Abstract
Subarachnoid hemorrhage (SAH) is the severe form of hemorrhagic stroke. SAH affects 100,000 people per year in the United States. SAH can be associated with catastrophic and fatal complications. The aim of this review is to present the most recent advances in understanding the pathophysiology of SAH and the perioperative management of a patient’s intracranial aneurysm and SAH. Moreover, we discuss the most up to-date management of cerebral vasospasm following SAH.
Keywords
cerebral vasospasm, intracranial aneurysm, stents, subarachnoid hemorrhage
Case Synopsis
A 58-year-old female patient with a past medical history significant for hypertension, type 2 diabetes, and smoking developed a severe headache and was admitted to the emergency department. Computed tomography (CT) of her head showed a subarachnoid hemorrhage Fisher grade 3. The patient’s cerebral angiogram showed a ruptured wide neck aneurysm in the anterior communicating artery region. The patient underwent endovascular occluding of the aneurysm using a flow diversion system (pipeline embolization device) with coiling. Four days after the procedure the patient developed hemiparesis and impaired mental status. The patient’s transcranial Doppler examination showed increased velocities in the anterior cerebral artery up to 170 cm/s from baseline on admission at 110 cm/s. The diagnosis of cerebral vasospasm and delayed cerebral ischemia was confirmed using CT angiography, which showed vasospasm of small distal cerebral blood vessels. In addition, the patient underwent magnetic resonance imaging (MRI) that showed multiple cerebral infarcts. The patient was managed by oral nimodipine, euvolemia, and induced hypertension.
Problem Analysis
Subarachnoid Hemorrhage
Despite stroke dropping from third to fourth place among the most common causes for mortality, subarachnoid hemorrhage (SAH) from intracranial aneurysm has not declined and still affects 9 per 100,000 people per year in the United States, with about 600,000 cases annually worldwide. The intracranial aneurysm is more predominant in the female gender compared with the male gender by a 3:1 ratio. In addition, female patients have a higher incidence of developing multiple aneurysms. Estrogen is essential for promoting normal endothelial function and its effect on vascular structure, with the fall in estrogen during menopause resulting in a peak of SAH between 50 and 59 years of age. In males, both unruptured and ruptured aneurysms present at an earlier age.
Factors associated with poor outcome are older age, poor neurologic examination on admission, and amount of blood seen on the initial head CT imaging by Fisher grade. The Fisher scale has four grades: 1, 2, 3, and 4. Fisher grade 3 (diffuse subarachnoid blood on CT) itself is significantly associated with the development of cerebral vasospasm. Two clinical scales used for SAH patient evaluation are the Hunt and Hess and the World Federation of Neurological Surgeons (WFNS). Hunt and Hess grade ranges from grade 1, denoting a person who is normal except for mild headache, to grade 5 in a person who is comatose. The WFNS combines the Glasgow Coma Scale and focal neurologic signs for a score of 1 to 5. Higher scores predict poorer outcomes.
Noncontrast head CT is 98% to 100% sensitive for diagnosing SAH within 12 hours, but this rate drops to 93% at 24 hours and may be as low as 57% at 6 days. If the CT scan is negative and clinical suspicion exists, a diagnostic lumbar puncture should be performed.
The blood pressure should be closely monitored and controlled to a maximum systolic blood pressure of 150 to 160 mm Hg in the presecuring phase. The risk of rebleeding within 24 hours of initial SAH may be 15%, with recurrent hemorrhage fatality around 70%. Therefore early treatment of a ruptured aneurysm is recommended to reduce rebleeding and to facilitate treatment of cerebral vasospasm using induced hypertension.
Treatment of Intracranial Aneurysms
Aneurysm treatment is indicated in patients with aneurysmal SAH given the risk of early rebleeding. There are a variety of treatment options for intracranial aneurysms. Microsurgical clipping involves performing a craniotomy to place a metallic clip across the neck of the aneurysm ( Fig. 61.1 ). Endovascular coiling is an alternative to clipping that was introduced in the 1990s, and the number of coiling procedures has greatly increased over the past 2 decades. Coiling involves accessing the aneurysm with a microcatheter and deploying platinum coils to occlude the aneurysm sac ( Fig. 61.2 ). Adjuncts to coiling include balloon- and stent-assisted techniques, which can prevent coil prolapse and decrease the risk of recurrence. More recently, flow-diverting stents have emerged as a useful tool for the treatment of aneurysms that were previously difficult to treat with either clipping or coiling ( Fig. 61.3 ). Flow-diverting stents have a lower porosity than other intracranial stents, which causes stasis of blood flow within the aneurysm, leading to thrombosis and eventually occlusion, while at the same time permitting patency of branch vessels that are covered with the stent.



The choice between treatment modalities is complex and depends on multiple factors, including aneurysm location, aneurysm shape, patient clinical status and comorbidities, and preference of the patient and surgeon. Although there have been three randomized studies comparing clipping to coiling for ruptured aneurysms, there are insufficient data to draw strong conclusions for most aneurysms. The International Subarachnoid Aneurysm Trial (ISAT) is the largest of these trials and found a lower rate of death or dependency in patients who underwent coiling (11%) versus those who underwent clipping (14%); however, only 22% of eligible patients were randomized. Furthermore, the follow-up study of death and clinical outcomes in 1644 patients at 22 UK neurosurgical centers showed that the patients in the coiling group were more likely to be alive and independent at 10 years than were the patients in the clipping group. The Barrow Ruptured Aneurysm Trial (BRAT) reported better outcomes for coiling compared with clipping for patients with posterior circulation aneurysms, but no difference for anterior circulation aneurysms. Aneurysm morphology is an important consideration, with a narrow neck being a favorable characteristic for coiling. Older age, medical comorbidities, and poor clinical grade may favor endovascular therapy as these patients may be at higher risk for adverse events associated with craniotomy.
Clipping tends to be a more durable treatment and has been associated with lower recurrence rates and less likelihood of requiring retreatment. The potential for complications is an important consideration when treating both ruptured and unruptured aneurysms. Common periprocedural complications for patients undergoing neurosurgical or endovascular treatment of aneurysms include intraoperative rupture and stroke. Clipping requires a craniotomy, which adds morbidity and prolonged recovery time. Patients undergoing stent placement (including stent-assisted coiling and flow diverters) require dual antiplatelet therapy for several months to prevent in-stent thrombosis during the period of epithelialization. Furthermore, flow diversion does not offer immediate protection from rehemorrhage. For these reasons, stents and flow diverters are used sparingly in patients with SAH, but they may be necessary in special circumstances.
In summary, there are a variety of options for the treatment of cerebral aneurysms. Although the interpretation of randomized trials of clipping versus coiling is confounded by selection bias and crossover, it appears that coiling has more favorable outcomes for ruptured posterior circulation aneurysms. Surgical decision making continues to rely heavily on operator experience.
Cerebral Vasospasm and Delayed Cerebral Ischemia
Delayed cerebral ischemia (DCI) is a common and serious complication after SAH. DCI occurs in approximately 20% to 40% of patients and is associated with an increased incidence of cerebral infarction and mortality. It is usually caused by vasospasm. Vasospasm is a reversible narrowing of the subarachnoid arteries. It usually occurs from the 3rd to 5th day to the 15th day after the hemorrhage, with the peak at the 10th day. It is observed in 70% of patients on angiographic scans but causes symptoms in only 20% to 30%. Therefore vasospasm primarily describes findings on diagnostic studies, whereas DCI should be used to describe clinical deterioration such as hemiparesis, aphasia, and altered consciousness after SAH diagnosed by a decrease of at least 2 points on the Glasgow Coma Scale.
Angiographic Vasospasm
Angiographic narrowing is mainly due to vasoconstriction caused by subarachnoid blood clot. Angiographic vasospasm always resolves, although arterial fibrosis, endothelial thickening, and reduced compliance can persist. Although arterial narrowing of proximal intracranial vessels can be observed in up to 60% to 70% of patients with SAH, only 30% will experience DCI. Of note, development of DCI depends on the extent and severity of angiographic vasospasm, as well as on preexisting collateral and anastomotic blood flow, cerebral metabolic demand, and blood pressure.
Pathophysiology of Delayed Cerebral Ischemia
Genetic Factors
Plasminogen activator inhibitor–1 (PAI-1) is the main inhibitor of tissue plasminogen activator (t-PA). It inhibits the conversion of plasminogen into active plasmin. The 4G allele in the PAI-1 gene is correlated with higher PAI-1 levels. Elevated PAI-1 levels are associated with poor outcome after SAH as it enhances microthrombosis.
The endothelium nitric oxide synthetase (eNOS) 27 VNTR (particularly the “4a” allele) is significantly associated with the occurrence of aneurysmal SAH. The eNOS T-786C SNP (particularly the “C” allele) is significantly associated with the occurrence of cerebral vasospasm.
Microthrombi
The presence of microthrombi induced by platelet aggregates in parenchymal microvessels after SAH has been identified as one of the main culprits for DCI. The process of platelet activation is associated with the release of proteases such as matrix metalloproteinase 9 that digests collagen IV in the blood vessel lamina. Of note, the constriction of cortical and/or intraparenchymal arterioles usually precedes the thrombus formation either acutely or days after SAH.
In addition to formation of microthrombi within hours of SAH, large-artery angiographic vasospasm induces endothelium injury that enhances platelet aggregation and microthrombi formation. Furthermore, the activation of the clotting process after SAH, which contributes to cessation of the hemorrhage, could generate more emboli in the distal circulation. Finally, the systemic hypercoagulable response to SAH enhances the formation of microthrombi in the cerebral circulation.
Subarachnoid Blood and Inflammation
After SAH, the presence of free hemoglobin in the subarachnoid space induces the expression of cell adhesion molecules in the endothelial cells, which in turn facilitates the migration of leukocytes into the subarachnoid space, inducing inflammation, scavenged nitric oxide (NO), and impaired NO-mediated vasodilation.
The reactive oxygen species (ROS) formed during hemoglobin auto-oxidation constitute a major etiologic factor underlying the development of cerebral vasospasm. ROS oxidize bilirubin to bilirubin oxidation products, which in turn inhibit eNOS and NO production.
Haptoglobin (Hp) is an iron-recycling protein that mediates clearance of extracorpuscular free hemoglobin after hemorrhage. Hp counters hemoglobin toxicity by capturing the released hemoglobin and directing it to CD163 receptor–expressing macrophages, which internalize the free hemoglobin. In humans, two Hp alleles exist and therefore three possible genotypes are possible: Hp 1-1, Hp 1-2, and Hp 2-2. The Hp 2-2 genotype appears to promote a proinflammatory state and is cleared slowly by leukocytes, an effect that greatly prolongs NO scavenging. Therefore patients with the Hp 2-2 genotype are at higher risk of developing symptomatic cerebral vasospasm after SAH. L-citrulline injection to supplement NO in animals with the Hp 2-2 genotype has been shown to prevent vasospasm.
Blood in the subarachnoid space and lipid peroxidation initiate a significant inflammatory response. Activated leukocytes in the cerebrospinal fluid of patients with SAH synthesize release endothelin-1 (ET-1) in addition to other cytokines such as interleukin-6 (IL-6), interleukin-1β, tumor necrosis factor–α, and oxygen-free radicals. The intercellular adhesion molecule–1 (ICAM-1) and vascular adhesion molecule–1 (VCAM-1) are necessary for leukocytes to adhere to the endothelium and enter the subarachnoid space, inducing the inflammatory response after SAH. Cerebrospinal fluid and serum concentrations of ICAM-1 and VCAM-1 are correlated with the development of vasospasm diagnosed by transcranial Doppler. Ibuprofen, which inhibits ICAM-1 and VCAM-1 upregulation, was found to reduce experimental vasospasm. However, the doses of ibuprofen required to prevent ICAM-1 and VCAM-1 upregulation can produce systemic toxic effects. Ibuprofen polymers implanted into the subarachnoid space releasing high and controlled sustained drug concentrations in a monkey SAH model prevented the development of angiographic vasospasm without signs of local or systemic toxicity. However, despite the detrimental effects of inflammation process after SAH, it can have beneficial effects in repairing processes after SAH.
Blood-Brain Barrier Disruption
Blood-brain barrier disruption after SAH probably contributes to brain injury after SAH, but it might have beneficial effects. Therefore activation of matrix metalloproteinases that acutely disrupt the blood-brain barrier may facilitate brain repair at a later stage.
Cortical Spreading Ischemia
Cortical spreading depression (CSD) has been posed as the putative mechanism for DCI after SAH. CSD is a depolarization wave in cerebral gray matter that propagates a slow velocity of 2 to 5 mm/min. This mass depolarization of cells results in the redistribution of ions and neurotransmitters, leading to a cycle that ends in neuronal inactivation followed by a period of transient depression in the cortex. In the normal brain, CSD is usually associated with an increase in regional cerebral blood flow (CBF) and oxygen delivery as a means of delivering the necessary energy required for the ionic pumps driving neuronal repolarization. However, after SAH, the hyperemia coupled with CSD does not occur, leading to a reduction of CBF and inducing the spread of ischemia.
Patients with SAH after clipping of ruptured cerebral aneurysm developed DCI associated with clusters of CSD despite the absence of angiographic vasospasm in some patients. The number and duration of CSD clusters correlated significantly with the development of DCI.
CSD is accompanied by a marked rise in cytosolic calcium. It depolarizes neuronal mitochondria and triggers the rise in oxygen use via the calcium uniporter mitochondria membrane. CSD also activates glycolytic pathways, which increase cortical lactate production. An increased extracellular potassium level due to erythrocyte lysis directly depolarizes arteriolar smooth muscle cell and increases intracellular calcium via voltage-gated calcium channels, thus causing vasoconstriction. Furthermore, depolarization of neurons removes the magnesium block of N -methyl- d -aspartate (NMDA) receptors and sensitizes the receptor to small increases in interstitial glutamate. The interaction of glutamate with the NMDA receptor triggers further release of potassium and glutamate with further propagation of the CSD. The presence of blood in the subarachnoid space avidly scavenges NO and enhances the development of CSD. Arterial hypotension prolongs the duration of CSD, whereas hypertension reduces it. Therefore maintaining cerebral perfusion pressure after SAH is very important to mitigate the harmful effects of CSD after SAH.
Predictors of Delayed Cerebral Ischemia
Thick subarachnoid blood clots, poor clinical condition on admission, and loss of consciousness at ictus increase the risk of DCI. Strong evidence suggests that smoking increases the risk of DCI, and moderate-strength evidence indicates that diabetes, systemic inflammatory response syndrome, hyperglycemia, and hydrocephalus also increase the risk. ET-1, IL-6, and some markers of thrombin activation in the cerebrospinal fluid might be useful for predicting DCI after SAH.
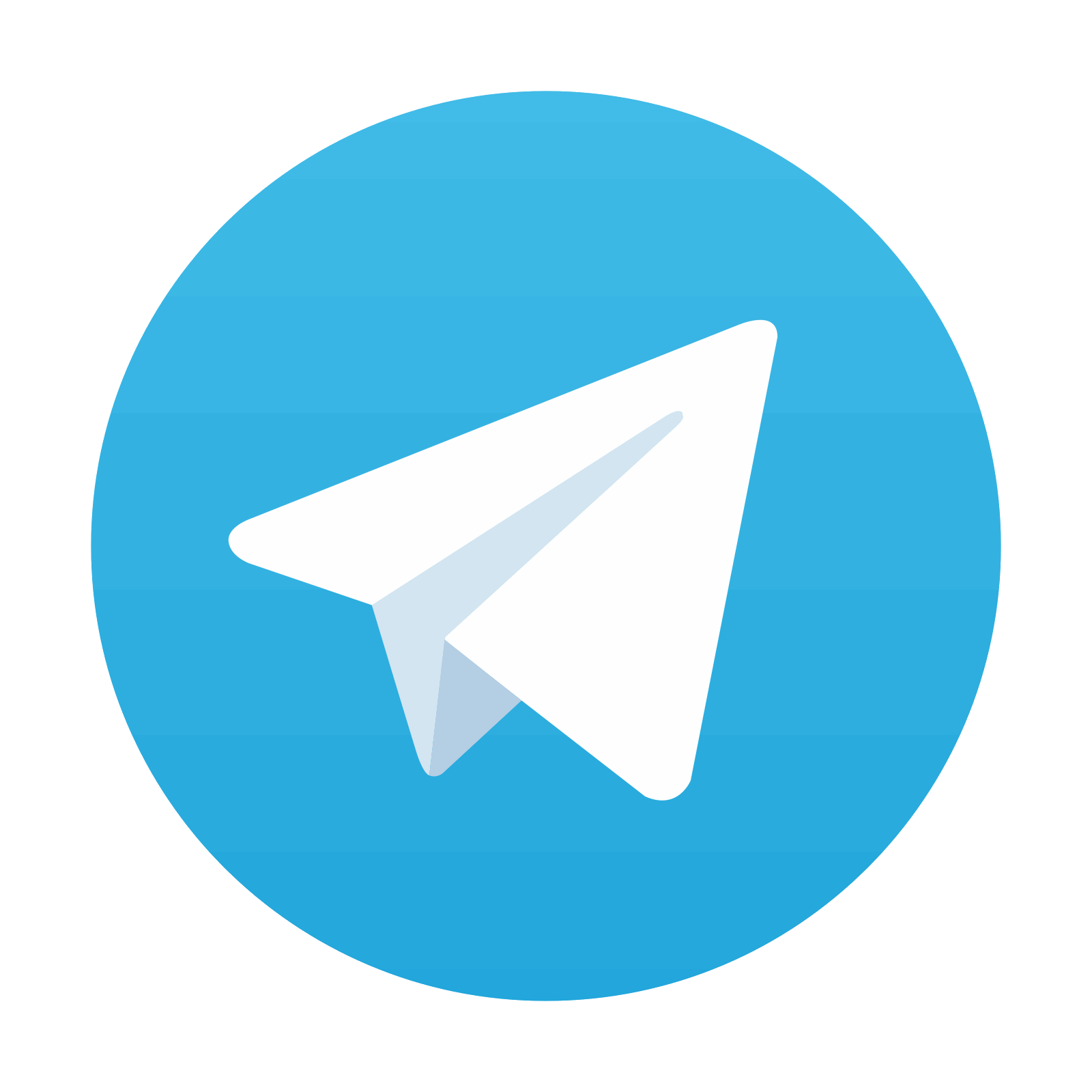
Stay updated, free articles. Join our Telegram channel

Full access? Get Clinical Tree
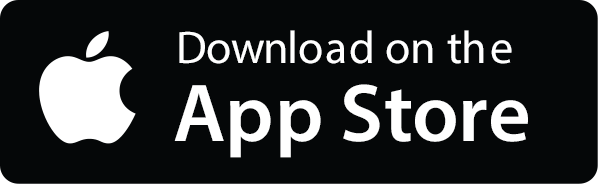
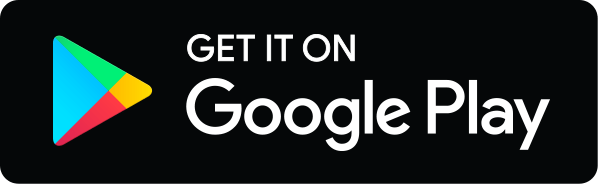