Minimal sedation
Moderate sedation
Deep sedation
General anaesthesia
Responsiveness
Normal response to verbal stimulation
Purposeful response to verbal or tactile stimulation
Purposeful response following repeated or painful stimulation
Unrousable even with painful stimulus
Airway
Unaffected
No intervention required
Intervention may be required
Intervention often required
Spontaneous ventilation
Unaffected
Adequate
May be inadequate
Usually inadequate
Cardiovascular function
Unaffected
Usually maintained
Usually maintained
May be impaired
The Academy of Medical Royal Colleges (UK) is more simplistic: “If verbal responsiveness is lost the patient requires a level of care identical to that needed for general anaesthesia”. Hence, if propofol is ever to be self-administered by patients without an anaesthetist present, the methods of delivery must have some way of limiting drug administration to avoid loss of verbal contact.
Indications and Demand for Sedation
Patients request sedation for many types of invasive procedures, e.g. gastrointestinal endoscopy, bronchoscopy, dental surgery, interventional radiology, emergency department procedures and oocyte retrieval for assisted fertility. Patients request sedation for a variety of reasons: anxiolysis , amnesia and reduced consciousness. Regardless of the reason, provision of sedation generally improves patient satisfaction [4, 5]. For example, the scale of sedation occurring in the UK is vast (Table 21.2).
Table 21.2
Hospital episode statistics Department of Health 2000–2001
Procedure | Three-digit OPCS4 code | Completed episodes | % As day case |
---|---|---|---|
Upper GI endoscopy | G01-25 | 561,572 | 72 |
ERCP | J38-45 | 33,504 | 21 |
Colonoscopy | H04-30 | 331,646 | 75 |
Bronchoscopy | E01-63 | 205,984 | 61 |
Given the large numbers of patients receiving sedation, it is not practical that anaesthetists provide all sedation. Indeed, it seems that anaesthetists already do not provide the majority of sedation. For instance, the National Confidential Enquiry into Patient Outcome and Deaths (NCEPOD) 2004 studied deaths associated with invasive gastrointestinal endoscopy surgical procedures in the UK [6]. An estimated 128,000 procedures were performed in 2003, with a 3 % 30-day mortality. In approximately 85 % of cases, the operator or nurse administered sedation.
Dentistry
Historically general anaesthesia was used to treat the severely anxious patient in general dental practice; however, there were serious concerns about the safety of this and was highlighted in the Poswillo report [7]. The subsequent reduction in general anaesthetic numbers was not sustained [8]. Eventually in 1998 the General Dental Council banned general anaesthesia by all non-anaesthetists [9]. This led to a large increase in the sedation required in primary care [10].
A survey of primary care dentists revealed around 75 % felt a need for sedation in their practice, with around half providing sedation for their patients [11]. Only about 10 % of these had any training in sedation. The sedation techniques being used were mainly i.v. sedation with midazolam , oral sedation with temazepam and nitrous oxide. One dentist was using propofol routinely. Most patients were monitored using pulse oximetry, with few using blood pressure and electrocardiography (ECG ) [11]. There has been development of general dental practitioners with special interests in anxiety and sedation, this is likely to develop further, and training is likely to become standard for undergraduates and encouraged for those already in practice [12]. There is also a parallel secondary care service [13], which provides both primary and secondary restorative dental care for patients under sedation [14]. There are also patients who are medically compromised who may require sedation but are not considered suitable for treatment in primary care. The majority of those using sedation in secondary care used i.v. midazolam, with a minority using propofol and diazepam. An overwhelming majority of 80 % of these had a separate operator and sedationist. Secondary care sedation in dentistry is more developed than in many other specialties with systems of training and accreditation [15]. Realising the likely increase in demand for sedation the British Dental Association was proactive in instituting guidelines for sedation practice and coordinated training for this via the Dental Sedation Teachers Group.
Gastrointestinal Endoscopy
Sedation is widely used in endoscopy. Older reports suggested that benzodiazepines were predominantly used, often in conjunction with opioids [16, 17].
Practice appears to have changed recently, and propofol has been used increasingly. Although primarily an anaesthetic drug, it has been given with a surprisingly good safety profile by non-anaesthetists, such as general practitioners [18]. It is popular with non-anaesthetists because of its many desirable qualities [19, 20]. There are numerous studies examining its efficacy in colonoscopy [21], and emerging data that in this environment morbidity is relatively rare [22]. Its use by non-anaesthetists is however controversial to say the least. For example, the European Society of Anaesthesiologists after trying to control its use has come out quite strongly against its use by non-anaesthetists [23]. The Academy of Medical Royal Colleges in the UK has been more pragmatic and tried to improve standards of care and training.
Emergency Medicine
Historically sedation in the emergency department was mainly based using a benzodiazepine, with limited monitoring, training and knowledge [24]. More recently it has been proposed strongly that propofol use in emergency medicine is safe, a better sedative than benzodiazepines, and that serious complications are acceptably rare [25]. The use for sedation in the UK by emergency departments has become popular; it seems mainly administered by physicians (most of whom) have at least some anaesthetic experience. Early reports were, however, worryingly naive in their proclamations of safety [26] and came in for strong criticism by our group [27].
In fact some patients were almost certainly receiving general anaesthesia, and with a far worse safety record than other published experience. In defence, the criticism has been taken on board and larger case series are being reported. In addition, there has been a serious attempt at clinical governance with regard to index incidents using the World Society for Intravenous Anaesthesia Sedation Index Events framework [28, 29]. The Academy of Medical Royal Colleges Sedation publications are almost certainly helping, but it has been pointed out again that even with a more conservative dose regimen, many patients are still receiving anaesthesia [30].
The Main Options for Sedative Agents
Benzodiazepines
Theoretically any benzodiazepine can be used to provide sedation. However midazolam is the most popularly used agent owing to its relatively rapid onset and offset compared to the alternatives diazepam and lorazepam [31]. It seems most logical to give midazolam intravenously. However, it is also possible to give it sublingually or intranasally. Though popular in children, the volume required in adults limits its usefulness.
Midazolam gives good amnesia and anxiolysis [32, 33] though it is also associated with delayed return of cognitive function and discharge. The most common regimens involve relatively frequent titration of small doses until the patient reaches the desired sedation level either by a physician [34] or even using patient-controlled sedation (PCS ) [32] or target-controlled infusion (TCI) [35]. It is recognised that the elderly are more sensitive [36], and most titrate such patients much more cautiously. It seems that recovery from midazolam is the fastest of the benzodiazepines, but longer than propofol [32, 37]. The main unrecognised problem with midazolam is that its pharmacodynamics have a relatively slow onset and longer than appreciated offset [38]. The t1/2ke0 (the half-life for midazolam in the plasma equilibrating with the brain) is relatively slow, one estimate being around 9 min [39]. This means that when the patient has reached the desired sedation level, and drug administration stops, the clinical effect of the drug (effect-site concentration (Ce)) is still rising for 10–20 min (Fig. 21.1) [38].
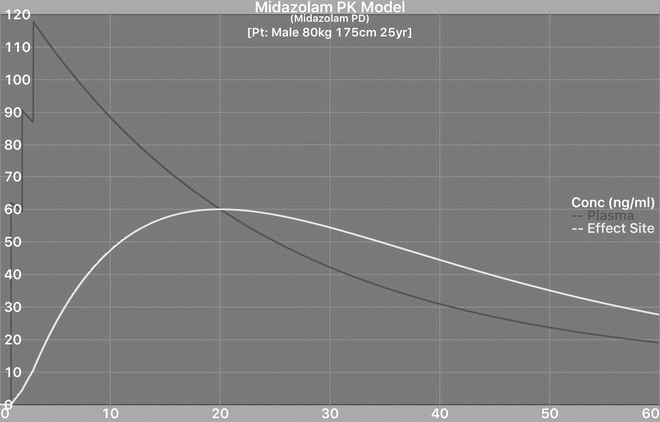
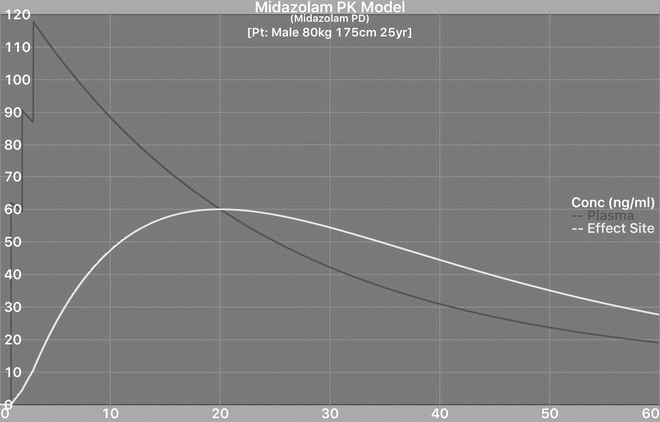
Fig. 21.1
Typical titration of midazolam i.v. with 2 mg followed by 1 mg, 1 mg at minute intervals. Note the effect-site concentration significantly lags behind plasma concentration. Hence if clinical sedation is judged to be adequate after the last dose, the effect-site concentration continues to rise for the next 17 min. This is calculated with a pharmacokinetic simulator using the Zomorodi model and a recent estimate of ke0
This perhaps explains the reports of prolonged hypoxia after the use of midazolam in conjunction with opioids in the elderly for upper GI endoscopy (Fig. 21.2) [40]. Common problems are respiratory depression, reduction in blood pressure and paradoxical excitement [41].
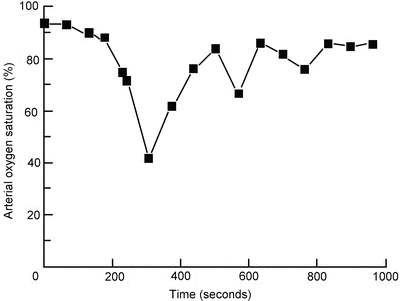
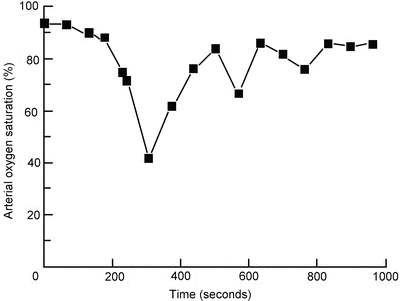
Fig. 21.2
Example of prolonged arterial oxygen desaturation after sedation with midazolam and pethidine (meperidine) [40]
A major advantage of midazolam is the availability of the reversal agent flumazenil, which can reverse the sedative and respiratory depressant effects. However, sedationists should be wary that the half-life of flumazenil is relatively short, and its effects may wear off before the midazolam has been eliminated [42].
Many of the adverse events in previous studies of morbidity and mortality were attributed to inappropriate use of benzodiazepines and poor monitoring and recovery [16, 17]. The NCEPOD report in 2004 still identified about 14 % of deaths for endoscopy where sedation was a factor, predominantly excessive doses and combinations of drugs, particularly in the elderly. Although recently its safety profile has improved [43], there are still warnings of adverse events with midazolam from the National Patient Safety Agency [44].
Propofol
Propofol has been used in sub-anaesthetic doses for over 25 years [45]. Propofol is an excellent sedative due to its pharmacokinetics and dynamics [45]. Its onset is rapid [46] and recovery faster than midazolam [32, 34, 37, 47]. It has long been known to have anxiolytic and sedative properties with minimal amnesia at plasma concentrations less than 1.0 μgċml−1 [48]. It seems to offer excellent anxiolysis and has even been used as a premedication using target-controlled infusions (TCI) before surgery, significantly reducing state anxiety visual analogue scores [49]. A subset of these patients rated propofol superior to previous benzodiazepine premedication. Interestingly subsequent analysis of these data revealed that those with the highest visual analogue anxiety score before sedation had the largest drop in anxiety after propofol (Fig. 21.3).
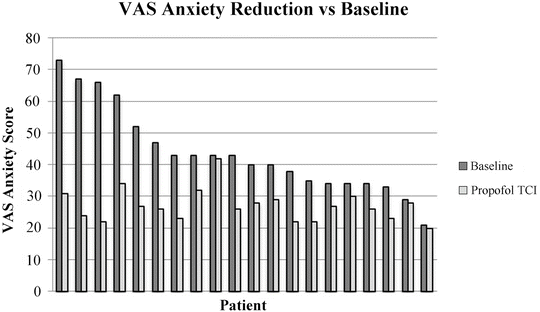
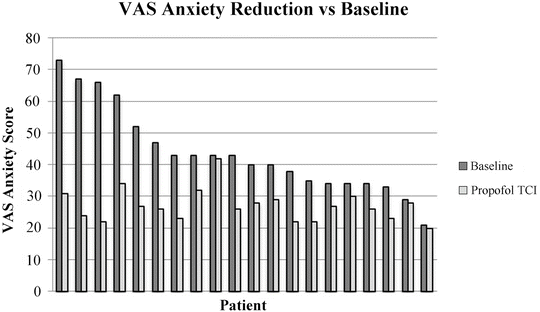
Fig. 21.3
Individual patients ranked by baseline state anxiety measured by visual analogue scale (dark grey column), with subsequent anxiety measurement after propofol premedication. Of note is that the patients with the highest baseline state anxiety had the greatest reduction in anxiety with propofol [49]
The benefits of propofol delivered by patient-maintained sedation compared to standard sedation with midazolam were compared in a large randomised controlled trial by our group [50]. This study was powered to detect a difference in arterial oxygen saturation, and indeed there was a statistically higher SpO2 in the propofol compared to midazolam patients, with the only patient reaching an unsafe level in the midazolam group. Perhaps the most striking result was that propofol was shown to be a superior anxiolytic to midazolam. The VAS recordings of anxiety taken after sedation immediately prior to surgery were compared with baseline anxiety measurements. The propofol patients had almost double the mean reduction in anxiety compared to the midazolam patients (Fig. 21.4). Despite this superior anxiolysis in the propofol group, there was significantly less depression of psychomotor function (as measured by digit-symbol substitution scores) when the patients felt adequately sedated and faster return to baseline after cessation of sedation. Furthermore, despite taking longer to reach readiness for the start of surgery, the faster recovery to meet discharge criteria more than offset this reducing overall sedation time.
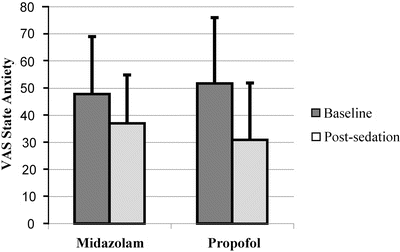
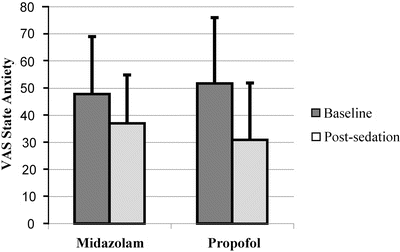
Fig. 21.4
Reduction in baseline anxiety score measured by VAS after receiving sedation with midazolam and propofol delivered by patient-maintained sedation (PMS) [50]
Propofol has potential problems with respiratory depression, reduction in blood pressure and paradoxical excitement [51]. However, experience in over 4000 patients receiving true conscious sedation with TCI propofol and alfentanil analgesia for oocyte retrieval suggests that respiratory and airway events are extremely rare (0.5/1000), as is paradoxical excitement (1.4/1000) [52]. There is no reversal agent for propofol, so with oversedation the drug administration must be stopped, and supportive care provided until the plasma drug level reduces. This reinforces the need for reliable prevention of oversedation and loss of verbal contact.
Its main limitation is that it generally needs a quite sophisticated mechanism of delivery. Intermittent bolus provides fleeting sedation with possible over and undersedation. It has been delivered by patient-controlled sedation [46, 53, 54], by manual infusions [37, 45] and more recently with target-controlled infusions [55, 56]. The measured plasma concentration required to reach a standard sedation level (approximating the loss of verbal contact) varies hugely between individuals, 0.2–2.7 μgċml−1 range [55]. Even after temazepam premedication, this wide range did not change much, but the elderly were more sensitive [56].
Pharmacokinetics : The Difficulty with Propofol Administration for Sedation [57]
Although propofol has many desirable properties, the main problem with its use for sedation is deciding the best mode of delivery. Its three-compartment pharmacokinetics make it obvious that for anything except a simple induction of anaesthesia (bolus dose), quite complicated dose regimens are required to achieve and then maintain a steady plasma concentration [58].
It is known that intermittent boluses provide oscillating plasma concentrations (Cp) and predicted brain concentrations or effect-site concentrations (Ce), and thus alternating overdose and underdose is quite possible (Fig. 21.5). A combination of bolus and continuous infusions is more useful as they provide steadier Cp, Ce and clinical effects. It is relatively easy to calculate a loading dose if we know the desired plasma concentration (Cdesired) and the volume of distribution (Vd). Maintenance infusions require knowledge of clearance and the rate constant values between the compartments of the appropriate pharmacokinetic model. The concept of context-sensitive half-time (the principle that observed half-life is dependent on the duration of the infusion) and its relationship to published redistribution half-life (t1/2α) and elimination half-life (t1/2β) are not straightforward and make matters more complex [59, 60]. In short, the pharmacokinetics involved are complicated and difficult in practice for the clinician [60].
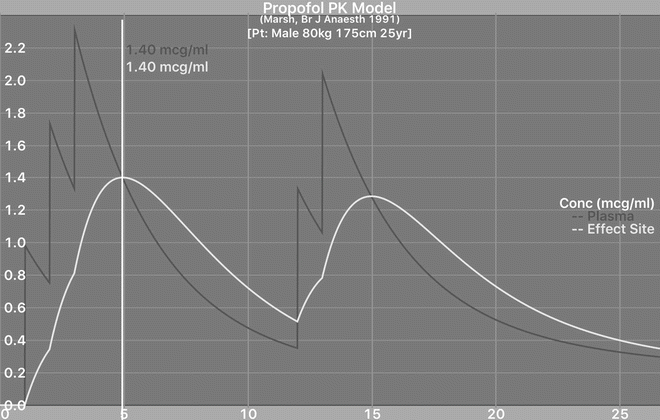
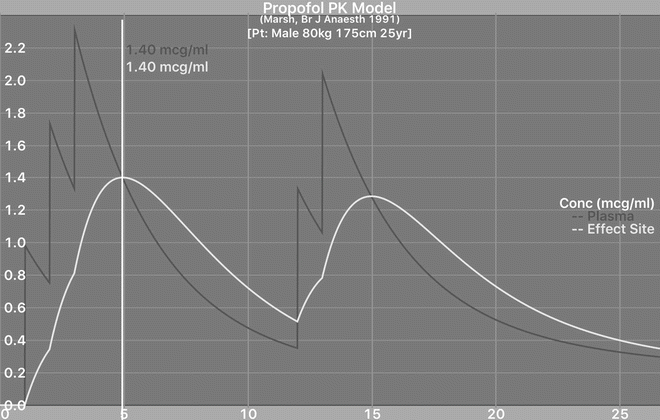
Fig. 21.5
Intermittent boluses of propofol for sedation showing oscillating predicted plasma concentrations and the effect-site concentration (y-axis) lagging behind. Note when the last bolus is given, there is a shorter lag in effect-site concentration than with midazolam. However, after the last dose, both plasma and effect-site concentration fall rapidly. These are calculated using a pharmacokinetic simulator using the Marsh model
Target-Controlled Infusions [61]
These use a pharmacokinetic model and a microprocessor to control delivery of the sedative or anaesthetic drug via a syringe pump. The anaesthetist enters some patient factors such as weight, age, sex and height, some drug factors such as drug concentration and the desired plasma (Cp) or effect-site (Ce) concentration—the “target concentration”. The microprocessor calculates the amount of drug required to achieve that concentration, automatically administering a loading dose, and varying the infusion rate to keep the Cptarget steady. The anaesthetist observes the patient and alters the target up or down if required depending on the clinical response of that patient. The development of TCI techniqu es using microprocessors and pharmacokinetic models such as the Diprifusor™ revolutionised the delivery of propofol infusions in anaesthesia. This made simple delivery of propofol infusions possible for the average anaesthetist.
The actual plasma concentration may not be the same as the calculated concentration; however, it is generally more stable and predictable compared with a manual infusion regimen. Although, there is little evidence for anaesthesia that outcomes are better with TCI [62], anaesthetists have to make fewer adjustments and seem to prefer it [63, 64]. TCI pumps can also calculate predicted brain concentration or “effect-site concentration” [65]. For this it uses a rate constant ke0 that describes equilibration of plasma concentration and clinical effect (usually measured by a depth of anaesthesia monitor). It can also calculate time to reach a predetermined concentration, which can be set by the user.
It seems clear that delivery is simpler using TCI technology and practitioners prefer this to manual infusions. Indeed it did not take long for anaesthetists to apply this technology to the provision of sedation [56], and anaesthetist-controlled sedation using TCI propofol remains a popular technique. Similarly to data from studies providing general anaesthesia with TCI propofol, when it is used for conscious sedation during monitored anaesthetic care, anaesthetists have to make fewer pump adjustments than with manual infusions [66]. The main problem is that non-anaesthetists deliver the majority of sedation in our healthcare system, and they generally do not have access to TCI pumps or training in their use.
Patient-Activated Drug Delivery Systems: PCA, PCS and PMS
Patient-Controlled Analgesia
At a similar time to the development of TCI techniques, patient-controlled analgesia (PCA) was becoming popular in the control of postoperative pain with opioids. The premise being that the patient could self-administer small doses of an opioid when experiencing pain, giving them control over their own pain relief. The main safety feature is the “lockout” which is the period of time when they can’t self-administer any further drug, until the first dose had begun to achieve its effect. PCA opioid was a revolution. Despite patients using similar amounts of drugs to when administered by a nurse and consequently having very similar pain scores and adverse event rates [67], patient satisfaction with these techniques was much better [67, 68].
Patient-Controlled Sedation
The first application of the PCA principle to sedation, and the first report of patient self-administered sedation with propofol “patient-controlled sedation” (PCS ), was in 1991 [53]. When compared with midazolam, propofol seemed a better choice of agent as patients had a feeling of well-being [32, 54], higher satisfaction [34, 54] and faster recovery [32, 34, 54], despite less amnesia and less suppression of psychometric testing [32, 54]. There have been some concerns about restlessness or disinhibition with the use of propofol by PCS [33]; however, others have rated patient cooperation higher [34].
Patient satisfaction seems higher with PCS than when propofol is administered by a physician or nurse either by infusion or bolus [55, 69–71]. Generally anaesthetists give more drug and titrate to deeper levels of sedation [69, 70, 72, 73] and higher plasma concentrations [55]. Accordingly there is less oversedation [55, 69, 70, 72] and less amnesia [70] with PCS. Patients generally prefer self-administration when they try both as a crossover trial [55, 69] or have higher levels of satisfaction when group comparisons are done [70]. This higher satisfaction occurs, even when exactly the same PCS pump with the same dose and lockout is self-administered, compared with when given by a nurse for them [71].
Various approaches have been made to vary the “bolus” dose administered with each button press and the “lockout” period between boluses to achieve optimum sedation, patient satisfaction without oversedation. The first report described a 0.7 mgċkg−1 bolus and a 1 min lockout [54]. There are two broad approaches: a small bolus dose generally less than 10 mg and short or no lockout [46, 74, 75], a larger dose 20–40 mg with a longer lockout of 3–5 min [4, 33, 76–78]. There is also an in-between approach of moderate dose 18–20 mg with a short 1 min lockout [32, 69, 79]. Even within the same group, settings have changed study to study, presumably searching for the perfect balance of access to drug and safety [32, 53, 54, 69]. In the end there are lots of opinions on what is best, but no direct comparisons.
The zero lockout patient-controlled sedation (ZLPCS) is interesting [80]. With this instead of using the lockout to limit the dose of sedative, the maximum infusion rate of the pump is used. The dose rate depends on the physical maximum rate of travel of the plunger on the pump, and to add complexity also depends on the cross-sectional area of the syringe used [81]. Surprisingly it seems that even with unlimited access to propofol, patients deliver less than if an anaesthetist was controlling a TCI pump [55]. Some have made the concept of PCS even more complicated by advocating the principle of fuzzy logic demonstrated in PCA [82] applied to PCS [81].
Many PCS settings use a fixed dose making no attempt to individualise drug administration to the patient. Even using rudimentary pharmacokinetic principles, one would expect patient factors, e.g. weight, to affect the relationship between dose and plasma concentration hence effect.
Patient-Maintained Sedation
Patient-maintained sedation (PMS ) combines the steady plasma concentrations of TCI with the patient-controlled function of PCS [83]. Initially, the system is set to administer propofol to a predetermined target Cp (or more recently Ce). The first reported initial target 1.0 μgċml−1 was based on experience of range of Cp that patients obtain adequate anxiolysis and sedation. It then allows the patient to increase the TCI target by pressing the button. The size of the stepwise increase is determined by the “increment” and was originally described as 0.2 mgċml−1 up to a preset maximum 3.0 μgċml−1. There is a lockout period (originally 2 min) that prevents the patient increasing the target concentration until the clinical effect has had a chance to equilibrate after an increase in Cp. There is usually some programmed condition, e.g. a period of no button presses (6–12 min), that prompts the pump to reduce the target. There is an implied safety feature, similar to the premise used in PCS, that an oversedated patient would not be able to press the button and demand more drug. This was modified to make it more difficult for an uncoordinated sedated patient to increase the Cp. The original versions of PMS required a double click of the button within 1 s to activate an increased Cp. Despite this sedation scores in volunteers encouraged to take as much propofol as possible reflected some oversedation. Subsequent experience in a younger cohort of real patients having dental surgery revealed no loss of verbal contact, high patient satisfaction and cooperation with the surgeon [84]. However, there was one patient desaturated to less than 90 % and an epileptic patient who had three grand mal seizures. Attempts were then made to alter the settings to prevent possible oversedation as reported in the initial study. Despite these technical problems in the early studies, a crossover comparison was performed with PCS; patients seem to prefer PMS [85]. PMS patients reached adequate sedation faster, but this was at the cost of some oversedation. It seems that the theoretical benefits of steadier plasma concentration improved patient satisfaction with the quality of sedation.
Pharmacokinetics and Pharmacodynamics Relevant to Propofol Sedation
The main pharmacological issues when using any microprocessor-delivered drug system (such as TCI or PMS) are the choice of pharmacokinetic model, choice of ke0, and deciding whether to deliver drug to target plasma or effect-site concentration. All versions of the PMS system used the same PK and PD models as the Diprifusor™. It is important to know the suitability of this model in contrast to alternatives.
Pharmacokinetic Models
Most anaesthetists globally became familiar with the use of TCI to deliver propofol with the Diprifusor™ system. The first description of the prototype Diprifusor™ [86] used the Marsh propofol PK model [87] as its basis for drug delivery. The Diprifusor’s PK model was first explicitly described in a study looking at the performance of the adult model used in children [88].
This study reported that this adult PK model was unsuitable for use in children and detailed an alternative data set for use in children. This became known as the Marsh model.
Subsequently other models have been suggested, the main rival model being the Schnider model [89]. This was proposed considerably after the clinical introduction of the Diprifusor™ and about the same time that the first estimates of Cp50 were being reported [90]. Confusingly some of the first open TCI systems available in the UK allowed the Schnider model to be chosen to deliver TIVA even though the predictive performance was not clear at the time, and there were major errors in the implementation in that with a heavy patient, the calculated lean body mass could decrease to zero or less. The importance of knowing the features of the models available and the relative merits of each has been stressed [91]. The major difference between the Marsh/Diprifusor™ model and the Schnider model is the small fixed volume of distribution of the Schnider model, which therefore gives a considerably smaller bolus at induction than the Marsh. Because of the small central volume, the Schnider must be used in so-called “effect” mode, but in reality this model still delivers less drug than the Marsh model in plasma mode. The result is that the calculated effect-site concentration for the Schnider model is incorrect for the first 15 min after changing a target concentration [115]. So an anaesthetist inadvertently using the Schnider model when used to using the Marsh will deliver significantly less drug than they intended. A further major factor is that the Marsh PK model is the only one which has been approved for clinical use by regulatory authorities throughout the world. All of our studies used the Marsh model.
Predictive Performance of Pharmacokinetic Models
It was acknowledged from an early stage that the target concentration (Cptarget) was an estimate of the individual patient’s actual plasma concentration and that measured plasma concentration (Cpmeasured) would be different [92]. The predictive performance of TCI systems should be described in terms of the magnitude and direction of this inaccuracy [93]. The terms used to describe performance error are defined in Table 21.3. The initial study to establish the magnitude of these errors with the Diprifusor™ was published in 1998 [92]. Measured and predicted concentrations from the Diprifusor™ were compared in 46 patients of both sexes between 18 and 80 years old, at different phases of propofol infusion (induction, maintenance and recovery). The performance of the Diprifusor™ system was established by comparing the predicted concentration Cptarget with a laboratory measured value Cpmeasured, for each patient at each time point. The inaccuracy (MDAPE) was 24 %, bias (MDPE) +15 %, divergence −8 % h−1 and wobble at steady state 22 %. These were felt to be clinically acceptable and of similar magnitude to the difference between end-tidal and arterial anaesthetic gas monitoring [94]. The most interesting observation was pattern of errors: measured concentration was higher than predicted during induction and lower than predicted during emergence.
Performance error | Plain language description | |
---|---|---|
Bias Median performance error (MDPE) | (Cpmeasured − Cptarget) × 100/Cptarget The median value as a % and direction | Systematic over or under prediction of a device |
Precision Median absolute performance error (MDAPE) | Median (Cpmeasured − Cptarget) | Size of error/inaccuracy |
Divergence | 1. Slope of the linear regression of absolute performance error (APE) versus time 2. Regression of signed PE against time | Change in performance error over time |
Wobble | The median absolute deviation of PE from MDPE | Failure to keep a steady plasma concentration over time |
Accuracy | Size of the average difference between Cpmeasured + Cptarget |
More recently other models have been proposed: the Schüttler model [95] which was defined after studying the largest number of recruited subjects, 270, and an adapted Diprifusor™ model proposed by White [96] which addresses the difference in clearance between men and women, especially related to aging. Comparisons have been made of these four main pharmacokinetic candidates: Diprifusor™ (Marsh), Schnider, Schüttler and White [97]. Overall median bias (MDPE) was lowest for Schnider at −0.1 % and then Diprifusor™ +2.3 % and worst for White at −12.6 % although the range of bias in patients was remarkably similar. The devil is as always in the detail, as the bias is negligible for the Schnider model because measured concentration is less than predicted in the early phase and greater than predicted at termination of infusion phase cancelling each other out. The Diprifusor™ is the opposite, measured concentration is greater than predicted in the early phase (by around half of the level of Schnider prediction error) and less than predicted at termination of infusion. The overall median inaccuracy (MDAPE) was very similar between all groups at 20–24 % as was the wobble at 14–19 %; however divergence was worst for the Schnider model at +13 %.h−1 compared to 1–2 % for the other models. More recently a similar comparison was performed between Diprifusor™, White and Schnider using the original data from the Swinhoe study [98]. Essentially, overall Diprifusor™ and Schnider have very similar levels of bias (MDPE) 16 and 15 %, respectively, and of inaccuracy (MDAPE) of 26 and 23 %, respectively. The pattern of over and under-reads was the same. The newer White model appears better with a bias of 5 % and inaccuracy of 19 % [98]. None of the models accounted fully for the extent of interindividual variation in propofol clearance, but the improved performance with the White model suggests it may have some advantages over the others. Eleveldt and colleagues have taken an innovative approach to defining the “best” PK model [99]. The models discussed generally describe the best PK parameters for the (often small) group of patients studied in the situation studied. They rightly point out that there is uncertainty of the accuracy of the models with different patients and clinical conditions, and caution should be applied when extrapolating a model to a population different to that from which it was developed. To this end they have created a large data set from 21 previously published studies using opentci.org data and collaborative data sharing between groups. This gave them over 10,000 data points from 660 individuals of with widely varying patient features such as age, BMI and clinical situations. A new PK model was generated using allometric scaling, which when retrospectively tested performed better than all other models for the complete data set. This may be a model that has wider applicability in clinical practice. This needs to be tested prospectively, but initial indicators are that it could be very useful in clinical practice [100].
Applying Population Pharmacokinetics to Individuals
The transformation of a drug dose profile to a plasma or effect-site concentration is done by each individual patient’s body, and drug concentration achieved is a result of their variation from the summary values of the PK model used to guide drug administration. This is present whether using standard dose regimens or TCI technology. Shafer’s group has published simulation studies of a population bearing the same variation as reference pharmacokinetic data and described the coefficient of variation (CV) after bolus dose, fixed rate infusion and TCI. They show that CV is highest for bolus dosing and identical for both types of infusion [101]. These simulation data are backed up with limited retrospective animal data. He addresses the criticism of the performance of TCI systems by pointing out that this is what we would expect by biological variation and less variation than we accept every day with intravenous induction of anaesthesia by bolus dose. Measuring the predictive performance of TCI devices shows the magnitude of biologic variability. However they do not cause the biological variability. It is completely understandable that investigators strive to compare and refine models and produce systems with minimal bias, inaccuracy, wobble and divergence. It is likely that TCI will in fact reduce the impact of biologic variability by accounting for patient factors that influence the concentration achieved from the same dose with increasingly complex models. For instance, a vast array of patient factors have been shown to influence the accuracy of PK and PD models for propofol: from the common known and accepted weight [88, 102]and age [89, 95, 96, 103] to the less known but interesting smoking status [104], alcoholism [105], cirrhosis [106], use of anticonvulsants [107], ethnicity [108] and even phase of the menstrual cycle [109], to the controversial obesity [110–113] and to the speculative cardiac output [114] and eventually perhaps even pharmacogenetics.
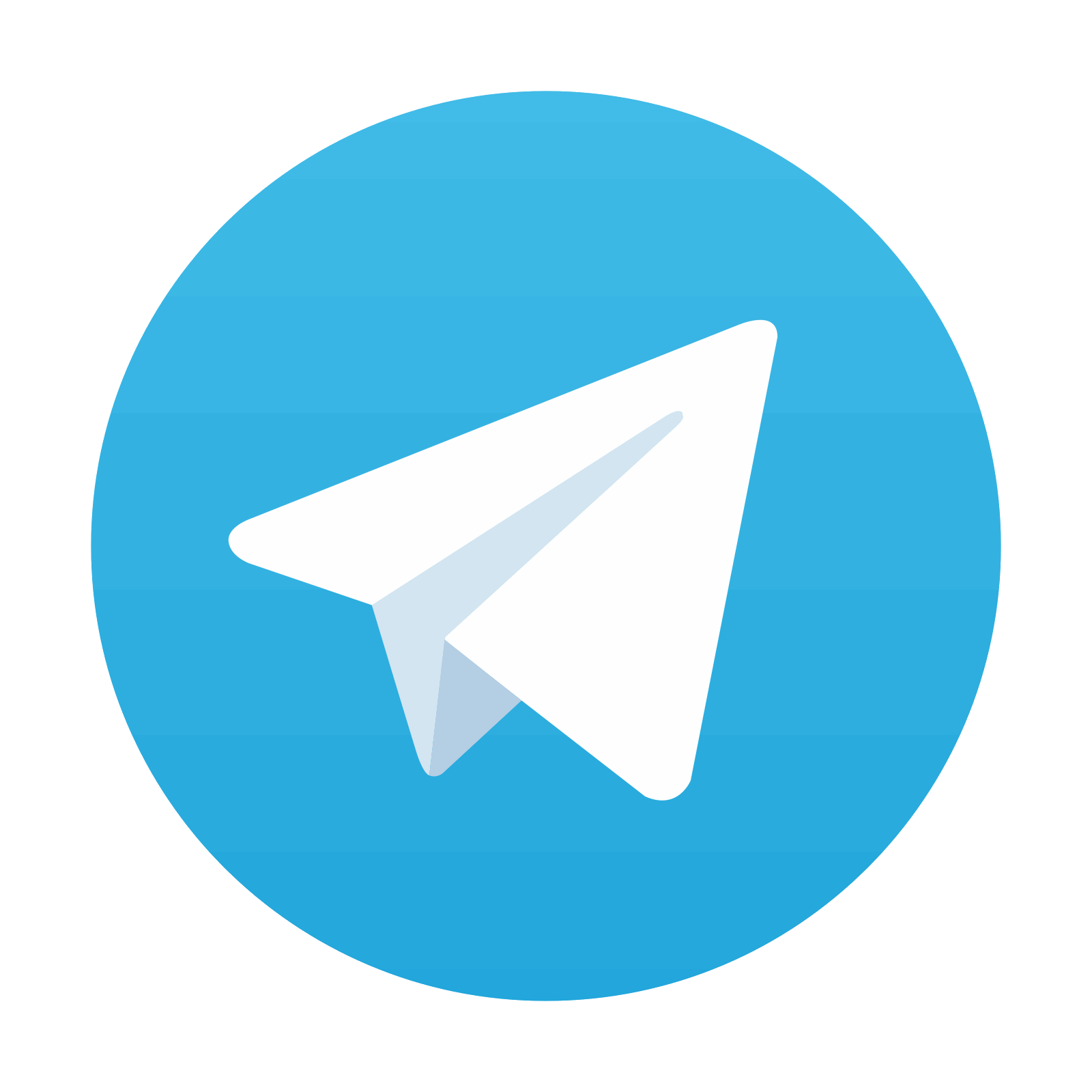
Stay updated, free articles. Join our Telegram channel

Full access? Get Clinical Tree
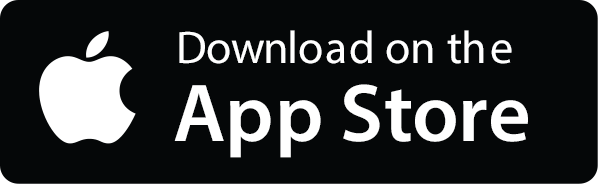
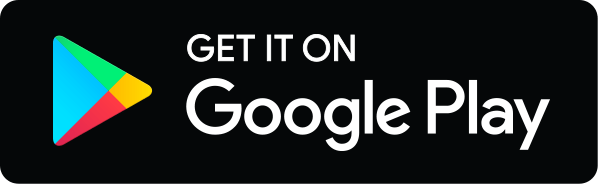