CHAPTER 45 Invasive Hemodynamic Monitoring in the Cardiac Intensive Care Unit
HEMODYNAMICS IS derived hydrodynamics, the physics of the motion and action of water. The dimensions of hydrodynamics include: flow, pressure, static resistance, dynamic impedance, reflectance and compliance, branching effects, viscosity, fluid friction, turbulence, and other physical characteristics.1 The goals of hemodynamic assessment and manipulation in the critically ill patient are to ensure adequate organ blood flow and oxygen supply.2 Noninvasive parameters to measure organ perfusion include systolic and diastolic blood pressure, body temperature, heart rate, and respiratory frequency.3 Though established clinical signs of organ perfusion should be used for monitoring, these sometimes have limited value as accurate indicators of cardiovascular function.4 Important pathophysiology may remain obscured if judged solely on clinical signs.2 The evolution of modern technology has allowed for quantitative biologic measurements. The principles of hemodynamic monitoring have allowed basic physiologic measurement to be applied in a meaningful manner in the management of cardiovascular aspects of critical illness.5 The development of bedside intravascular catheterization procedures has permitted extension of the application of the physiologic principles established early in the century to the population of critically ill patients.5
Systemic Arterial Blood Pressure
The continuous measurement of arterial pressure is essential in hemodynamic monitoring. Arterial pressure is the input pressure for organ perfusion.6 It is usually monitored noninvasively with a sphygmomanometer. In the Cardiac Intensive Care Unit (CICU), however, a more invasive technique with insertion of an indwelling arterial catheter into either the arm (brachial or radial sites) or groin (femoral arterial site) is often used to provide more precise monitoring. The advantages of arterial catheterization over noninvasive means are continuous monitoring of arterial pressure and its waveform, and serving as a site for repetitive blood sampling.6
The arterial pressure is a function of both vasomotor tone and cardiac output. Local metabolic demands determine local vasomotor tone that in turn determines blood flow distribution.6 The perfusion pressure and local vascular resistance determine organ perfusion of all capillary beds. Flow is proportional to local metabolic demand if there is no hemodynamic instability to cause increased sympathetic tone.6 Cardiac output primarily determines arterial pressure in the setting of varying degrees of local blood flow and because it is proportional to local metabolic demand, there is no normal value in an unstable, metabolically active patient.6 The literature currently suggests maintaining previously nonhypertensive patients at a mean arterial blood pressure (MAP) of 65 mm Hg.6,7 Based upon a clinical trial that examined the effects of resuscitative efforts with fluid and vasopressors in circulatory shock patients to varying MAP targets ranging from 60 to 90 mm Hg. No increased organ blood flow could be determined above a MAP of 65 mm Hg.8 Thus, there is no proven benefit in forcing either cardiac output or arterial tone to higher levels above this threshold.
Table 45-1 displays indications for arterial catheterization. The transducers used for blood pressure measurement are connected to the circulation via fluid-filled tubing. The accuracy of the system is optimized when the catheters and tubing are stiff, the total length of tubing is not excessive, the number of stopcocks is limited, and residual air bubbles are eliminated.2 The “zeroing” of the transducer at the appropriate level in relation to the patient (i.e., midthorax) must be correct to prevent errors caused by hydrostatic pressure of the fluid column.2,9 In most cases, the choice of location for insertion of the catheter is the radial artery because the femoral artery approach is more often associated with displacement during patient movement and hemorrhage that is more difficult to control.1 Although arterial catheterization is an invasive procedure with inherent risks, most complications are not severe (temporary vascular occlusion 20% and hematoma 14%) with permanent ischemic damage, sepsis, and pseudoaneurysm formation occurring in less than 1% of cases.10
Table 45–1 Arterial Catheterization∗
Indications for arterial catheterization |
As a guide to synchronization of intra-aortic balloon counter pulsation |
Probable indications for arterial catheterization |
Guide to management of potent vasodilator drug infusions to prevent systemic hypotension |
Guide to management of potent vasopressor drug infusions to maintain a target MAP |
As a port for the rapid and repetitive sampling of arterial blood in patients in whom multiple arterial blood samples are indicated |
As a monitor of cardiovascular deterioration in patients at risk for cardiovascular instability |
Useful applications of arterial pressure monitoring in the diagnosis of cardiovascular insufficiency |
Differentiating cardiac tamponade (pulsus paradoxus) from respiration-induced swings in systolic arterial pressure; tamponade reduces the pulse pressure but keeps diastolic pressure constant. Respiration reduces systolic and diastolic pressure equally, such that pulse pressure is constant. |
Differentiating hypovolemia from cardiac dysfunction as the cause of hemodynamic instability. Systolic arterial pressure decreases more following a positive pressure breath as compared with an apneic baseline during hypovolemia. Systolic arterial pressure increases more during positive pressure inspiration when LV contractility is reduced. |
∗ Adapted from Polanco PM, Pinsky MR: Practical issues of hemodynamic monitoring at the bedside. Surg Clin North Am 2006;86(6):1431-1456.
Right Heart and Pulmonary Artery Catheterization: A Historical Perspective
The data that is the basis of our current understanding of cardiac function was obtained nearly 100 years ago. Claude Bernard performed the initial cardiac catheterization in 1844 entering both the right and left ventricles of a horse.9 The physiologic and anatomic data obtained over the ensuing years lead to the development of important concepts, such as pressure manometry and cardiac output measurement. In 1929, Werner Forssmann successfully performed a right heart catheterization on himself. In 1947 Lewis Dexter passed a catheter to the distal pulmonary artery and measured pulmonary capillary wedge pressure and oxygen saturation for the first time. Subsequent work from his laboratory noted pulmonary capillary wedge pressure to be a reliable estimate of left atrial pressure.11 In 1956 Dickinson Richards and Andre Cournard along with Forssmann were awarded the Nobel Prize in Medicine for their work describing cardiovascular physiology using the pulmonary artery catheter (PAC).9
Right heart catheterization permitted measurements of cardiac output and right heart and pulmonary pressures that make it possible to calculate other derived hemodynamic parameters, such as cardiac work indices and systemic and pulmonary vascular resistance.2 Initially, the procedure was exclusively performed in the cardiac catheterization laboratory primarily to diagnose congenital and valvular heart lesions.12 However, the increasing numbers of admissions to CICUs for hemodynamic instability as a result of ischemic heart disease prompted an expanded role for invasive hemodynamic monitoring. The 1960s ushered in the establishment of Coronary Care Units (CCU) to manage cardiac arrhythmias in the setting of acute myocardial infarction.1,13 It was noted that profound hemodynamic changes might be the primary factor in arrhythmic deaths.1 However, practical limitations in transporting critically ill patients to a cardiac catheterization suite and the increased risk of induction of cardiac arrhythmias limited the application of invasive hemodynamic monitoring.1
In 1970, motivated by a lack of understanding of the disease process in those patients admitted with ischemia, Swan developed a balloon-tipped, flow-guided catheter that for the first time established a reliable way to perform bedside catheterization in specialized cardiac care units.14 The initial catheter was a double lumen extruded polyvinylchloride catheter with an outside diameter of 1.6 mm and a length of 110 cm.5 There was a minor lumen of 0.4 mm in diameter and a major lumen of 1 mm in diameter. The minor lumen was used to inflate the balloon to a volume of about 0.8 mL. Their initial study involved 100 patients in whom successful bedside hemodynamic monitoring was preformed.14 They concluded it was possible to safely catheterize the pulmonary artery (PA) at the bedside without fluoroscopy in a high proportion of patients. The information gained proved to be important in understanding the altered pathophysiology of a given illness and thereby altered management in these patients.5
It is following this landmark report that widespread use of PACs began. The relative ease in hemodynamic monitoring transformed the care of cardiac pump failure in the CCU. It allowed recognition of hemodynamic subsets in myocardial infarction, such as right ventricular infarction, and provided additional prognostic insight and allowed for the selection and monitoring of pharmacologic therapy.15
As stated earlier, the PAC allows for determination of various fundamental hemodynamic parameters, including measurement of cardiac output by the thermodilution (TD) right atrial (RA), right ventricular (RV), PA, and pulmonary capillary pressures; and sampling of blood from the PA, the RV, and RA. Pulmonary vascular and systemic resistance and right and left ventricular stroke work could then be derived. Further developments include continuous measurement of PA blood oxygen saturation16 and near continuous or instantaneous cardiac output.17
The Catheter
The catheter most commonly used is a 7.5 French thermodilution catheter. It is a 3-lumen radiopaque catheter, 110 cm long and made of polyvinylchloride. The outside is marked with black rings every 10 cm from the tip that allow determination without fluoroscopy of the appropriate catheter length at which to inflate the distal balloon. The distal lumen terminates at the tip of the catheter, whereas the RA lumen terminates 30 cm proximal to the tip. There is a venous infusion lumen 1 cm proximal to the RA lumen. A thermistor bead located 3 to 5 cm from the tip is connected to an external thermistor connector by a wire. The external thermistor is in turn linked to a computer that allows for determination of cardiac output by the thermodilution method.14,15,18 A latex balloon with a maximum inflating capacity of 1.0 to 1.5 mL is positioned at the tip. Upon inflation, the balloon engulfs the catheter tip reducing the transmitted force and hence limiting irritation and injury of endocardium and reducing the frequency of arrhythmia.14 The inflated balloon facilitates flow-directed advancement of the catheter through the right heart into the pulmonary artery. Once inflated in a distal branch of the pulmonary artery, the balloon occludes the vessel and allows for measurement of pulmonary capillary pressure. Most catheters are heparin-coated to reduce thrombogenicity. In addition, electrodes implanted in some catheters allow for pacing and electrocardiographic recording. The inclusion of fiberoptics allows measurement of oxygen saturation in the PA. The catheter can serve multiple functions including: measurement of CO by TD, PA temperature, intracardiac pressures (RA, RV, PA, PCW), and PA occluded pressure. Blood sampling can be done through the active lumens of the catheter.
Equipment and Signal Calibration
Properly functioning electronic monitoring equipment is essential. The fluid-filled PAC is connected via a semirigid pressure tubing to pressure transducers. These transducers consist of a fluid-filled dome, a diaphragm, and a strain gauge Wheatstone bridge arrangement.18 An electric current directly proportional to the fluid motion is amplified and transmitted to the oscilloscope equipment for display. Other factors that influence accurate reproduction of a biologic signal include frequency response, natural frequency, damping, and catheter whip artifact.
The system must have a frequency response of flat to 15 to 20 Hz to be adequate for human studies. Pressure waveforms are not reliable in patients with excessively rapid heart rates of greater than 180 beats/min. The length of the pressure tubing determines the natural frequency of fluid-filled systems. Excessively long tubing will drop the natural frequency to below physiologic range, causing an overamplification of signal resulting in falsely elevating pressure readings. The recommended length of pressure tubing is 3 to 4 feet.18 Damping is the opposite effect with a loss of physiologic signal, which most often results from air trapped in the circuit. Because air, unlike fluid, is compressible, less motion is transmitted to the diaphragm per unit of pressure. Damping of the PA pressure signal may make it difficult to discern from the wedge pressure. Catheter whip artifact results from motion imparted to the catheter with each cardiac contraction. High-frequency filters can eliminate this artifact.18
Accurately measuring pressure signals requires proper calibration of the monitoring system. With a patient supine, the pressure transducer is aligned with the fourth intercostal space midway between the front and the back of the chest.18 This site serves as the standard zero reference point. The calibration of the monitor involves the introduction of a known pressure signal. This can be done either internally or externally. Zero reference and calibration should be checked each day of hemodynamic monitoring.18 Table 45-2 lists the required equipment for PAC insertion. Prepackaged kits with the above materials are available.
Table 45–2 Equipment Required for Swan-Ganz Catheter Insertion
Appropriate Swan-Ganz catheter |
Dilator-sheath-side arm assembly |
Three-way stopcocks |
Pressure tubing |
Transducers |
18-gauge, thin-walled Cook needle |
Sterile gowns, drapes, gloves |
1% lidocaine |
Heparinized saline |
J-tipped guidewire |
Towel clips, syringes, suture material |
Electrocardiography and pressure-monitoring equipment |
Intravenous line |
Atropine |
Defibrillator unit |
Catheter Insertion
Catheter equipment should be inspected and calibrated. All lumens should be flushed with normal saline and be free of air. Insertion site selection can vary between internal jugular, subclavian, and femoral veins (Table 45-3). Although the site of insertion is at the discretion of the operator, the right internal jugular vein is preferable because of the straight course to the superior vena cava. Meticulous preparation of the chosen site with aseptic technique is crucial. The operator and any assistants should be in sterile gown, gloves, facemasks and caps. Any other personnel in the room should also have masks and caps.
Table 45–3 Comparison of Venous Access Routes
Vein | Advantages | Disadvantages |
---|---|---|
Internal jugular | ||
Subclavian | Air embolism, more frequent pneumothorax and hemothorax; subclavian artery puncture; injury to nerve bundle may occur | |
Femoral | Sepsis, in situ thrombosis, and pulmonary embolism may occur usually requires fluoroscopy |
The patient should be properly prepped, draped, and in the Trendelenburg position.
The site is then accessed percutaneously through a modified Seldinger technique. After local anesthesia, an 18-gauge, 7.6-cm Cook needle with attached syringe is inserted bevel up at approximately 45 degrees between the heads of the sternocleidomastoid muscles toward the ipsilateral nipple, while palpating the ipsilateral carotid artery. After free flowing venous blood is obtained, the syringe is disconnected from the needle and a 40 cm long J-tipped guide wire is inserted into the needle and passed gently into the vein. The guide wire should pass without any resistance. The guide wire should never be advanced if any resistance is encountered. The needle is then removed and the skin puncture is enlarged with a scalpel blade. The dilator sheath system is then advanced over the guide wire into the vein with a gentle rotating movement. Once the sheath is properly positioned, the wire and dilator should be removed and the sheath sutured in place.
The catheter is then inserted and advanced approximately 10 cm before the balloon is inflated with 1 to 1.5 mL of air. In most patients the catheter will reach the RA within 10 to 15 cm from the internal jugular or subclavian vein. Once in the RA, the catheter should be quickly advanced under continuous pressure and electrographic monitoring across the tricuspid valve, through the RV, PA, and into the pulmonary wedge position. The catheter should reach the PA approximately 50 to 55 cm from the internal jugular vein. One should suspect catheter coiling if significantly greater lengths are required. Figure 45-1 illustrates typical pressure waveforms.
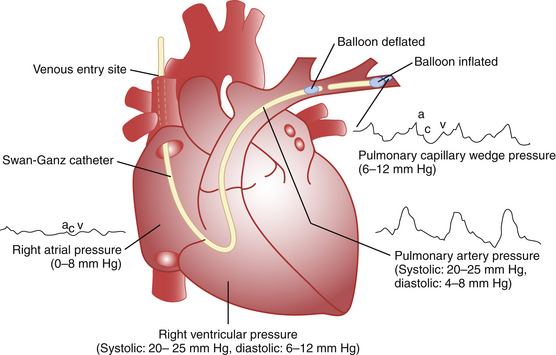
Figure 45-1 Normal hemodynamic pressures.
(Adapted from Swan HC: The pulmonary artery catheter. Dis Mon 1991;37:518.)
The deflated catheter should recoil slightly into the proximal PA. With inflation, the catheter should then float to a wedge position. The goal is passive occlusion of a distal vessel impeding blood flow to that area. The balloon should be slowly inflated while pulmonary artery pressure is continuously monitored. Wedge tracings obtained at substantially less than the full inflation volume indicates distal migration of the catheter. With the balloon deflated, it should be repositioned with slow withdrawal of 1 to 2 cm at a time. Poor wedging can be due to patient movement, mechanical ventilation, positive end-expiratory pressure (PEEP), and eccentric balloon inflation.18 Catheter position should be checked routinely by overpenetrated chest x-rays.18 To minimize the risk of endothelial damage to the PA, PA rupture, or pulmonary infarction, wedge time should be kept to a maximum of about 8 to 15 seconds.18 End-expiration diastolic pulmonary pressures should approximate mean wedge pressure in the absence of increased pulmonary arteriolar resistance, such as with pulmonary hypertension or pulmonary embolus.18
The pressure recorded through a wedged end-hole catheter is that of the next vascular system, which in most circumstances reflects the left atrium (LA) or left ventricular (LV) end-diastolic pressure. This assumes that the vascular system distal to the catheter tip provides a direct connection to the LA without any anatomic or functional interruption. The ideal placement of the catheter is the lower lung zone. In zone 3, the most dependent portion, both pulmonary artery and venous pressures exceed alveolar pressure thereby maintaining an open vascular system from the catheter tip to the LA.18 In the upper lung, or zone 1, alveolar pressure exceeds pulmonary artery and venous pressures, keeping the capillaries closed and therefore disrupting the system preventing accurate measurement of LA pressures. The arterial pressures in the central lung, or zone 2, should exceed alveolar pressure, but low pulmonary venous pressure may prevent retrograde transmission of pressures to assessment of the LA. Fortunately, most of the lung in the supine position is in zone 3 and flow-guided catheters will usually enter this zone. A lateral chest x-ray can confirm position of the catheter tip below the LA.
Pulmonary Artery Occluded and Wedge Pressure
Pulmonary capillary pressure is a phase-delayed, amplitude-damped version of LA pressure. During diastole with a nonstenotic mitral valve, the pulmonary venous system, LA, and LV is a continuous circuit and the pulmonary capillary pressure is then reflective of the LV diastolic pressure.15
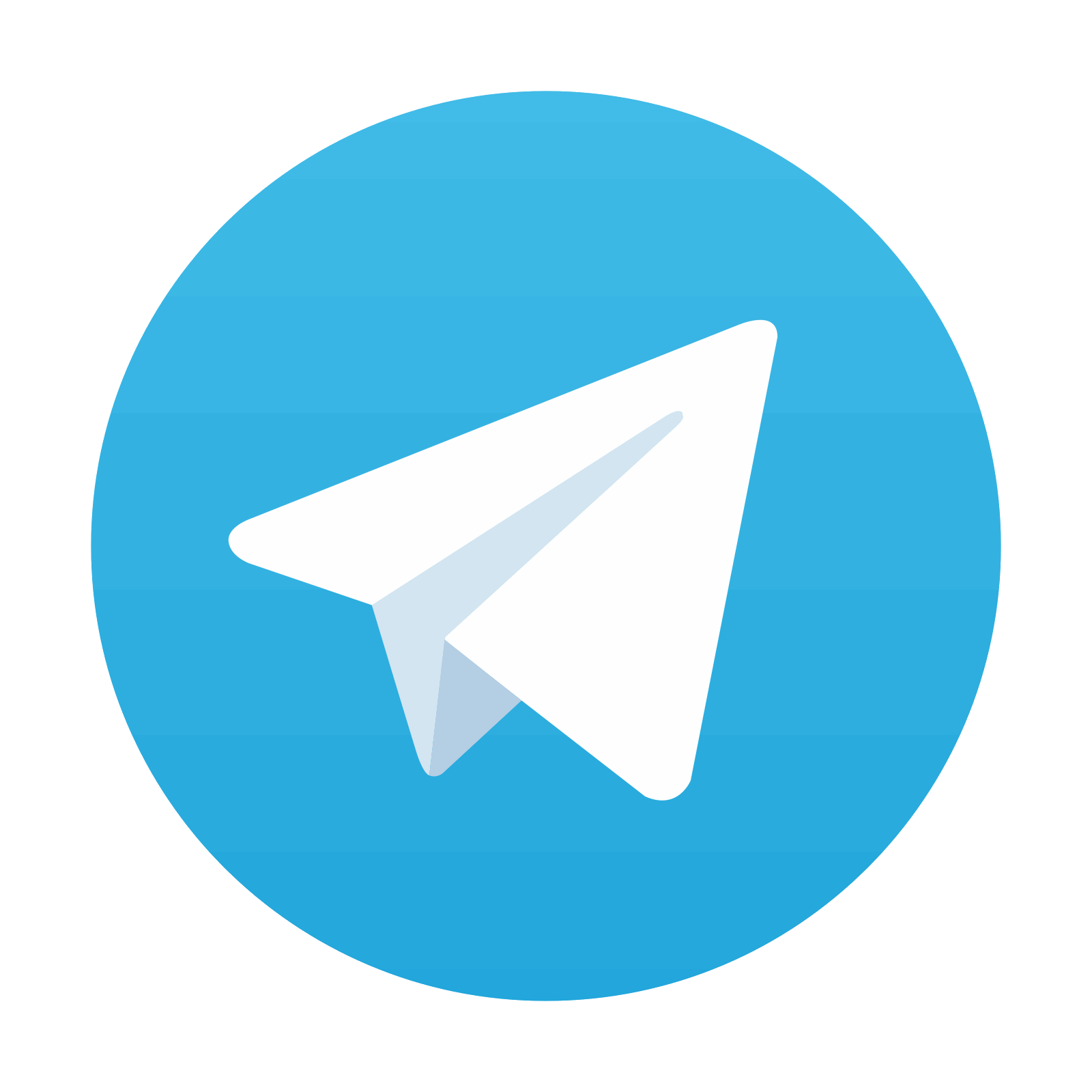
Stay updated, free articles. Join our Telegram channel

Full access? Get Clinical Tree
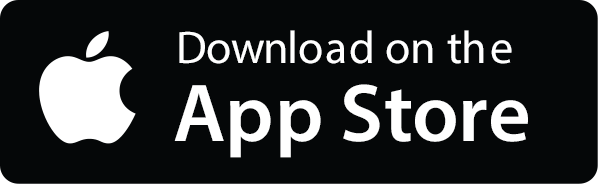
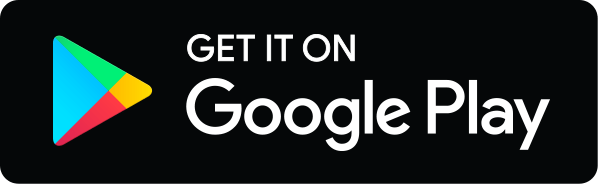