123 Macrolides
The macrolide class of antibiotics is based on the structure of erythromycin, the prototype natural macrolide isolated from Streptomyces erythreus.1 Commonly the term macrolide is expanded to include the azalide, azithromycin. The newly developed ketolides, owing to their similar structural bases, are close members of the macrolide family. There are many macrolides available throughout the world, the most commonly used being erythromycin, clarithromycin, and azithromycin. Roxithromycin is available in Europe and Asia. Telithromycin is the only currently available ketolide.
Mechanism of Action
The macrolides inhibit bacterial protein synthesis by binding to the 50S ribosomal subunit.2 The advanced macrolides have improved binding to ribosomes compared with erythromycin. Telithromycin, the ketolide, has a similar target site, but its structure allows for enhanced binding, even in the presence of ribosomal mutations.
Mechanisms of Resistance
There are three major mechanisms of bacterial resistance to macrolides: drug efflux, ribosomal mutations, and enzymatic inactivation. Active efflux, mediated by mef genes, and ribosomal methylation of the target site, mediated by erm genes, are the most clinically important resistance mechanisms.3 Organisms containing the mef gene commonly express low-level resistance that can often be overcome with larger doses of the antibiotic. In contrast, erm-containing organisms, expressing phenotypic macrolide-lincosamide-streptogramin B resistance, often express high-level resistance, rendering macrolides clinically ineffective.
Antimicrobial Spectrum of Activity
The macrolides have activity against many classes of bacteria but have only sporadic activity within each of these groups. Their primary microbiologic activity is directed against respiratory and intracellular pathogens (Table 123-1).3–21
Gram-Positive Aerobes
Among the gram-positive aerobes, erythromycin activity is limited to the streptococci with reasonable activity against Streptococcus pneumoniae. The advanced macrolides (azithromycin, clarithromycin, roxithromycin) have similar activity against S. pneumoniae. The utility of the macrolides against pneumococci is hampered by increasing resistance, commonly coupled with penicillin resistance. A 2001-2004 study from 40 countries reported 37% worldwide erythromycin resistance.3 In the United States, macrolide-resistant pneumococci are found in up to 35% of isolates.22 The predominant worldwide resistance mechanism is ermB-mediated high-level resistance (58%), but there is considerable international variability. Resistant North American isolates most commonly contain low-level mefA resistance, whereas most European and Far East countries report higher levels of ermB-containing pathogens. The prevalence of pneumococci expressing both mechanisms simultaneously is increasing.3
Resistance mechanisms for macrolides are important, because low-level resistance may possibly be overcome with conventional dosing of the macrolides.23 Pneumococcal resistance to one macrolide commonly confers resistance to all members of the macrolide class. The ketolides, however, maintain their activity against macrolide-resistant S. pneumoniae possessing both erm- and mef-mediated resistance.3
Gram-Negative Aerobes
The macrolides, with the exception of erythromycin, and the ketolide, telithromycin, have activity against Haemophilus influenzae. The activity of clarithromycin against H. influenzae is enhanced in the presence of its active metabolite.24 The macrolides and ketolide also display activity against Moraxella catarrhalis, Bordetella pertussis, Neisseria gonorrhoeae, and Neisseria meningitidis. Clarithromycin has been the most commonly used macrolide against Helicobacter pylori, although resistance rates are currently 13% in the United States, with higher rates reported worldwide.25 The macrolides are largely ineffective against the Enterobacteriaceae and other nosocomial pathogens. Though not directly active, limited auxiliary azithromycin activity has been noted against Pseudomonas spp.26
Miscellaneous
The macrolides and the ketolide attain high intracellular concentrations and are active against Legionella spp., Chlamydia spp., and Mycoplasma pneumoniae. In vitro activity is also present against Rickettsia, Bartonella, and Brucella spp.,27–29 as well as Borrelia burgdorferi, the agent of Lyme disease.30 In addition, azithromycin, clarithromycin, and telithromycin have activity against some strains of atypical nontuberculosis mycobacteria, including Mycobacterium avium complex.31–33
Pharmacokinetics
Erythromycin base is acid labile but still adequately absorbed from the gastrointestinal (GI) tract. Food can decrease absorption (Table 123-2).1,34–42 More stable oral formulations have complexed erythromycin with salts or esters to form erythromycin estolate, stearate, and ethylsuccinate. Erythromycin lactobionate has also been formulated to allow for intravenous (IV) delivery. Peak concentrations are 0.73 µg/mL after 250 mg base orally and 10 µg/mL after 500 mg IV.1,34 The half-life is 1 to 1.5 hours.35 Like all macrolides, erythromycin is widely distributed throughout the body, with higher tissue and intracellular concentrations compared with plasma. Erythromycin is not found in the cerebrospinal fluid in normal volunteers, but low levels have been reported in patients with meningitis.35 Erythromycin is metabolized by cytochrome P450 (CYP) enzymes in the liver and excreted as inactive metabolites, primarily in the feces.
Clarithromycin is well absorbed from the GI tract (bioavailability 52%-55%), with or without food. An IV lactobionate form is available in some countries. A peak concentration of 1.65 to 2.12 mg/mL is obtained after a 500-mg oral dose with a half-life of 3 to 5 hours.37 Similar pharmacokinetics are observed after dosing with the oral suspension, even in critically ill patients.43 An extended-release formulation is available that delays the time to peak concentrations, provides similar total drug exposure, and allows for once-daily dosing.36 Clarithromycin is well distributed throughout the body, with respiratory tract tissue and fluid concentrations 3 to 30 times that of the plasma and alveolar macrophage concentrations 102 to 103 higher than plasma.37 Cerebrospinal fluid concentrations are unknown. Hepatic metabolism is the major metabolic pathway and leads to the formation of 14-hydroxy-clarithromycin, an active metabolite with greater activity than the parent compound.24,37 Clarithromycin is extensively metabolized, with 18.4% and 4.4% of unchanged drug excreted in the urine and feces, respectively, after a 250-mg dose.37 Dosing changes are required in patients with moderate to severe renal dysfunction.
Azithromycin is 37% bioavailable when administered orally but is also available in an IV formulation.39,44 Food has little effect on bioavailability. Peak concentrations in the plasma after a 500-mg dose range from 0.4 µg/mL for the oral formulation to 3.6 µg/mL for the IV formulation.38,39 An extended-release formulation of a single 2-g azithromycin dose shows a similar overall pharmacokinetic profile to standard oral dosing.40 Azithromycin is unique in its extended half-life of 14 to 40 hours, thus providing low sustained plasma concentrations that persist after cessation of dosing.39 Whereas plasma concentrations are very low, azithromycin attains very high concentrations in tissues (100 times plasma) and phagocytes (3000-7000 times plasma).38 Little to no azithromycin can be recovered from the cerebrospinal fluid, but brain tissue concentrations well exceed those in the serum.45 Azithromycin is minimally metabolized and largely excreted via the biliary tract into the feces.
Roxithromycin is well absorbed orally, with peak plasma concentrations of 6.6 to 7.9 µg/mL after a 150-mg oral dose, and a half-life of 8.4 to 15.5 hours.41 Fasting prior to dosing improves absorption. Tissue concentrations exceed those of the plasma. Roxithromycin is metabolized by multiple mechanisms, with the majority of the dose excreted in feces.41,46
Telithromycin is 57% bioavailable, with peak plasma concentrations of 1.9 and 2.3 µg/mL after single or multiple 800-mg doses, respectively.42 The half-life of telithromycin is 7 to 10 hours. Like the macrolides, telithromycin achieves high concentrations in respiratory tissues, alveolar macrophages, and peripheral polymorphonuclear cells. Telithromycin is metabolized by CYP3A4 and non-CYP–related mechanisms. Fecal elimination of metabolites accounts for the majority of the excretion of telithromycin.
Pharmacodynamics
The macrolides and ketolides appear to have time-dependent antibacterial activity that is slowly bactericidal or bacteriostatic.47,48 The relationship between drug concentration and bacterial effect that best explain drug activity is the free (unbound) drug area under the inhibitory curve (fAUC: MIC).47,48 Differences in the pharmacokinetics of the individual agents and limited analyses do not allow absolute determination of the best dosing strategy. It should be noted that pharmacodynamic principles for antibacterials have generally been related to plasma concentrations.49 As noted earlier, the plasma concentrations of the macrolides are usually lower than those of the tissues, where the majority of bacteria reside. Models looking at both epithelial lining fluid (a proxy for lung concentrations) and serum concentrations suggest that increasing bacterial resistance favors telithromycin in its ability to maintain favorable pharmacodynamics.50
Immune Modulation
Increasing evidence suggests that antibacterial macrolides have antiinflammatory effects.51–53 In vitro studies have suggested macrolide effects on a number of cellular mechanisms within human cells.54 In addition to in vitro and animal models, clinical data suggest that macrolides may have activity in the treatment of inflammatory diseases including cystic fibrosis, diffuse panbronchiolitis, chronic sinusitis, and inflammatory skin diseases.51,53 Azithromycin has been cautiously recommended for use in cystic fibrosis for its antiinflammatory effects in patients colonized with Pseudomonas spp.55
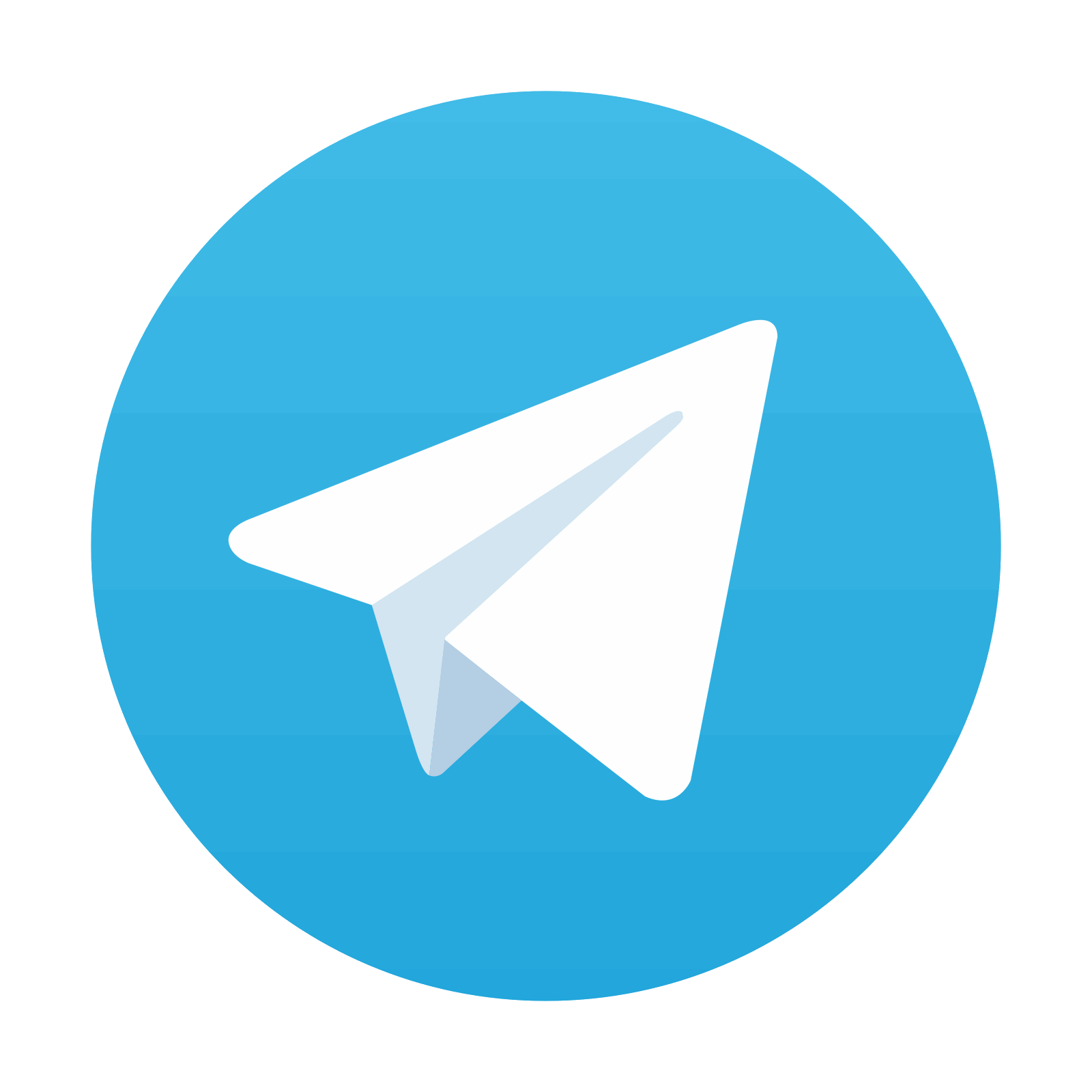