension) going from 13% in 1990 to 2% in 2007. Transplantation options include single lung transplant (SLT), bilateral lung transplant (i.e., sequential bilateral single lung transplantation [BLT]), heart-lung transplant (HLT), or living-donor lobar lung transplant (LDLLT). Over the last 15 years, the number of SLT procedures has remained stable, with a steady increase in the number of BLT procedures accounting for 69% of transplant procedures in 2007.4 The trend toward bilateral transplantation has been most noticeable in patients with chronic obstructive lung disease, either from emphysema or α1-antitrypsin deficiency, which is the most frequent diagnosis leading to transplantation.5
Donor selection, procurement, and lung preservation protocols tend to be individualized on an institutional basis. The limited availability of donor lungs, however, has increased the scrutiny with which organs are judged in order to avoid rejecting them inappropriately.3 Significant lung contusion, smoking-related lung damage, pneumonia, pulmonary edema, and significant aspiration are prime concerns in evaluating the suitability of donor organs. Although already described as an independent association for primary graft dysfunction (PGD, also known as primary graft failure [PGF] or pulmonary reimplantation response [PRR]),6 donor’s older age is being challenged at some centers as a risk factor for worsened outcomes.7 Procurement and lung preservation protocols often include administration of antiinflammatory agents, pulmonary vasodilators, and antioxidants.
Hyperinflation
In SLT with emphysema, the transplanted lung can be relatively noncompliant. As a result, the native lung may be hyperinflated. This is one reason that chronic obstructive lung disease recipients are preferably offered bilateral lung transplantation.4 This problem becomes even more apparent when higher levels of PEEP are needed because of allograft dysfunction. Hyperinflation of the native, more compliant lung leads to mediastinal shift, deterioration in gas exchange, and hemodynamic instability. Although inserting an expiratory pause into the ventilator cycle can be used to assess the level of intrinsic PEEP (“autopeep”), excessive air trapping is easily diagnosed by disconnecting the patient’s ETT from the ventilator tubing for 5 to 10 seconds. In patients with significant air trapping and hyperinflation, “popping the patient off” leads to a significant improvement in blood pressure and oxygenation. Management strategies using only a single ventilator to provide ventilation include reducing PEEP, reducing tidal volume, and accepting a modest level of respiratory acidosis. Alternatively, conversion to independent lung ventilation may be appropriate, particularly in the setting of significant allograft dysfunction and high PEEP requirements. In order to switch to independent lung ventilation, an EBT is advanced into the left mainstem bronchus, and the bronchial balloon is inflated. Positioning can be verified by measuring tidal volumes delivered to and returned from each lumen of the EBT. Bronchoscopy with a small-caliber bronchoscope is appropriate to verify that (1) the left bronchial balloon is distal to the carina and (2) that the end of the left endobronchial tube does not protrude too far distally into the left lung, compromising flow to either the upper or lower division bronchi. Ventilator settings are adjusted for each machine individually. Initially, PEEP for the allograft is set at 10 cm H2O, tidal volume is set at 3 mL/kg and rate is set at 20 to 25 breaths/min. Initial settings for the emphysematous native lung typically use a larger tidal volume and a slower rate with 0 to 2.5 cm PEEP. There is no need to synchronize the ventilators. Lung hyperinflation is associated with a longer ICU stay, longer duration of mechanical ventilation, and a trend toward worsened mortality.8
Early Postoperative Respiratory Complications
Airways are affected variably by the ischemic/implantation insult. Anastomotic dehiscences are rare, although a recent report suggests incidence of this complication is increased when sirolimus was used as an immunosuppressive agent.9 Anatomically, the transplanted bronchus derives its blood supply from the lung and pulmonary blood flow, since the bronchial arteries are not typically anastomosed. The longer left mainstem bronchus, particularly adjacent to the anastomosis, is at higher risk for ischemic injury compared with the right bronchus, which generally is anastomosed adjacent to the right upper lobe take-off. Early bronchoscopy often demonstrates relatively normal epithelium. However, more severe airway injury patterns can become apparent over the next several days. The earliest findings are patchy areas of subepithelial hemorrhage that can become confluent. In more severe cases, white plaques can form, and frank areas of desiccated sloughed epithelium become evident. In the most severe cases, eschar is evident, and bronchial cartilage may be exposed. Severe airway injury poses the risk of infection and bronchomalacia. The infections are typically due to Candida and Aspergillus species.10 In many centers, lung transplant recipients are treated prophylactically with antifungal agents such as inhaled amphotericin or an azole such as voriconazole. This strategy seems to reduce the rate of airway infection.10 If suspicious plaques are evident bronchoscopically, we perform bronchial biopsy to exclude invasive disease. Inhaled amphotericin B (50 mg twice daily) is generally administered to patients with severe airway injury and those with cultures demonstrating growth of fungus. Bronchomalacia is generally a long-term complication, although in some cases, this complication can become evident within the first 6 weeks after transplantation. Dynamic airway collapse or fixed stenoses are diagnosed by bronchoscopy. In addition to endobronchial infections, malacia, and stenosis, other airway complications include dehiscence, granulation tissue formation, and fistulas.11 These are not necessarily early complications, but increased awareness of their potential occurrence is warranted.
Primary graft dysfunction (PGD) is a severe form of ischemia-reperfusion injury (IRI) and is the leading cause of respiratory failure and morbidity early after transplantation.12 A recent consensus statement by the International Society of Heart and Lung Transplantation (ISHLT) Working Group on PGD standardized the grading of PGD on the basis of gas exchange (PaO2/FIO2 ratio) and plain chest radiologic findings.13 With this grading system, a grade 3 PGD (PaO2/FIO2 < 200 plus the presence of diffuse radiographic infiltrates) resembles the definition of acute respiratory distress syndrome (ARDS), and with this in mind, it has already been validated by demonstrating a worsened mortality and prolonged hospital stay.14 The reported incidence of grade 3 PGD ranges from 10% to 25%, with 30-day mortality close to 50%.15,16 Over 95% of patients have infiltrates in the allograft by chest roentgenogram during the first 72 hours.17 Although edema and atelectasis contribute to these early changes, worsening or persistent infiltrates most likely reflect diffuse alveolar damage (DAD) secondary to PGD. Although many cases of PGD are evident on chest films obtained on the first postoperative day, in some cases, it does not become apparent radiographically or physiologically for up to 72 hours post transplant. The timing of appearance of clinical and radiologic respiratory failure is extremely helpful, then, to elaborate a judicious differential diagnosis, understanding that while PGD occurs within hours and up to 3 days after transplantation, infection and rejection are more common past the first 24 to 36 hours. In some cases, patients will be successfully extubated only to deteriorate 24 hours later. When uncertain about the etiology, aggressive diagnostic efforts should be made, employing bronchoscopy, bronchoalveolar lavage (BAL), and biopsy to exclude superimposed infection or rejection. If pulmonary edema is unilateral, a diagnosis of pulmonary venous obstruction must be entertained. Although the incidence of this problem is extremely low, a transesophageal echocardiogram (TEE) should be performed to exclude unilateral venous obstruction.
The level of respiratory dysfunction secondary to IRI depends on the extent of the injury and the residual lung reserve. The latter factor is particularly important in SLT for emphysema, because the remaining native lung may have substantial residual function. The functional capacity of the native lung often allows the transplant recipient to tolerate a significant degree of allograft dysfunction.18 Such is typically not the case for IPF patients or recipients with significant pulmonary hypertension, since perfusion to the native lung is minimal once a donor lung with low pulmonary vascular resistance is implanted.
The management of PGD is largely supportive, including judicious diuresis and a protective ventilatory approach.19 Inhaled nitric oxide may be utilized to help address early postoperative hypoxemia.20,21 Extracorporeal membrane oxygenation (ECMO) remains a salvage therapy for severe PGD.22–24
Muscular weakness or mechanical issues can embarrass postoperative respiratory function. Patients requiring delayed closure of the clamshell incision, which is required in some patients with excessive bleeding, are at higher risk for respiratory dysfunction on this basis. Preoperative muscle wasting is a major contributing factor in most cases. Clinically significant phrenic nerve injury is rare. However, when dissection of the native lungs was difficult due to dense pleural adhesions, phrenic nerve injury can be present and significantly prolong the weaning process. Postoperative neuromuscular blockade is rarely used because of synergistic adverse effects on long-term neuromuscular function that have been associated with simultaneous administration of corticosteroids (a component of most immunosuppressive regimens) and neuromuscular blocking agents.25 If the patient has difficulty clearing secretions, tracheostomy should be performed early.
Patients are treated with broad-spectrum antibiotics perioperatively. In patients without suppurative lung disease, antibiotics are stopped within 72 hours if samples from the donor trachea and from the explanted lung are without pathogens. If cultures are positive for potential pulmonary pathogens, a directed course of antibiotics is continued for 7 to 10 days. In patients with septic lung disease, an antibiotic regimen based on preoperative cultures is continued for a minimum of 2 to 3 weeks. Patients also may be treated with prophylactic regimens to prevent CMV infection with valganciclovir if either the patient or the donor is CMV immunoglobulin G(IgG) positive before surgery (dose will vary with kidney function). Aggressive prophylaxis against deep vein thrombosis (DVT) is mandatory.26 At our center, critically ill transplant patients are screened liberally for DVT with lower extremity Doppler ultrasound examinations, with a low threshold for placement of an inferior vena caval filter when DVT is diagnosed.
New Pulmonary Infiltrates
Because the transplanted lung is in close contact with the external environment, the risk for infection is higher than is the case for other forms of organ transplantation. Accordingly, aggressive diagnostic efforts are the key to management of graft dysfunction after lung transplantation. Bacterial infections with traditional nosocomial pathogens are most common in the first 30 days. Subsequently, bacterial pneumonia is still responsible for the bulk of new infiltrates, although other etiologies must be considered as well. CMV infection, causing pneumonia among other problems, occurs in a large fraction of cases. Prophylactic and surveillance strategies to deal with CMV vary from center to center. Some institutions carry out weekly assays, seeking to detect CMV antigen in the bloodstream, and only institute early preemptive therapy when the antigen is present. Other centers provide prophylaxis using ganciclovir or valganciclovir for 3 to 6 months. Dosing of these antiviral medications will vary with the kidney function. Our center monitors CMV status via frequent plasma PCR assays.27 Other viruses such as adenovirus, parainfluenza virus, influenza virus, and respiratory syncytial virus are community-acquired pathogens that can cause significant morbidity and mortality.28
Acute cellular rejection (ACR) occurs in 36% of patients at some time in the first year after transplant.4 Patients typically present with allograft infiltrates, worsening hypoxemia and dyspnea. Fever and pleural effusion may occur, and pulmonary secretions are uncommon. Clinical findings have been shown to be inadequate for diagnosing ACR; establishing the diagnosis requires transbronchial biopsy.29 Maintenance immunosuppression in most centers is based on a three-drug regimen including either cyclosporine or tacrolimus, prednisone, and either mycophenolate or azathioprine. Sirolimus is also often included in the maintenance regimen in selected instances (Table 195-1). Episodes of significant acute rejection are treated with methylprednisolone (10-15 mg/kg intravenously [IV] daily × 3 days). Response usually occurs within 24-72 hours. Failure to respond should prompt re-biopsy to exclude refractory rejection.
< div class='tao-gold-member'>
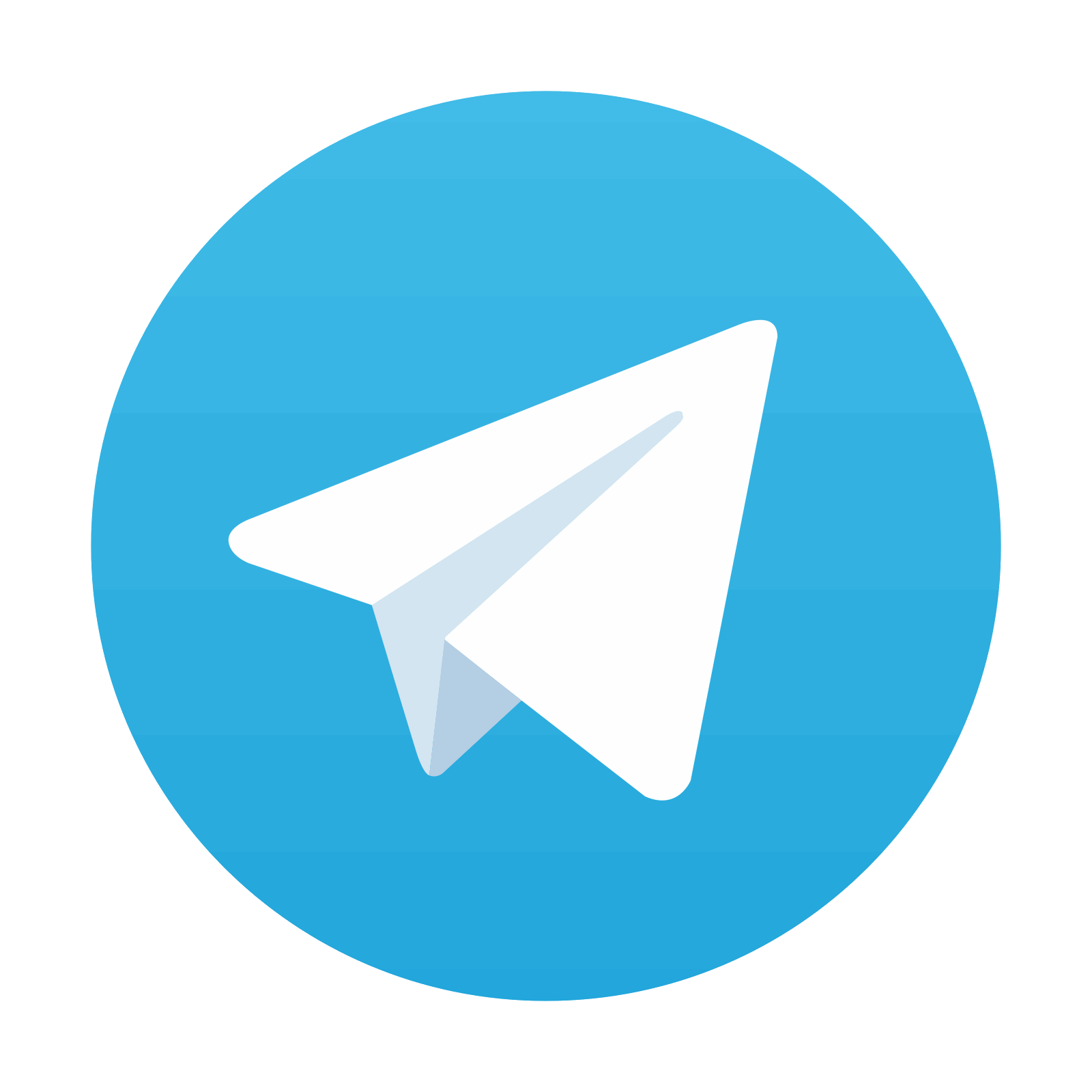
Stay updated, free articles. Join our Telegram channel

Full access? Get Clinical Tree
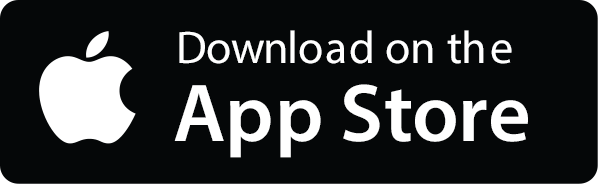
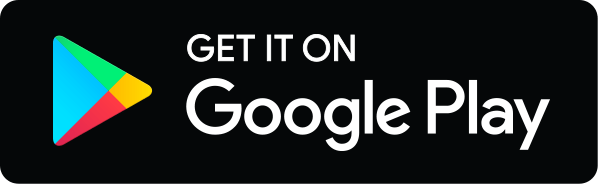