41 Intensive Care After Neurosurgery
Overview
The benefit of concentration of care in units with sufficient case volume has been well established in different fields of intensive care medicine including trauma,1 neonatology, and specifically neurointensive care.2,3
Treatment of patients with spontaneous intracerebral hemorrhage in a neurointensive care unit is associated with reduced mortality when compared with patients admitted to a general intensive care unit (ICU).4,5 Mortality following aneurysmal subarachnoid hemorrhage (SAH) is lower in centers with a higher case volume.6 Patel and colleagues7 unequivocally showed a 2.15 times increase in the odds of death (adjusted for case mix) for patients with severe traumatic brain injury (TBI) treated in non-neurosurgical centers versus neurosurgical centers. Their report makes a strong case for transferring and treating all patients with severe head injury in a setting with 24-hour neurosurgical facilities. Protocol-driven approaches also improve results.8–10
Priorities and Goals of Postoperative Neurosurgical Care
Postoperative complications may be systemic or neurosurgical (Table 41-1).
TABLE 41-1 Postoperative Complications
Systemic Complications | Neurosurgical Complications |
---|---|
Coagulation disorders: blood loss, disseminated intravascular coagulation, drug induced Thromboembolic: DVT, pulmonary embolism, myocardial infarction Pulmonary: atelectasis, pneumothorax Hypovolemia: insufficient pre- and perioperative hydration, blood loss Infection: pneumonia, urinary tract infection, catheter sepsis Metabolic: hyperglycemia [steroid induced], diabetes insipidus, hyponatremia Air embolism: sitting position, opening of large cerebral veins during surgery Pressure sores and decubitus ulcers: intraoperative positioning, cervical traction, paraplegia | Postoperative hematoma: subgaleal, epidural, subdural, intraparenchymal Cerebral ischemia: subarachnoid hemorrhage, vasospasm, vessel occlusion Brain swelling: edema, vasodilation Infection: meningitis, subdural empyema, cerebral abscess Seizures: infection, depressed compound skull fracture, cortical lesions Hydrocephalus: obstruction/resorption Tension pneumocephalus CSF fistula Inverse cerebellar herniation Cranial nerve lesions |
CSF, cerebrospinal fluid; DVT, deep venous thrombosis.
Prevention and Management of Systemic Complications After Neurosurgery
General Principles and Second Insults
The prevention and management of systemic complications after neurosurgical procedures follows general principles of “intensive care” medicine. It is, however, important to realize that systemic complications and second insults may initiate or aggravate cerebral damage. Aggressive treatment aimed at preventing and limiting second insults is of paramount importance. The main second insults, their causes, and adverse effects on brain homeostasis and function are summarized in Table 41-2, further illustrating the complex interactions between systemic events and CNS function.
TABLE 41-2 Systemic Second Insults
Event | Main Causes | Adverse Effects |
---|---|---|
Hypoxemia | Hypoventilation Aspiration atelectasis Pneumothorax Pneumonia Anemia | Decrease in oxygen delivery and increased risk of ischemic damage |
Hypotension | Hypovolemia | Decreased CPP, decrease in CBF, increased risk of ischemia |
Cardiac failure | ||
Sepsis, spinal cord injury | ||
Anemia | Blood loss | Decrease in oxygen transport and delivery and increased risk of ischemic damage |
Hypercapnia | Respiratory depression | Increased CBV, raised ICP |
Hypocapnia | Hyperventilation, spontaneous or induced | Cerebral vasoconstriction with increased risk of ischemic damage |
Hyperthermia | Hypermetabolism, stress response, infection | Metabolic requirements may exceed substrate delivery, resulting in energy depletion |
Central dysregulation | ||
Hypothermia | Exposure, central dysregulation | May be neuroprotective but can cause significant coagulopathy and electrolyte disturbances |
Hyperglycemia | IV infusion of dextrose, steroids, stress response | Acidosis, electrolyte disturbances |
Hypoglycemia | Inadequate nutrition, insulin overdose, pituitary insufficiency | Energy depletion in the brain, seizures |
Hyponatremia | Inadequate salt intake (hypotonic fluids) Excessive sodium loss (cerebral salt wasting/CSF drainage) Syndrome of inappropriate ADH | Increased edema, seizures |
Hypernatremia | Diabetes insipidus | |
Osmotic agents (mannitol, hypertonic saline) | Lethargy, coma |
ADH, antidiuretic hormone; CBF, cerebral blood flow; CBV, cerebral blood volume; CPP, cerebral perfusion pressure; CSF, cerebrospinal fluid; ICP, intracranial pressure; IV intravenous.
Cardiac Dysfunction
Electrocardiographic (ECG) abnormalities, usually diffuse ST-segment changes mimicking cardiac ischemia and cardiac arrhythmias, may be caused by SAH, TBI, or raised ICP. The devastating effects of a sudden catecholamine release following acute intracranial bleeding have recently received further attention. The left ventricle suffers a typical bulging (indicating ischemic changes and functional impairment) which has been awarded the term Takotsubo syndrome, with reference to the shape of a pot used by ancient Japanese fishermen for catching octopus. The extent of left ventricular dysfunction is variable, but it may lead to extreme cardiac failure and pulmonary edema.11,12
Neurogenic Pulmonary Edema
The development of neurogenic pulmonary edema has been described early in the postoperative period after a variety of neurosurgical procedures, including brain tumors (particularly those resected in the posterior fossa), cysts, hydrocephalus, intracranial hemorrhages, and brainstem lesions.13–16 Although an infrequent event, this is potentially life threatening and requires rapid evaluation and emergent therapy in the ICU. A 9% mortality rate directly attributable to neurogenic pulmonary edema has been reported in a recent review of this condition. Generally this complication appears in the initial 4 hours after the neurologic event and is more common in women than in men, possibly related to the preponderance of cases in patients with SAH.16 The mechanisms underlying this condition are unclear; a sudden central sympathetic discharge may trigger pulmonary venoconstriction, systemic arterial hypertension, increased left ventricle afterload, increased capillary permeability in the pulmonary vascular bed, and simultaneously cause cardiac ischemia and ventricular failure.12,17 Because of these multiple mechanisms, neurogenic pulmonary edema can be interpreted as noncardiogenic or, at least in part, as cardiogenic.18 Both low and high protein content have been reported in the edema fluid.16,19 It is commonly associated with raised ICP, so in addition to therapies directed at intracranial hypertension, therapeutic measures are mostly supportive. To attenuate the massive sympathetic discharge, opioids and sedatives are used. Supplemental oxygen is uniformly required, and tracheal intubation with mechanical ventilation and application of positive end-expiratory pressure (PEEP) has been reported in about 75% of patients.16 Diuretics have been used, provided volume status is adequate, but diuresis causes less effect than in cardiac edema. Most patients require vasoactive drugs.19
Hypercoagulopathy and Thrombosis Prophylaxis
Release of factors from damaged brain tissue may induce local and systemic hypercoagulopathy.20–22 Various studies have confirmed a transient hypercoagulopathy syndrome both in the immediate postoperative phase after brain surgery and in patients with TBI.20,23–25 In patients with a subdural hematoma, consumption of clotting factors may lead to coagulopathy in up to 22% of the patients.26
Deep venous thrombosis (DVT) has been reported to occur in 18% to 50% of neurosurgical cases27 and pulmonary embolism (PE) in 0% to 25%. DVT and PE incidence is particularly high in brain tumor patients. Nevertheless, neurosurgeons tend to underestimate the risk of DVT and PE28 and are sometimes reluctant to routinely prescribe anticoagulant prophylaxis for fear of increasing the risk of postoperative bleeding.29 Options for prevention of thrombosis prophylaxis in neurosurgical patients include both mechanical (graduated compression stockings, intermittent pneumatic compression stockings) and pharmacologic (low dose of classic heparin and low-molecular-weight heparin) therapies. Intuitively, mechanical therapies carry less associated risk, but pharmacologic approaches are more effective in preventing thrombotic complications. Various studies have indeed shown a higher incidence of postoperative hemorrhagic complications,30 but not all are clinically relevant.
Overall, existing evidence, however, shows that the beneficial effects in reducing DVT and in particular PE31,32 outweigh a slightly increased risk of clinically significant hemorrhagic complications with anticoagulant prophylaxis.
These data support the administration of antithrombotic prophylaxis to patients undergoing neurosurgical procedures,33 including those with intracranial hemorrhagic lesions,34 closed TBI,35,36 or high-risk trauma patients.37,38 It has been recommended to remove catheters or drainage tubes in the postoperative phase when anticoagulant effects are low (e.g., just prior to administration of next dose).39
Prevention and Management of Neurosurgical Postoperative Complications
Supratentorial Procedures
Postoperative Subgaleal Hematoma
Postoperative subgaleal hematoma may occur in up to 11% of procedures. These hematomas generally result from inadvertent damage of the superficial temporal artery, inadequate hemostasis, or hemorrhage from the temporal muscle. If the superficial temporal artery is damaged during the operation, ligation is preferred over coagulation. The occurrence of subgaleal hematomas can be minimized by routine use of postoperative wound drainage for 24 hours. Reoperation for subgaleal hematomas is seldom necessary unless there is a communication with the intracranial compartment, with secondary compression of the brain.40
Intracranial Hemorrhage
Parenchymal hemorrhages are the most frequent cause of postoperative hematomas following supratentorial procedures and generally occur at the site of operation, particularly following partial tumor resection. An increase in systemic BP at the end of surgery may increase the risk of parenchymal hemorrhage. In rare cases, the hematoma may be located distant from the primary site of operation, and cerebellar hematomas have even been described after supratentorial surgery.41,42 The possibility of a postoperative hematoma should be considered in all patients who are not fully alert post anesthesia, as well as in those who exhibit secondary deterioration.
Postoperative Brain Swelling
Modern neuroanesthesiology techniques have diminished the incidence of peri- and postoperative brain swelling. Nevertheless, significant swelling may sometimes occur, causing surgical difficulties and possibly critical problems in the ICU. Predisposing factors are hypercapnia, arterial hypertension, hyponatremia, obstruction of venous drainage, and silent or overt seizures during surgery or in the immediate postoperative phase. Further significant brain swelling after uneventful surgery has been attributed to intracranial hypotension caused by subgaleal suction.42,43 In any patient with brain swelling during the surgical procedure, the possibility of a deep hematoma should be considered, and an urgent computed tomography (CT) scan should be performed. Brain swelling due to vasodilation can be corrected by hyperventilation and barbiturate administration; brain swelling due to cerebral edema should preferentially be treated by mild hyperventilation and osmotic agents.
Tension Pneumocephalus
Some air collection is generally observed on postoperative CT scans.44 In rare circumstances, the rewarming of air in the intracranial compartment postoperatively or continuous air leakage due to a cerebrospinal fluid (CSF) fistula of the skull base may lead to a tension pneumocephalus, with clinical symptomatology including decreasing level of consciousness, signs of raised ICP, and occasionally seizures. Generally, postoperative air accumulations are self-limiting and do not require specific treatment.
Seizures
An epileptic seizure in the direct postoperative phase should be considered a serious complication that may cause significant deterioration secondary to vasodilation, increased cerebral oxygen consumption, and increased brain edema. Occult seizure activity can occur in 15% to 18% of patients with moderate and severe TBI.45 The benefits of prophylactic antiseizure medication should be balanced against risks. In some centers, routine prophylaxis is prescribed in all patients undergoing supratentorial brain surgery. In others, the indications are restricted to patients with a higher risk:
Infratentorial Procedures
The care for patients in the direct postoperative phase following infratentorial procedures poses specific problems. Postoperative complications in the posterior fossa can lead to rapid deterioration because of the relatively small infratentorial volume reserve and the immediate compression of the brainstem, resulting in respiratory insufficiency and acute herniation. Irritation of the brainstem may induce large swings in arterial BP, enhancing the risk of postoperative hemorrhage during hypertensive episodes. Cranial nerves are more susceptible to damage due to surgical manipulation than peripheral nerves.46 Lesions of the lower cranial nerves may lead to a diminished gag reflex, with increased risk of aspiration and pneumonia. After surgery in the cerebellopontine angle, specific attention should be paid to the function of the trigeminal and facial nerves and prophylactic measures to prevent damage of the cornea taken.
After posterior fossa surgery, some patients may develop a syndrome of aseptic meningitis.47 This is characterized by meningeal symptoms, headaches, and an inflammatory response of the CSF in the absence of evidence for infection. The origin of this syndrome has not been fully clarified, but symptoms may resolve sooner with intermittent CSF drainage.
An infrequent transitory complication observed after resection of large midline posterior fossa tumors is cerebellar mutism.47 The exact cause is poorly understood, but a vascular phenomenon has been hypothesized.48
Cerebrovascular Procedures
Postoperative care for patients undergoing cerebrovascular surgery poses specific challenges in neurointensive care. In patients operated for arteriovenous malformations, the risk of seizures is particularly high, and focal deficits may occur secondary to changes in cerebral hemodynamics. Following treatment for a cerebral aneurysm, medical and cerebral complications can occur either related to the disease or to treatment (surgical clipping or endovascular coiling). Medical complications specifically linked to SAH are neurogenic pulmonary edema, cardiac arrhythmias, and ventricular failure.11 Electrolyte disturbances, in particular hyponatremia, are also frequently observed.49
The main cerebral complications are:
Rebleeding occurs mainly in the first weeks after the aneurysmal rupture, and current approaches are to prevent rebleeding by early surgical clipping or endovascular obliteration of the aneurysmal sack. Delayed cerebral ischemia, often due to vasospasm is—besides rebleeding—the most common cause of death and disability due to SAH. The reported incidence of this complication varies widely, but angiographic vasospasm is seen with angiography in over 67% of untreated patients at the time of maximum spasm around the end of the first week.50
Delayed cerebral ischemia (DCI) is considered to be caused by vasospasm. However, not all patients with DCI have vasospasm. Inversely, not all patients with vasospasm develop clinical symptoms and signs of DCI. Recent studies show that DCI cannot always be attributed to vasospasm but more to the occurrence of microthrombosis.51,52 DCI is associated with an activation of the coagulation cascade within a few days after SAH, preceding the time window during which vasospasm occurs. Furthermore, both impaired fibrinolytic activity and inflammatory and endothelium-related processes may lead to the formation of microthrombi, further promoting the development of DCI.
Clinically evident delayed ischemic deficits (DID) affect approximately one third of patients. Various studies have shown a beneficial effect of the administration of oral calcium antagonists in preventing DID.53,54 Beneficial effects of intravenous administration of nimodipine remain unproven.
Following evidence that patients with SAH had reduced blood volume, plasma volume, and erythrocyte mass, triple-H therapy (hypervolemia, hypertension, and hemodilution) was proposed for both prophylaxis and treatment of DID after SAH. Various studies have shown a reduction of DID with triple-H prophylaxis,55 but some debate remains.56,57
The usefulness of triple-H treatment is generally accepted, but it has never been unequivocally demonstrated by a randomized controlled trial to be superior to simple moderate fluid loading. The relative importance of the three components of triple-H therapy is uncertain.58,59 Adequate fluid loading should be considered the most important aspect of early treatment and prophylaxis of DID, but it may be considered reasonable to reserve the more vigorous loading and induced hypertension for situations in which there is clinical evidence of delayed ischemia.59–61
Progressive signs of DID may require more aggressive approaches including angioplasty.62 Transluminal balloon angioplasty is generally recommended, but this requires special equipment and a highly skilled and experienced interventional neuroradiology team. Alternatively, “chemical angioplasty” in which the angiography catheter is used to instill papaverine or nimodipine may be considered.63
Chemical angioplasty often has to be repeated within hours or days and carries complications including pupillary changes, seizures, or respiratory arrest with vertebral artery injection. Alternatively, possibilities of cisternal therapy should be considered, injecting recombinant tissue plasminogen activator (tPA) or urokinase in the basal cisterns to break down the accumulated blood,64 or even nitric oxide donors to improve vascular tone.
Various studies have shown clinical benefit of this approach, with the added benefit of reducing the incidence of hydrocephalus. Acute hydrocephalus after SAH is not uncommon. The reported frequency depends on the criteria used for the diagnosis and ranges from 9%65 up to 67%.66 Spontaneous improvement of hydrocephalus has been reported in approximately half of patients with acute hydrocephalus and impaired consciousness on admission, but it may be difficult to predict spontaneous improvement, because treatment is generally instituted. Evidence exists that in the absence of a hematoma with mass effect or an obstructive element, serial lumbar punctures may be the initial optimal method of treatment, reserving continuous CSF drainage procedures for patients in whom the hydrocephalus does not resolve over time.
Admission Examination and Monitoring in the Intensive Care Unit
Specific care and monitoring of the postoperative neurosurgical patient requires accurate knowledge of the preoperative situation and the intraoperative procedure, including the surgery, anesthesiology, and any surgical complications or difficulties. Pertinent aspects are summarized in Table 41-3.
TABLE 41-3 Postoperative Intake After Neurosurgical Operations
Preoperative situation | Neurologic deficit (level of consciousness, focal paresis, cranial nerve lesions, hormonal deficits) Preexisting disease (especially pulmonary and cardiac) Preoperative medication History of seizures Allergy |
Intraoperative details (anesthesia) | Narcotic agents and antagonists Blood loss and substitution Intraoperative laboratory values Intraoperative second insults, diabetes insipidus, etc. |
Intraoperative course (surgical) | Indication, approach, and duration of surgery Surgical position Surgical difficulties and complications (brain swelling, difficult hemostasis, temporary or definite vascular occlusion, opening of air sinus) Immobilization/positioning of patient |
Postoperative instructions (surgeon and anesthetist) | Postoperative medication (e.g., anticonvulsants, antibiotics, steroids, mannitol, antithrombosis prophylaxis) Instructions for postoperative care and monitoring Instructions for removal of drainage, tubes, and stitches Preferred duration of postoperative artificial ventilation Instructions for follow-up CT or MRI examination (if indicated) |
On admission, a full examination of the patient is required; wherever possible, this includes assessment of level of consciousness and neurologic functioning. Medical care for the patient should be provided in joint collaboration between the intensivist and neurosurgeon. Intensive care monitoring includes clinical surveillance, technical monitoring, and follow-up CT or magnetic resonance imaging (MRI). Various approaches to monitoring are summarized in Table 41-4.
TABLE 41-4 Postoperative Monitoring After Intracranial Procedures
Clinical surveillance | Level of consciousness (Glasgow Coma Scale), pupillary reactivity, focal deficits, cranial nerve lesions |
Systemic monitoring | Electrocardiogram and heart rate, respiration, pulse oximetry, end-tidal CO2, blood pressure (invasive, noninvasive), temperature, central venous pressure, Swan Ganz catheter |
Brain-specific monitoring | Intracranial pressure and cerebral perfusion pressure, jugular oximetry, brain oxygen tension monitoring, microdialysis, transcranial Doppler, electroencephalogram, evoked potentials |
Accesses | Central or peripheral venous catheter, arterial catheter, urinary catheter, gastric tube |
Laboratory examinations | Blood gases, hematology, electrolytes, glucose and on indication coagulation status |
Imaging examinations | Chest radiograph (ventilated patients and after lung procedures) Computed tomography or magnetic resonance imaging follow-up (as required) |
Early Evaluation
The level of consciousness should be assessed by the Glasgow Coma Scale (GCS).67 In this scale, standardized assessment of three aspects of responsiveness is performed: the eye, motor and verbal reaction (Table 41-5).
Eyes | Motor | Verbal |
---|---|---|
1. None | 1. None | 1. None |
2. To pain | 2. Abnormal extension | 2. Incomprehensible (groaning) |
3. To speech | 3. Abnormal flexion | 3. Inappropriate |
4. Spontaneous | 4. Flexion (withdrawal) | 4. Disoriented, confused |
5. Localizing | 5. Oriented | |
6. Obeying commands |
NOTES: The best score for each response should be documented and communicated in the format described above. Assessment of the best motor score is based on the best response of the arms. For use in individual patients, separate description of the three components of the Glasgow Coma Scale (GCS) is strongly recommended. For purposes of classification, the total GCS can be calculated by adding the best score obtained in each category. The GCS should be annotated to indicate confounding factors: T signifies an intubated patient; S, sedation; P, neuromuscular blockade.
When administration of painful stimuli is necessary to assess the level of responsiveness, standardized administration is required: pressure on the nail bed and supraorbital pressure to test the localizing response of the motor scale (Figure 41-1).
Accurate determination of the full GCS is not always possible because of sedation and paralysis, but when possible, at least the best motor score should be recorded. Approaches to daily interruption of sedation that allow intermittent wake up in ventilated patients not only help care providers to monitor neurologic status but also have been shown to result in better outcome.68 Some authors advocate imputing the eye and verbal scores from the motor score in sedated and/or ventilated patients.69
Pressure on the oculomotor nerve leads to a loss of function of the parasympathetic fibers, causing a diminished pupillary response or absent pupillary reactivity, generally initially on the side of a lesion (Figure 41-2). With progressive increase in pressure, both pupils become dilated and unresponsive to light. In patients with a lesion of the optic nerve, the consensual light reflex—contraction of the pupil when a light is shone into the opposite eye—remains intact.
Systemic Monitoring: Cardiopulmonary, Respiratory Status, and Temperature
Hypovolemic shock is most common in the setting of multisystem injury or intraoperative blood loss with inadequate replacement. It is important to recognize that tachycardia and signs of peripheral vasoconstriction such as skin pallor and poor capillary refill may precede a drop in BP. Treatment is rapid fluid resuscitation employing isotonic crystalloid fluids, volume expanders, small-volume resuscitation (hypertonic saline), and blood transfusions. Central venous pressure monitoring, or preferably pulmonary artery catheterization, can guide the use of intravenous fluids and vasopressor therapy, aiming for a pulmonary artery wedge pressure of 12 to 14 mm Hg to optimize organ perfusion. After initial volume resuscitation, we suggest a hematocrit of approximately 30% to 33% as optimal in the acute postoperative period in patients in the neurosurgical ICU. Although debate still exists, available evidence suggests that restrictive blood transfusion strategies may be less appropriate in neurointensive care.70–74
After intracranial or spinal cord procedures, we would advocate a more liberal use of blood transfusions than generally recommended in intensive care medicine, aiming at a hemoglobin of at least 5.5 to 6.0 mmol/L (9-10 mg/dL) in order to promote adequate oxygenation of the CNS. This corresponds to the recommendations proposed by Goodnough et al.75 in case of ischemia.
Temperature monitoring is also important, since hypothermia can depress neurologic function to the point of obtundation or coma. Conversely, fever, by increasing metabolic requirements, may exacerbate secondary injury. Mean energy expenditure may be increased up to 200% in patients following brain injury,76 and it would therefore appear appropriate not to risk increasing metabolic requirements even further. Consequently, we recommend that core temperature should be kept lower than 38.0°C, using medications (e.g., acetaminophen, paracetamol, diclofenac) and surface or intravascular cooling.
Hypothermia may be due to adrenal or pituitary insufficiency, hypothalamic disorders, hypoglycemia, or intraoperative exposure. Deliberate hypothermia is sometimes used in complicated cerebrovascular procedures and as second-tier therapy in patients with TBI to reduce ICP. For the indication TBI, hypothermia has been shown to effectively reduce ICP, but uncertainty still exists whether this may translate into an improvement of functional outcome.77,78
Various approaches to cooling have been adopted, but the most frequently used employ surface cooling or gastric lavage with cold fluids. Marion79 reported favorable results with the use of devices for intravascular cooling, and this technique can be expected to become standard for induction of hypothermia in the near future.
Hypothermia has been associated with several complications including cardiovascular instability (mainly arrhythmias), coagulopathy, electrolyte shifts, fluid overload, and increased risk of infection and shivering.80,81 The management of a patient treated with hypothermia over longer periods of time for control of raised ICP can be much more complex than the use of short-term hypothermia post cardiac arrest. Ideally, normothermia could represent the best tradeoff between the dangers of hyperthermia and the complexities and side effects of hypothermia. In practice, a recent trial in neurointensive care comparing conventional treatments with prophylactic normothermia has failed to show benefit.82
< div class='tao-gold-member'>
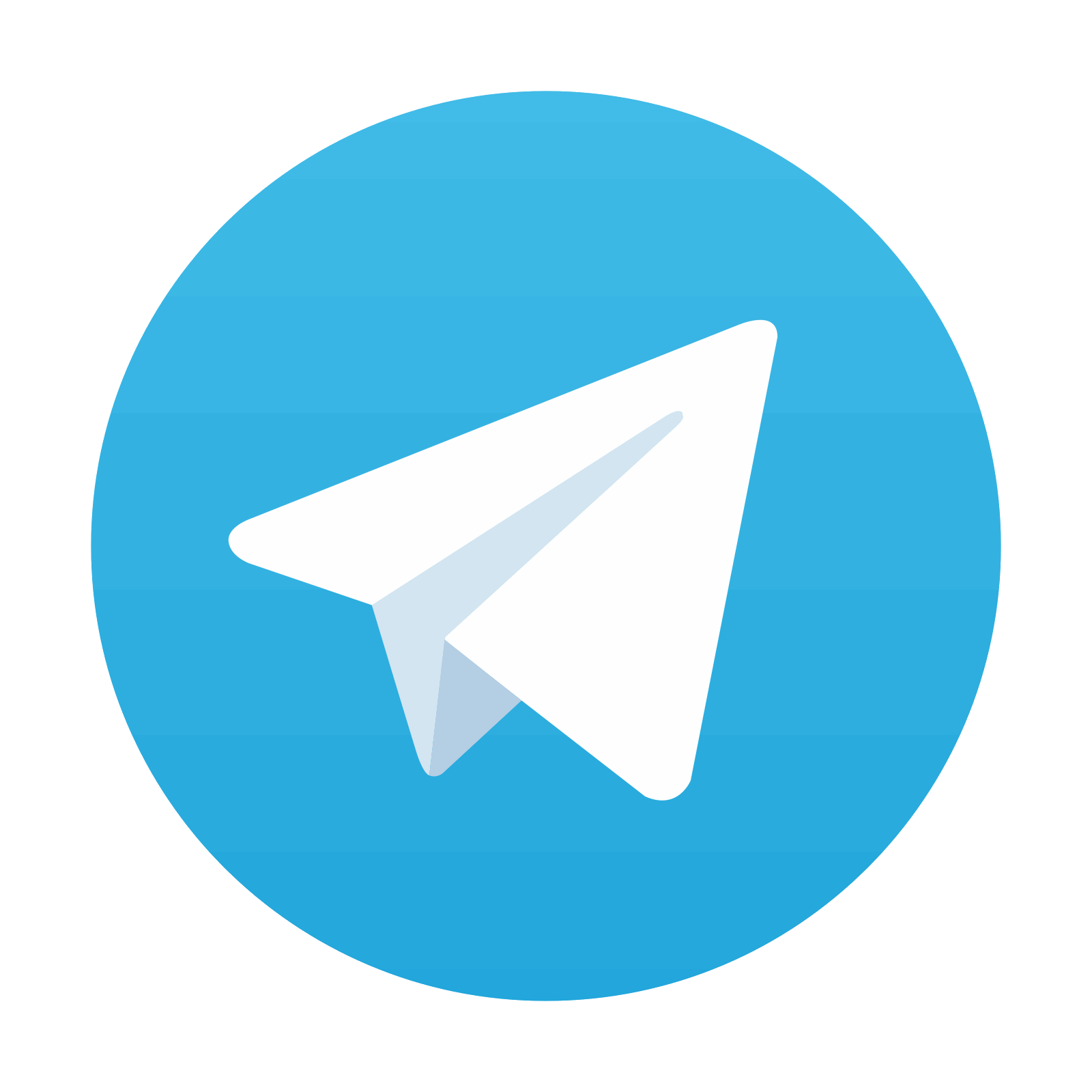
Stay updated, free articles. Join our Telegram channel

Full access? Get Clinical Tree
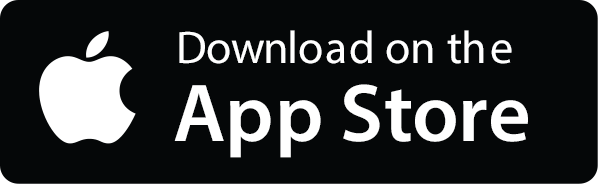
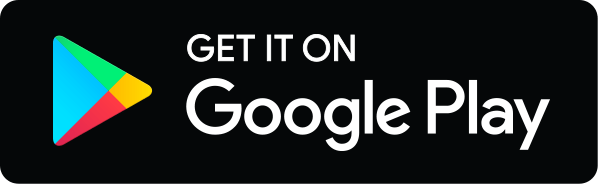