Innate
Macrophage
Reduced phagocytic activity
Reduced generation of nitric oxide
Reduced generation of superoxide
Neutrophil
Reduced phagocytic activity
Reduced generation of superoxide
Dendritic cells
Reduced pinocytosis and endocytosis activity
Reduced phagocytosis of apoptotic cells
Impaired cellular migration
Natural killer cells
Increased total cell number
Reduced cytotoxic ability
Reduced cell proliferation after interleukin-2 exposure
Cytokines
Increased production interleukin-6
Increased production TNF-α
Increased production IL-1β
Adaptive
T cells
Reduced naïve cell population
Increased memory cell population
B cells
Reduced antibody isotype switching
Reduced dendritic cell stimulation
Reduced naïve cell population
Increased memory cell population
Aging of the Innate Immune System
Neutrophils
Neutrophils play a critical role in the acute inflammatory host response. These short-lived phagocytic cells are recruited from the peripheral blood via a gradient of chemokines and cytokines produced locally at the site of infection. Aging per se does not have a known effect on the number of neutrophils in the blood or the number of neutrophil precursors in the bone marrow [2]. Despite their preserved quantity, most other aspects of neutrophil function are diminished such as phagocytosis and the generation of reactive oxygen species [3, 4]. There is conflicting data on the effect of aging on chemotaxis with some studies showing no effect [5, 6] and others demonstrating decreased chemotaxis [7–10].
Macrophages
Macrophages have many integral functions in the innate immune system. They function as sentinels for microbes in tissue; through the release of effector molecules, they orchestrate the adaptive immune response and play an essential role in wound healing. Macrophages function as first responders to invading microbes. They reside in numerous tissues, such as Kupffer cells in the liver, microglia in the brain, osteoclasts in the bone, and as undifferentiated monocytes in the blood. They detect pathogens by recognizing specific pathogen-associated molecular patterns (PAMPs) present on the microbes. They phagocytose invading bacteria, fungi, parasites, protozoa, and apoptotic cells and destroy them via both oxygen-dependent and oxygen-independent pathways [11].
Although the number of circulating blood monocytes in elderly and young subjects is similar, there is a significant decrease in macrophage precursors and macrophages in the bone marrow [12]. Macrophages in the elderly have reduced levels of MHC class II, which may contribute to poorer T-cell responses [13]. The macrophage’s phagocytic function and its chemotactic ability are also diminished with age [14, 15]. Additionally, the ability of aged macrophages to destroy microbes via products of the respiratory burst is diminished; this impaired bactericidal capacity may increase the duration of infection in the elderly [16].
Natural Killer Cells
Natural killer (NK) cells are responsible for destroying host cells that have been compromised by tumor or viral infection. The number of NK cells increases with age; however, cytolytic activity and production of interferon-γ are decreased [17]. The cytotoxicity of NK cells is facilitated by releasing perforin and granzymes which activate caspases that induce apoptosis. The loss of cytotoxic ability is thought to occur as the result of decreased perforin secretion and production [18, 19]. However, antibody-dependent cell cytotoxicity (ADCC) by NK cells appears to be preserved [20]. Clinically, these changes in the NK cell often result in a net increased risk of infection, morbidity, and mortality in elderly patients [4, 21].
Dendritic Cells
Dendritic cells serve as a bridge between the innate and adaptive immune systems. Acting as antigen-presenting cells, they capture microbes through phagocytosis, process extracellular and intracellular antigens, and migrate to lymphoid tissue to stimulate T cells. Dendritic cells also have a regulatory function as demonstrated by their production of type I interferons in response to viral infection and the TNF-α inducible nitric oxide synthase (iNOS) production to defend against bacterial infection [22, 23]. In addition to eliciting immune response, dendritic cells also provoke immunological tolerance by inducing deletion or anergy, thereby limiting autoimmunity [24, 25].
Aging dendritic cells generated from peripheral blood monocytes have been shown to be deficient in pinocytosis and endocytosis when presented with an antigen challenge [26]. Additionally, dendritic cells from aged patients display impaired migration and have an impaired capacity to phagocytose apoptotic cells [27]. Phagocytosis of apoptotic cells produces an anti-inflammatory effect by inhibiting pro-inflammatory cytokines [28]. Clinically, the impaired uptake and inefficient removal of apoptotic cells by dendritic cells from aged patients may result in the inflammation and autoimmunity commonly seen with aging [14, 27].
Aging of the Adaptive Immune System
T Lymphocytes
T lymphocytes, also known as T cells, play a central role in cell-mediated immunity. These cells recognize and eliminate cells that have undergone viral or malignant transformation. T cells are differentiated from B cells and natural killer cells by their expression of a T-cell receptor (TCR) on their surface membrane; this receptor binds to antigen and CD3. Progenitor cells from the bone marrow migrate to the thymus where they undergo a highly selective elimination process based on the ability of the cell’s TCR to recognize major histocompatibility proteins, degree of affinity for normal self-antigens, and the magnitude and duration of TCR signaling [29]. There are primarily two types of naïve T cells that leave the thymus: CD4+ helper cells and CD8+ cytotoxic T cells.
T cell responses are initiated in secondary lymphoid tissues by exposure to dendritic cells which present antigen. T cells that possess the specific antigen are then induced to proliferate and differentiate into effector cells that reenter the circulation from the lymph system and disseminate to the site of infection. After the infection is eradicated, the vast majority of the effector cells are destroyed with only a few cells remaining as long-lived memory cells [30].
T-cell function is significantly altered in the aging process through multiple factors including thymic involution and shifts in T-cell subpopulation types [31]. Thymus involution reaches its maximal level at age 50, resulting in the replacement of the lymphoid component and epithelial matrix of the thymus with fibrous and adipose tissue [32]. The net result of these changes is that generation of naïve T cells is severely compromised beginning at the age of 40 years [33–35]. Additionally, there is also a loss of diversity as a substantial shift from naïve T cell to memory T cell occurs, especially after age 65 for CD8+ T cells. Naïve CD4+ T-cell numbers are well maintained until age 70 after which their numbers begin to contract [36]. Elderly patients tend to rely on memory T cells for their primary T-cell response, which may result in compromised immune response following vaccination [37, 38].
B Lymphocytes
B lymphocytes, or B cells, mature in the bone marrow and function in the humoral immune response. During B-cell development, genetic rearrangement of immunoglobulin light and heavy chains occurs to produce the antigen-binding region of the B-cell receptor (membrane-bound immunoglobulin). B cells that react with self-antigen are removed by a process of apoptosis or inactivation in the bone marrow [39]. At this point the B cell enters the peripheral blood and lymph circulation as mature naïve B cells, where antigen activation occurs. Further differentiation is dependent on activation by antigen and signaling from helper T cells [40]. Mature naïve B cells can become either plasma cells, which produce and secrete large quantities of antibodies or memory B cells, which are long-lived cells capable of responding to reactivation by the same antigen.
While the number of precursor B cells and peripheral B cells do not decline with aging, there is a shift toward more antigen-experienced B cells and fewer naïve B cells [41, 42]. There is also a diminished ability for antibody isotype switching resulting in a shift in antibody isotype from IgG to IgM [43]. Isotype switching maintains the same antigen specificity but changes the effector functions of the antibody. Additionally, B cells in elderly patients are less efficiently stimulated by dendritic cells than younger patients due to their relative deficiency in the expression of co-stimulatory molecules CD40 and CD27 [44, 45]. The net effect of these changes is that elderly patients are forced to rely on a B-cell repertoire which lacks optimal diversity and have a low affinity to antigens and are therefore less protective [46].
Inflammaging: Age-Related Subclinical Chronic Inflammation
The aging immune system is characterized by a low-grade, chronic systemic inflammatory state sometimes referred to as “inflammaging” [47]. With aging, there is an increased production of pro-inflammatory cytokines such as interleukin 6 (IL-6), tumor necrosis factor-alpha (TNF-α), and IL-1β [48–51]. This subclinical inflammation may be caused by chronic antigenic stimulation, oxidative stress, and other age-related changes [54]. Additionally, the accumulation of senescent cells exhibiting the “senescence-associated secretory phenotype” (SASP) may contribute to this state by secreting pro-inflammatory cytokines which affect other immune cells and cause damage to the surrounding microenvironment [52–54]. The complex mechanisms behind inflammaging are not fully understood, but the resulting increase in pro-inflammatory cytokines is associated with significant morbidity and mortality in aging adults. Increased IL-6 levels are associated with lower muscle mass and strength in healthy elderly adults [55, 56]. Elevated levels of IL-6 and TNF-α have been associated with increased disability in older adults and have been identified as predictors of 10-year all-cause mortality in this population [55, 57, 58]. Inflammaging has also been implicated in the pathogenesis of various age-related disease, such as Alzheimer’s, osteoporosis, atherosclerosis, macular degeneration, and degenerative arthritis [59–62].
Risk Factors for Infection
Chronic Obstructive Pulmonary Disorder (COPD)
COPD is the 3rd leading cause of death for all patients 65 and older [63]. The causative agents associated with COPD are cigarette smoking, biomass exposure, and the resulting inflammatory response orchestrated by neutrophils, macrophages, and CD8+ T cells [64]. COPD has been shown to be an independent risk factor for developing infections after traumatic injury or thoracic surgery [65–67]. Mucosal lesions of the tracheobronchial tree in the presence of mucous hypersecretion promote bacterial adhesion, colonization, and growth that then impede mucociliary clearance. These changes in the histology of the airway in patients with COPD increase the risk for pneumonia [68, 69]. The majority of cases of community-acquired pneumonia (CAP) in COPD patients are caused by Streptococcus pneumoniae, followed by Chlamydia pneumoniae, Haemophilus influenzae, Legionella pneumophila, Streptococcus viridans, Coxiella burnetii, and Mycoplasma pneumoniae [70]. COPD patients hospitalized with CAP have higher 30-day and 90-day mortality rates compared to patients without COPD [71].
Diabetes Mellitus
Increasing age and diabetes work in concert to further weaken an elderly patient’s response to infections. Diabetes is known to increase the risk of surgical site infection (SSI) and nosocomial infections [72–77]. These infectious complications occur in 20–23 % of all patients presenting with postoperative sepsis [78, 79]. Large population studies have concluded that diabetes mellitus increases the risk of cystitis, pneumonia, cellulitis, and tuberculosis [73, 80, 81]. Recent studies have described an association between perioperative and postoperative hyperglycemia with increased risk for a SSI [82–85]. Controlling hyperglycemia has been shown to reduce perioperative nosocomial and wound infections in diabetic patients [85–87].
The increased risk of infection in diabetics is the result of deficiencies in neutrophil and humoral function [88]. Neutrophil functions such as adhesion, chemotaxis, intracellular bactericidal activity, and phagocytosis are impaired [88–92]. Total IgG levels are lower in both uncontrolled diabetic patients and insulin-treated diabetics but not those on oral medications [93, 94]. Furthermore, diabetic patients are less likely to develop a protective antibody response following hepatitis B vaccination [95–97]. Revaccination with 1–3 additional doses of hepatitis B vaccine can safely increase the proportion of adults that achieve protective antibody levels [98]. The duration of protection against symptomatic and chronic hepatitis B virus infection has been shown to last for more than 22 years in healthy vaccine responders [99]; however the duration of immunity among persons with diabetes is unknown. Data on vaccine response to influenza is less clear. Diabetic patients showed fewer activated lymphocytes but no reduction in antibody response following influenza vaccination [100, 101]. Although age has been associated with diminished immune response to influenza vaccination, elderly type 2 diabetics appear to have preserved B-cell responses similar to young healthy controls [102, 103].
Chronic Kidney Disease and End-Stage Renal Disease
It is estimated that approximately 40 % of adults over the age of 60 meet the current definition for chronic kidney disease (CKD) [104]. Overall mortality rates are declining for patients with end-stage renal disease; however infection remains a common cause of death, with rates peaking 2–3 months after starting dialysis [105]. Rates of hospitalization for infection are higher for patients with chronic kidney disease for every major organ system than for patients without kidney disease [106, 107]. Chronic dialysis patients often fail to respond to standard vaccination protocols and may require augmented regimens to achieve a protective effect [108–110]. Despite being a high-risk group for infection, vaccination rates for influenza and pneumococcal pneumonia in end-stage renal patients are far lower than recommended [111, 112].
End-stage renal disease and its precursor chronic kidney disease are associated with marked systemic inflammation and diminished immune response [113, 114]. Cytokine dysregulation results from kidney dysfunction, as the kidney is the main route for elimination of cytokines [115, 116]. Uremia causes deficiencies of both the innate and adaptive immune systems [114, 117, 118]. Uremic patients have increased T-cell turnover and apoptosis which leads to a depletion of naïve and memory CD4+ and CD8+ T cells [119–121]. Reduced B-cell proliferation and antibody production are seen in uremic patients [122–124]. Uremia decreases the function of antigen-presenting dendritic cells [125, 126]. Phagocytic function in macrophages and neutrophils are also diminished [127, 128]. These alterations in immune function are further exacerbated by aging.
Traumatic Injury
Infections contribute to significant morbidity and mortality in the geriatric trauma population. The incidence of nosocomial infections in elderly admitted trauma patients has been reported to be 39 %, which was twice as high as younger patients [129]. The most common of these being respiratory and urinary tract infections. The development of a nosocomial infection in elderly trauma patients was associated with 3.6 times greater risk of mortality [65].
Recent research has been undertaken to try to elucidate the immunological dysfunction specific to the older trauma patient. A study of older patients with hip fractures (age >65) demonstrated acute neutrophil dysfunction and chronically elevated TNFα [130]. Studies in murine models have demonstrated reduced phagocytic neutrophils in the elderly after traumatic injury, supporting the notion that increased infections in geriatric trauma patients may be due to failure of bone marrow progenitors and neutrophils to mount an appropriate response capable of clearing infection [131]. A study of critically ill geriatric patients with blunt injuries found that patients who developed secondary infections had relatively blunted IL-6 and IL-10 levels. This same study found that geriatric patients who died had a more robust immune response with higher IL-6 and IL-10 levels than geriatric survivors, suggesting that inflammation-mediated end-organ failure may contribute to mortality in this population [132]. These findings highlight the complexity of derangements that occur in the aged immune system in response to traumatic injury, rendering them susceptible to infections and increased risk of mortality.
Challenges in Diagnosing Infection in the Elderly
Elderly patients often do not present with pathognomonic signs and symptoms of infection [133, 134]. Cardinal markers of infection such as fever are often absent in older patients. Physiologic changes in the skin cause older patients to conserve less of the body heat they generate. As a result, many noninfected elderly patients fail to achieve a normal body temperature of 37 °C [135]. Nearly a third of patients over the age of 65 with infection have temperatures below the threshold of fever (38.3 °C) and by age 80 approximately 50 % of patients fail to reach this threshold [136–138].
Nonspecific symptoms such as change in mental status, decline in functional status, failure to thrive, loss of appetite, and incontinence can all be presenting signs of infection [139, 140]. Additionally, cognitive impairment can render older adults incapable of communicating their symptoms to providers. These nonspecific findings are also commonly seen in noninfectious diseases, making the diagnosis of infection in this population challenging. This is particularly true of residents of long-term care facilities, who are at a unique risk of infection. The Infectious Disease Society of America recommends suspecting infection in elderly long-term care residents when there is a decline in functional status and fever. They defined fever as either a single oral temperature over 37.8 °C, repeated oral temperatures over 37.2 °C (or rectal temperatures over 37.5 °C), or an increase in temperature of 1.1 °C over the baseline temperature [141].
Microbiology of Infection in the Elderly
Infectious diseases in the elderly are caused by a more diverse group of pathogens than in younger patients [140, 142]. Changes in microbiology may be related to age, comorbid disease, and environmental setting, e.g., community, long-term care, and hospital [69, 135, 143–146]. Long-term care residents are at great risk for infection [141]. There are more than 15,000 long-term nursing facilities serving approximately 1.5 million residents in the United States, of these residents 90 % are older than 65 years [147]. Antibiotic use is common among long-term care residents with durations that can vary dramatically from less than 10 days to greater than 90 days [148]. Low dose and prolonged use of antibiotics select for bacterial resistance [149, 150]. This practice has led to the emergence of highly resistant pathogens among residents at long-term care facilities which impacts both empiric antibiotic selection and infection control during periodic hospitalizations [151, 152].
Common Infections
Urinary Tract Infection
Urinary tract infections (UTI) account for 25 % of community-acquired bacterial infections and 30 % of infections from long-term nursing facilities [153]. Age-related changes contributing to increased risk of UTI include thinning of the mucopolysaccharide layer of the urinary epithelium and reduction in the Tamm-Horsfall protein (THP) in urine which covers type 1 fimbriae on gram-negative bacteria. THP reduces bacterial attachment and deterioration of bladder and urethral function [154, 155]. E. coli is the most commonly isolated organism in urine from patients in both community and long-term care facilities. Gram-negative polymicrobial infections with multidrug-resistant organisms are more frequent in residents of long-term care facilities [156, 157]. Microbiologic differences are seen in diabetic patients where E. coli remains the most common causative agent but to a lesser extent than in nondiabetic patients, and greater proportions of Klebsiella species are reported [146, 158, 159]. Enterococci and Staphylococcus species are the most common gram-positive causative agents.
There is a high prevalence of asymptomatic bacteriuria in residents of long-term nursing facilities ranging from 15 to 30 % in men and 25 to 50 % in females [160]. It is not recommended to initiate antibiotic therapy for asymptomatic bacteriuria, as there is no improvement in survival rate and a tendency toward increased mortality secondary to adverse side effects and super infection with resistant organisms is observed [161, 162].
The treatment of symptomatic UTI should be based on antimicrobial susceptibility testing. The selection of antibiotic agent is similar to that in the younger community-dwelling population taking into consideration the local antimicrobial resistance patterns [163]. In patients with a symptomatic uncomplicated UTI, the current recommendations for antibiotic duration are 3–7 days, whereas, for more complicated UTI, 10–14 days is appropriate [164, 165]. Men with recurrent UTI require work-up for chronic bacterial prostatitis, which could require 6–12 weeks of therapy [166]. For catheter-associated UTI, the Infectious Diseases Society of America suggests 7 days of treatment in patients who have a prompt response and 10–14 days in those who have a delayed response [167].
Respiratory Infections
Influenza and pneumonia ranks as the seventh leading cause of death for patients 65 years and older [63]. In addition to changes in the immune system, age-related changes in oral clearance, mucociliary clearance, respiratory muscle strength, cough reflexes, and lung structure increase the risk for pneumonia in the elderly [134]. A large population study estimated roughly 915,900 cases of community-acquired pneumonia per year in individuals older than 65 years [168]. Several studies examining the etiology of community-acquired pneumonia (CAP) have shown Streptococcus pneumoniae to be the most common pathogen, accounting for 20–60 % of cases followed by Haemophilus influenzae (3–10 % of cases) [169, 170]. Other causative organisms include the atypical pathogens Mycoplasma pneumoniae, Chlamydophila psittaci, and Legionella species [171]. Polymicrobial infection and gram-negative pneumonia tend to occur more commonly in patients with COPD and in residents of long-term care facilities [172, 173].
Older patients with CAP can be treated similarly as the general adult population, but special considerations should be taken for the possibility of healthcare-associated pneumonia (HCAP), defined as pneumonia in a patient with an additional risk factor (Table 7.2) [174, 175]. HCAP, as well as hospital acquired pneumonia, and ventilator-associated pneumonia have been associated with higher rates of multidrug-resistant organisms (MDRO), including methicillin-resistant Staphylococcus aureus and gram-negative bacteria [172]. Interestingly, a recent study at a large US medical center found a low prevalence of MDRO in HCAP at their institution. However, predictors of MDRO included admission from a nursing home, duration of previous hospitalization in the last 90–180 days, P. aeruginosa colonization/infection in the previous year, and antimicrobial use in the last 90 days [176]. Local microbial epidemiology and culture results should be taken into account when choosing appropriate therapy.
Hospitalized in an acute care hospital for 2 or more days within the past 90 days |
Resident of a nursing home or long-term care facility |
Treated with intravenous antibiotic therapy, chemotherapy, or wound care within the past 30 days |
Received hemodialysis at either a hospital or outpatient clinic |
Prevention and Potential Therapies
Not surprisingly, diet and exercise seem to play a key role in counteracting the negative effects of aging on the immune system. Regular exercise has been associated with greater T-cell proliferation, lower number of senescent T-cells and inflammatory cytokines, and improved function in neutrophils and NK-cells [177]. Exercise has also been shown to enhance vaccination responses in the elderly [178, 179]. Various nutrients act to temper epigenetic changes that contribute to inflammaging [180]. The “Mediterranean diet,” consisting mainly of fruits, vegetables, grains, fish, olive oil, and regular red wine intake, with low consumption of saturated fats has been associated with modifying free radical production and expression of inflammatory mediators, along with other pathways implicated in malignancy and inflammatory responses [181]. There are also a multitude of plant-derived compounds exhibiting anti-inflammatory properties [182]. As we come to understand more about the alterations in immune function that occur with aging, further investigations will be needed to develop effective prevention and treatment strategies.
Summary
Age-related changes in immune function, underlying chronic disease, and environmental factors contribute to the increased incidence and severity of infections seen in the elderly population. Atypical clinical presentations can often confound the diagnosis of infection leading to delays in treatment, whereas environmental factors predispose the elderly to infection by multidrug-resistant organisms. The net effect of this increased susceptibility to infection combined with a relative incapacity to mount an effective immune response contributes to the increased morbidity and mortality resulting from infectious complications following trauma or emergency surgery. However, research demonstrates that targeted nutritional therapies and exercise may stall the process of immunologic deterioration in the aging.
References
1.
Strausbaugh LJ. Emerging health care-associated infections in the geriatric population. Emerg Infect Dis [Internet]. 2001;7(2):268–71. Available from: http://www.pubmedcentral.nih.gov/articlerender.fcgi?artid=2631705&tool=pmcentrez&rendertype=abstract.CrossrefPubMedPubMedCentral
2.
Chatta GS, Andrews RG, Rodger E, Schrag M, Hammond WP, Dale DC. Hematopoietic progenitors and aging: alterations in granulocytic precursors and responsiveness to recombinant human G-CSF, GM-CSF, and IL-3. J Gerontol [Internet] ed. 1993;48(5):M207–M212. Available from: http://www.ncbi.nlm.nih.gov/pubmed/7690056.
3.
Fortin CF, McDonald PP, Lesur O, Fulop Jr. T. Aging and neutrophils: there is still much to do. Rejuvenation Res [Internet]. 2008/10/14 ed. 2008;11(5):873–882. Available from: http://www.ncbi.nlm.nih.gov/pubmed/18847379.
4.
Mahbub S, Brubaker AL, Kovacs EJ. Aging of the innate immune system: an update. Curr Immunol Rev [Internet]. 2011/04/05 ed. 2011;7(1):104–115. Available from: http://www.ncbi.nlm.nih.gov/pubmed/21461315.
5.
MacGregor RR, Shalit M. Neutrophil function in healthy elderly subjects. J Gerontol [Internet] 1990/03/01 ed. 1990;45(2):M55–M60. Available from: http://www.ncbi.nlm.nih.gov/pubmed/1968921.
6.
Biasi D, Carletto A, Dell’Agnola C, Caramaschi P, Montesanti F, Zavateri G, et al. Neutrophil migration, oxidative metabolism, and adhesion in elderly and young subjects. Inflammation [Internet]. 1996/12/01 ed. 1996;20(6):673–681. Available from: http://www.ncbi.nlm.nih.gov/pubmed/8979154.
7.
Niwa Y, Kasama T, Miyachi Y, Kanoh T. Neutrophil chemotaxis, phagocytosis and parameters of reactive oxygen species in human aging: cross-sectional and longitudinal studies. Life Sci [Internet] 1989/01/01 ed. 1989;44(22):1655–1664. Available from: http://www.ncbi.nlm.nih.gov/pubmed/2733545.
8.
Antonaci S, Jirillo E, Ventura MT, Garofalo AR, Bonomo L. Non-specific immunity in aging: deficiency of monocyte and polymorphonuclear cell-mediated functions. Mech Ageing Dev [Internet]. 1984/03/01 ed. 1984;24(3):367–375. Available from: http://www.ncbi.nlm.nih.gov/pubmed/6717097.
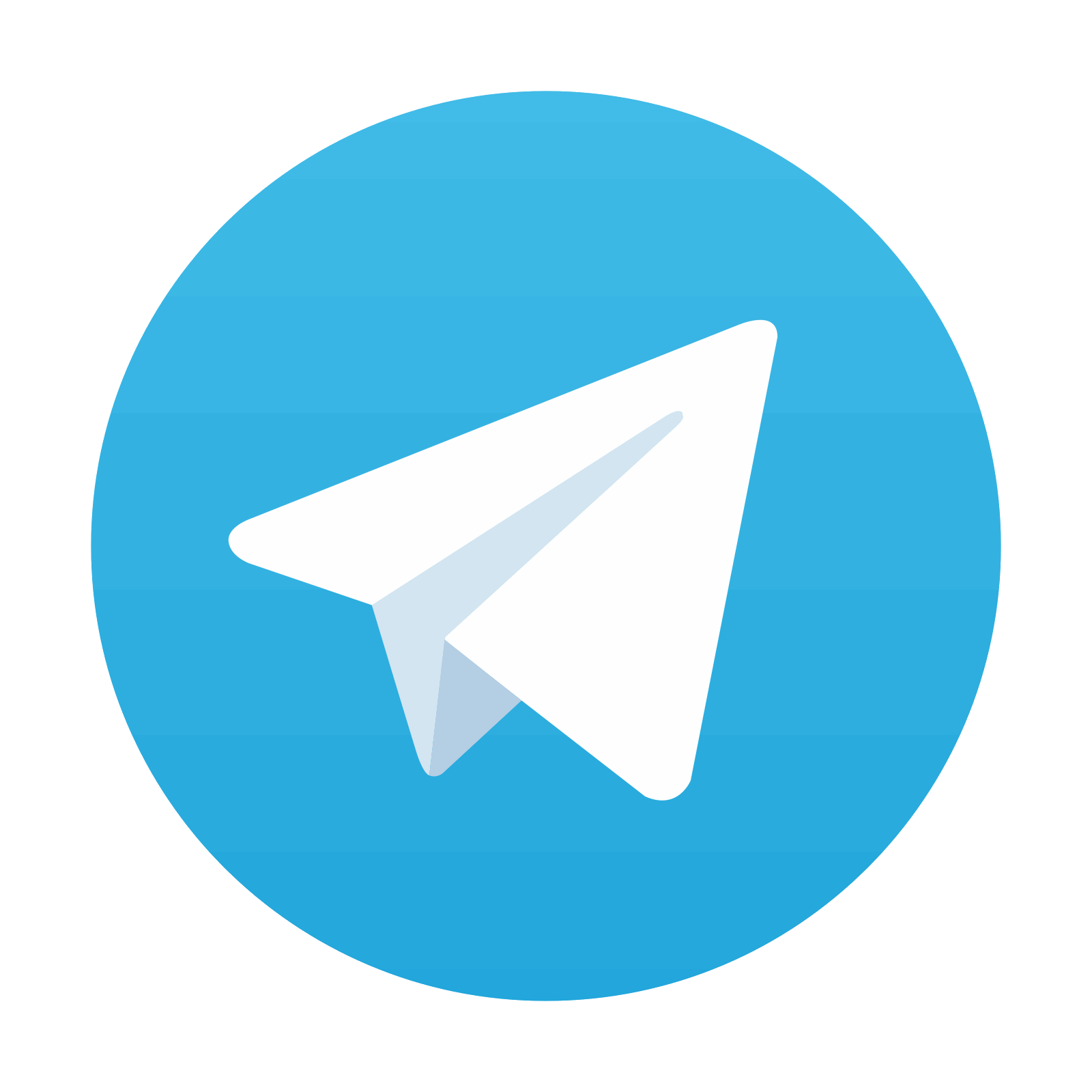
Stay updated, free articles. Join our Telegram channel

Full access? Get Clinical Tree
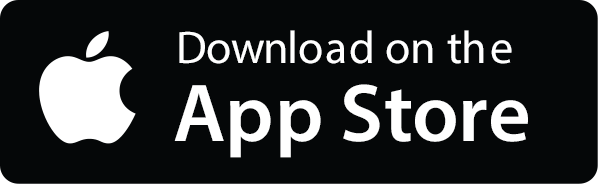
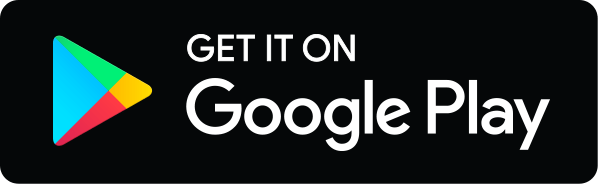