© Springer International Publishing AG 2017
Fred A. Luchette and Jay A. Yelon (eds.)Geriatric Trauma and Critical Carehttps://doi.org/10.1007/978-3-319-48687-1_3535. Cardiovascular/Invasive Monitoring
(1)
Department of Surgery, Division of Surgical/Critical Care, Program in Trauma, R Adams Cowley Shock Trauma Center, University of Maryland School Of Medicine, Baltimore, MD 21201, USA
Keywords
ElderlyCardiovascularAtrial fibrillationMyocardial ischemiaInvasive monitoringContinuous central venous oximetryPulse contour analysisEchocardiographyIntroduction
The elderly population, those over the age of 65 years of age [1–4], represents one of the fastest-growing sections of the US census. In 2010, there were 40.3 million people aged 65 and older, 12 times the number in 1900 [5]. It is estimated that by the year 2050, the population of elderly is expected to double as compared to 2000 and account for almost 21 % of the US population [5]. Additionally, it is predicted that by the year 2030, those over the age of 85, the oldest old, will number more than 9 million [6]. This increase in the number of elderly is multifactorial but is due in large part to longer life expectancy as well as the aging baby boomers.
Physiologic Changes
As people age, their physiologic reserve decreases. This loss of physiologic reserve is a slow process of deterioration often starting in people’s 30s and continues with increasing age. Despite this decrease in reserve, most elderly people can compensate physiologically to meet needs under normal condition. However, when stressed, including acute trauma or illness, the demand is too much, and they are unable to effectively compensate and meet the physiologic demand to ensure adequate perfusion. The rate of physiologic change is variable from organ to organ and individual to individual [7].
Age is a major risk factor for the development of cardiovascular disease (CVD), and as such many elderly patients have some degree of CVD. CVD has been shown to be responsible for more than 40 % of death in patients over the age of 65 years [8]. Age-related changes in the myocardium affect anatomical as well as physiologic and electrophysiologic activity of the heart [9]. The elderly often have decreased myocardial contractility and ventricular compliance for a given preload as a result of loss of myocytes and increased myocardial collagen [10]. Autonomic tissue is replaced with connective tissue and fat, while fibrosis of the myocardium results in conduction abnormalities. The change in the conduction system increases the incidence of dysrhythmias including sick sinus syndrome, bundle branch blocks, and atrial arrhythmias and subsequent syncope in the elderly [11–13].
As one ages, systolic blood pressure increases due to augmented afterload from stiffening of the outflow tract. During times of stress, such as acute illness or injury, this results in decreases in peak ejection fraction and cardiac output [10, 14–[16]. Compounding the situation is that between the ages of 20 and 85 years, it is estimated that maximum heart rate decreases by as much as 30 % [17]. Additionally aged myocardium does not respond as well to increased levels of endogenous and exogenous catecholamine [18]; thus, cardiac output in the elderly must be augmented by increasing ventricular filling and stroke volume [10, 19]. This reliance on adequate volume (preload) makes the elderly very sensitive to even minimal hypovolemia and the resulting cardiac collapse. However, one must be diligent during times of volume resuscitation in the elderly and balance the need for adequate cardiac output with the potential for pulmonary edema due to decreased ventricular compliance.
Atrial Fibrillation
Atrial fibrillation (AF) is the most common arrhythmia [20–24]. Its reported incident varies but is less common after non-cardiothoracic surgery than cardiothoracic surgery [24]. However, the true incidence of AF may be underestimated due to a lack of continuous monitoring outside an intensive care unit [24]. Advanced aged has been shown to be independently associated with higher incidence of AF. AF is associated with the higher daily fluid requirements, the prevalence of severe infections, and the need for vasoactive and inotropic support [23]. It has been shown to be associated with significantly longer intensive care unit (ICU) and hospital length of stay (LOS) as well as increased morbidity and mortality [22–24].
The mechanism for the development of AF can be multifactorial. Underlying myocardial disease in the elderly along with increased systemic inflammation can cause further myocardial dysfunction causing AF [24, 25]. Increased intravascular volume from large quantity resuscitation increases the chance of AF. Alternatively, hypovolemia leading to an increased adrenergic state can increase the likelihood of AF [24, 26]. Regardless of the cause, atrial fibrillation in the geriatric ICU patient appears to be a marker of severity of illness as demonstrated by higher simplified acute physiologic scores II, nursing workload in the ICU (OMEGA), and mortality [23].
There is a wide variation in the treatment of elderly patients with AF [27]. Clinicians should investigate for any treatable causes including thyroid dysfunction, electrolyte abnormalities, or acute cardiac ischemia. Therapy goals include rate control, rhythm control, and prevention of thromboembolism [24].
Elderly patients in the ICU with AF often become hemodynamically unstable as a result of the loss of the atrial contribution to ventricular filling and leading to a decreased cardiac output [27]. Those with hypotension, altered level of consciousness, angina, or heart failure should be immediately cardioverted with synchronized direct current cardioversion [24]. For those with a normal blood pressure and no evidence of hypoperfusion, medical therapy should be initiated.
Over the years, there has been a debate whether it is more important to focus pharmacological intervention on rate control versus rhythm control. Two large studies demonstrated no benefit from rhythm control over rate control [28, 29]. Unfortunately most of the patients in the studies had persistent AF as opposed to postoperative new onset AF; thus, the results may not be extrapolated to this patient population [30]. Thus, many believe that pharmacological interventions should focus on controlling the ventricular rate with atrioventricular nodal blockers [24].
Currently, there are a number of treatment options available. Amiodarone, for many, has become the initial drug of choice for atrial fibrillation. It is a class III antiarrhythmic agent that depresses atrioventricular conduction and controls the ventricular rate. It is safe to use in patients with reduced ejection fraction. Side effects include bradycardia, thyroid dysfunction, as well as drug interaction, specifically warfarin. Intravenous (IV) amiodarone has been associated with acute elevation in liver enzymes; however, levels normalize with discontinuation on the IV form [31]. Additionally, long-term amiodarone use has been associated with the development of pulmonary fibrosis. Dronedarone, similar to amiodarone, has been studied for rate and rhythm control in AF. It reduces morbidity and mortality in inpatients with high-risk AF; however it is contraindicated in patients with acute severe heart failure [32].
Other pharmacological options include β-blockers and calcium channel blockers (CCB). β-blockers may be more appropriate for postoperative hyperadrenergic states, while CCB, specifically diltiazem, may be better suited for patients with severe asthma or chronic obstructive pulmonary disease. Side effects of CCB include hypotension, heart failure, and heart block. Both should be used with caution in patients with systolic heart failure [24]. Digoxin, one of the oldest antiarrhythmic agents for AF, is still used by many clinicians. Digoxin works by slowing down conduction at the atrioventricular node by parasympathetic activation. It may be the ideal pharmacological choice if patients have simultaneous heart failure or left ventricular dysfunction. Digoxin may be synergistic with β-blockers or CCB. However, it is less effective in situations of high sympathetic tone (postoperative period) [24]. Additionally, digoxin has many drug-drug interactions which often limit its use in critically ill patients. Furthermore, digoxin is renally metabolized, and drug levels need to be closely monitored and adjusted accordingly.
Duration of antiarrhythmic therapy remains unknown. There is no specific data that guides the duration of therapy. Some authors suggest 1–2 weeks of therapy if the AF was transient [24]. Longer therapy may be necessary for patients with recurrent or extended episodes of AF [24]. Some patients do not respond to pharmacological interventions and remain in AF. Patients who remain in AF for more than 48 h have an increased risk of atrial clot formation, and it is reasonable to consider anticoagulation therapy [24]. The CHADS2 score or the newer developed CHA2DS2-Vasc score may be used to determine which patients have an indication for anticoagulation [33]. However, clinicians need to take into account the risks of systemic anticoagulation in the critically ill geriatric patient including falls risks and drug interactions. Additionally, bleeding risks can be calculated using the HAS-BLED score [34]. Alternatively, additional medical attempts at rate control and rhythm alone may be safer in this patient population.
Myocardial Ischemia/Infarction
Age and preexisting cardiovascular disease predispose the elderly to cardiac complications when critically ill. Cardiac complications have been reported to range from 12 to 16.7 % in this patient population especially for those over the age of 80 years [35–37]. Cardiac complications are one of the highest causes of mortality in the elderly surgical patient, and for those over the age of 80 years, myocardial infarction (MI) has been shown to be the leading cause of postoperative death [38]. The prevalence of postoperative MI in the elderly ranges from 0.1 to 4 % [39–41], with most occurring within 72 h following surgery [39].
β-blockers have traditionally been considered a mainstay of treatment to help prevent perioperative cardiac complications in the elderly. They are believed to decrease the shear force across atherosclerotic plaques that when ruptured can be the culprit of up to 50 % of perioperative MI [42]. Additionally β-blockers assist in minimizing ventricular dysrhythmias, improve myocardial oxygen balance, and decrease sympathetic tone leading to fewer perioperative cardiac complications. Initially a number of studies and reviews were published supporting the use of β-blockade to prevent postoperative complications [43–47]. In 2002 Auerbach and Goldman reviewed the efficacy of perioperative β-blockade in reducing myocardial ischemia, infraction, and cardiac-related mortality [47]. The authors concluded a benefit from β-blockade in preventing perioperative cardiac morbidity. These benefits were similar to those found in patients with myocardial infarction, and thus the use of perioperative blockade was quickly adopted due to the potential benefit with few adverse side effects demonstrated [43, 48].
Subsequent studies have demonstrated no differences in cardiac complications or mortality between patients treated with β-blockers and those not treated [49, 50]. While others have demonstrated potential harm and increased surgical mortality [43, 51, 52]. In 2008 the POISE study group evaluated the use of perioperative β-blockade and concluded that although the treatment group has significantly lower rates of MI, there was a significantly higher death rate and stroke rate in patients receiving β-blockers [53].
Despite the vast literature on the use of β-blockers, there is little consensus regarding the appropriate patient population that would benefit from their use. Some authors believe patients having one or two risk factors (high-risk surgery, known ischemic heart disease, history of congestive heart failure or cerebrovascular disease, baseline creatinine >2 mg/dl) would benefit, while others believe patients with more than three risk factors are more appropriate [42, 52, 54]. The 2014 ACC/AHA guidelines state that patients having surgery who are on chronic β-blockers should continue therapy [43]. The remaining recommendations are based on less convincing data and should be individualized to the patient.
Hemodynamic Monitoring
During times of acute illness or trauma, many elderly patients cannot augment their cardiac output and rather increase their systemic vascular resistance [55]. As a result, elderly patients may demonstrate a normal blood pressure while having severely depressed and compromised cardiac function leading to overall poor systemic perfusion. In a landmark study of geriatric trauma patients, Scalea and colleagues demonstrated as many as 50 % geriatric trauma patients who appeared clinically stable with “normal” blood pressure had unrecognized cardiogenic shock and a poor outcome [56]. Using a pulmonary artery catheter, resuscitation was optimized using volume, inotropes, and afterload reduction, and survival increased from 7 to 53 %. The authors determined that identifying occult shock early in the geriatric population with the use of invasive monitoring improves survival.
Despite years of attempting to identify the ideal method for assessing a critically ill patient’s intravascular volume, clinicians still struggle [57]. Physical exam has not been shown to be helpful in accurately determining intravascular volume; thus, continued efforts to identify the best method continue [57, 58]. Unfortunately, there is little to no data specifically looking at invasive monitoring in the elderly, and as such, data must be extrapolated from existing literature based on various patient populations.
Long considered the gold standard of hemodynamic monitoring, the pulmonary artery catheter (PAC) was first described and used in 1970 by Swan et al. [59]. However, the literature on its utility is variable with some showing no effect [60, 61], some with decreased mortality [56, 62, 63], and some showing increased morbidity, mortality, and utilization of resources [64, 65]. It has been suggested that the reason some studies do not show a benefit or even a detriment with the use of the PAC is due to incorrect interpretation and subsequent clinical decision making based on the information provided [66–68]. Over time, newer and less invasive modalities have been developed, but still there is no consensus on the ideal method.
Continuous Central Venous Oximetry
Continuous central venous oximetry (ScvO2) has been used as an alternative to the PAC (SvO2). Using a modified central venous catheter with fiber-optic technology, clinicians are able to continuously monitor venous oxygenation in the superior vena cava. ScvO2 is generally higher than SvO2 in critically ill patients with circulatory failure [69]. The literature varies on the correlation between ScvO2 and SvO2 [69–71]. Some authors advocate the use of ScvO2 which can help identify global tissue hypoxia allowing earlier intervention in the clinical course and affect outcome [72, 73]. Additionally, it has potential for time and cost savings as well as fewer risks than the PAC.
Pulse Contour Analysis
Other alternatives to the PAC include pulse contour analysis. The idea is based on the Windkessel model first described by Otto Frank in 1899 [74]. It is centered on the principle that stroke volume can be continuously estimated, on a beat-to-beat basis, using arterial waveform from an arterial line. Calculations using the area under the curve of the systolic arterial pressure waveform helped develop an algorithm for monitoring stroke volume [75]. Limitations of pulse contour technology include its accuracy in patients with irregular cardiac rhythms, right heart failure, spontaneous breathing, and mechanical ventilation using low tidal volumes (<8 mL/kg body weight) [76].
Examples of pulse contour technology include the FloTrac™ (Edwards Lifesciences, Irvine, CA). It provides continuous cardiac output, stroke volumes, and stroke volume variation. The device does not require a central venous access, only an arterial catheter. The technology concept is based on arterial waveform analysis and the principle that pulse pressure is proportional to stroke volume [77], thus deriving a cardiac output on a beat-to-beat basis. Studies by Cannesson et al. and Button et al. showed clinically acceptable agreement between the PAC and FloTrac™ [78, 79]. Others, however, have had less favorable results [57, 80, 81].
The PiCCO™ (Pulsion Medical Systems, Munich, Germany) is another pulse contour analysis device. Unlike the FloTrac™, it requires both an arterial line and a central venous catheter. The arterial line must be placed in either the femoral or axillary artery, which may limit its use. The PiCCO™ system can provide clinicians with similar data to that of the FloTrac™; however, it also has the ability to measure a number of volumes including intrathoracic blood volume (ITBV), global end-diastolic volume (GEDV), and extravascular lung water (EVLW). These volumes have been shown to better measure cardiac preload than traditional values including central venous pressure (CVP) and pulmonary capillary wedge pressure (PCWP) [82, 83]. Additionally, ITBV and GEDV are not affected by mechanical ventilation. Unfortunately, unlike the FloTrac™ which does not need any calibration, the PiCCO™ system requires calibration on a regular basis depending on the hemodynamics of the patient. A study by Della Rocca and colleagues compared the PAC and the PiCCO™ and found that the two methods yielded similar measurements [84]. A study by Uchino et al. looked at outcomes when using a PiCCO™ versus using a PAC. The authors concluded that the choice of monitoring did not influence major outcomes [85].
Noninvasive Methods
A number of noninvasive techniques have been used to measure cardiac output and other hemodynamic parameters. These techniques include partial gas rebreathing (NICO system), thoracic bioimpedance, impedance plethysmography, and portable Doppler devices. Currently these techniques are not widely available or used. Additionally they have not been validated versus other more invasive techniques [86].
Despite much effort, an accurate and reliable marker to guide fluid management has yet to be determined. Traditional measurements of preload including CVP and PCWP have not been shown to reliably predict cardiac preload and the need for volume to optimize cardiac function [87]. A study by Boulain and colleagues demonstrated that passive leg raises (PLR) change pulse pressure and can predict the need for fluid administration in mechanically ventilated patients [77]. As stated previously, pulse pressure is proportional to stroke volume thus allowing pulse contour technology to calculate a stroke volume variation (SVV). SVV has been used to try to answer the never-ending, possible unanswerable critical care question of “is a patient wet or dry?” Some believe it to be an accurate marker of fluid responsiveness in patients and may be the simplest way to predict fluid responsiveness [88]. In general, a lower SVV implies adequate fluid balance, while elevated measurements of SVV imply the need for ongoing volume resuscitation. Both the FloTrac™ and PiCCO™ systems can calculate SVV. Studies have demonstrated that the PiCCO™ system can predict fluid responsiveness in a variety of clinical situations [89, 90]. Studies regarding the FloTrac™ system ability to predict volume responsiveness are more varied [78, 79, 91]. When compared head to head, the FloTrac™ and PiCCO™ system both predict fluid responsiveness in a similar manner [92].
What is the exact threshold of SVV for determining fluid responsiveness? Unfortunately, the literature has yet to answer this question [92]. A report by Berkenstadt and colleagues determined that an SVV value of 9.5 % or more will predict an increase in stroke volume [93]. Suehiro and Okutani determined an SVV cutoff value of 10.5 % to predict fluid responsiveness [94]. When comparing values derived from the FloTrac™ to those of the PiCCO™, Hofer and colleagues found that although both equally predicted fluid responsiveness, the FloTrac™ had a lower threshold of 9.6 % versus 12.5 % for the PiCCO™ [92]. In general, normal SVV values are thought to be 10–15 %.
Echocardiography
The role of ultrasound and echocardiography continues to grow and expand in the critical care setting. Although echocardiography cannot provide continuous monitoring, it is the best method to repeatedly assess cardiac function [95]. Information including fluid status and inferior vena cava diameter and collapsibility can rapidly be attained at bedside providing reliable monitoring of intravascular volume in the mechanically ventilated patient [96]. Echocardiography allows clinicians to choose a therapeutic intervention and rapidly asses the hemodynamic and functional response to the therapy [95]. Additionally echocardiography can provide a number of dynamic responses to preload augmentation. The gold standard to evaluate cardiac function is transesophageal echocardiography (TEE); however, it is invasive and requires specialized training to perform. Transthoracic echocardiography (TTE) however is less invasive, requires less specialized training, and can effectively assess cardiac function. Handheld echocardiography (HHE) using a smaller device has been shown to be as accurate and clinically helpful as TTE [97].
The first focused echocardiography ultrasound protocol for non-cardiologists was the “focused assessment with transthoracic echocardiography” (FATE) [98]. The authors demonstrated the ability to describe the hemodynamics of patients as well as the ability to optimize care. Since then a number of similar protocols have been created. In 2008, Gunst et al. used the “bedside echocardiographic assessment in trauma” (BEAT) to compare function and volume status in critically ill patients with a PAC [99]. The authors demonstrated a significant correlation with cardiac index and CVP between the two modalities. However, as stated previously, CVP has been suggested to be a poor measurement of volume status. In 2011, the “focused rapid echocardiographic examination” (FREE) was first described by Ferrada and colleagues [100]. It was designed to be performed by both surgeons and intensivists. It is a “transthoracic examination, which incorporates hemodynamic information from the echo with the patient’s clinical scenario to generate broad treatment recommendations about fluid, inotropic agents and vasopressors” [100]. When compared to PAC, the FREE was found to have similar results in the measurement of cardiac index; however, when compared to the FloTrac™, there was less agreement, especially in patients with low (<40 %) ejection fractions [101].
Conclusion
The population of those over the age of 65 years continues to increase. As people age their physiologic reserve decreases, and when stressed, including acute trauma or illness, they are unable to effectively compensate and meet the physiologic demand to ensure adequate perfusion. Underlying cardiac dysfunction becomes more pronounced, and complications including cardiac dysrhythmia, myocardial ischemia, and infarction occur. Early aggressive hemodynamic monitoring has been shown to improve survival in the elderly patient population; however, the optimal method by which to do this has yet to be definitively determined.
References
1.
2.
Champion HR, Copes WS, Buyer D. Major trauma in geriatric patients. Am J Public Health. 1989;79:1278–82.CrossrefPubMedPubMedCentral
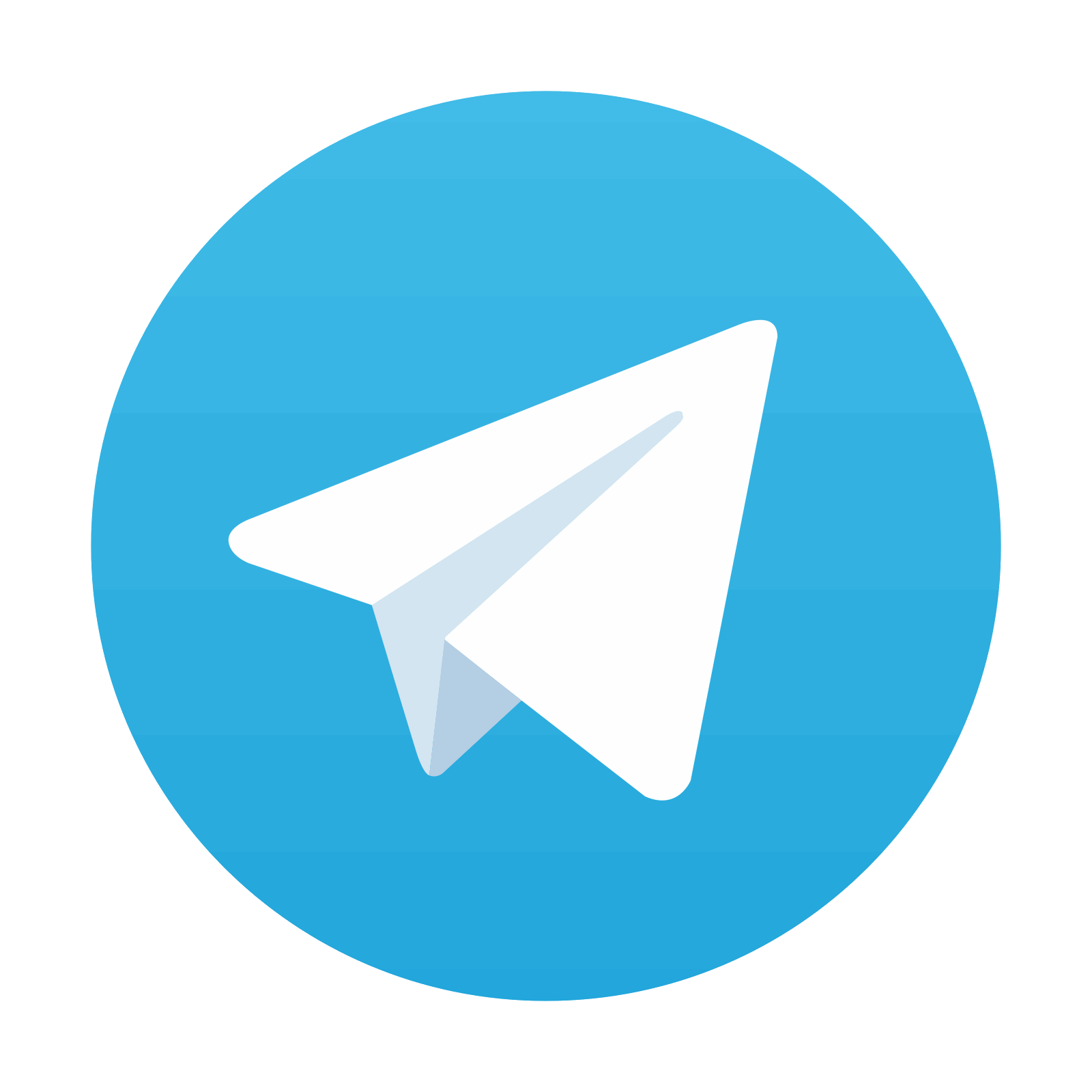
Stay updated, free articles. Join our Telegram channel

Full access? Get Clinical Tree
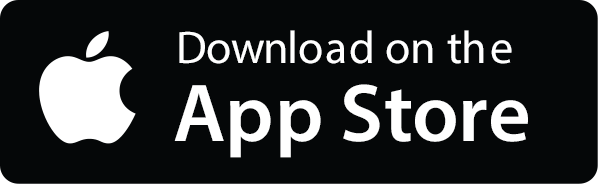
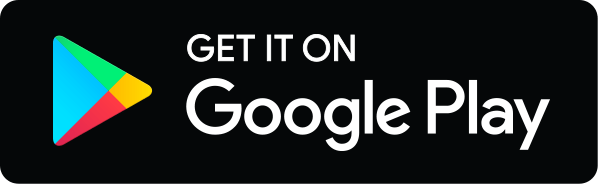
