Overview
Under normal physiologic conditions, the upper airway adds heat and moisture to inspired air to prevent drying of lower airway secretions, plugging, and mucosal injury. When dry medical gases bypass the upper airway via an endotracheal tube, the normal heat and moisture exchange function of the upper airway is compromised. This function of the upper airway may be replaced in two ways. First, heat and humidity can be actively added with a heated humidifier; second, and more commonly, heat and moisture may be passively retained using a heat and moisture exchanger (HME).
The upper airway also fulfills the function of filtration and expulsion of particles, including bacteria and viruses, from inspired gas. This is achieved by the mucociliary elevator. The anesthesiologist is responsible for minimizing the risk of infection from inhaled microorganisms. The use of HME filters (HMEFs) protects patients from inhaled microorganisms and also protects the anesthesia circuit from the patient, thereby allowing its reuse.
Physics of Humidity
Water vapor is the gas phase of water. The transition from the liquid to the gas phase is called vaporization, a process that requires energy. The energy required for this process is the latent heat of vaporization, which is 2.27 MJ/kg water.
Humidity describes the amount of water vapor in air. Absolute humidity is the partial pressure of water vapor above its liquid at equilibrium. As such it may be measured in kilopascals (kPa) or other unit of pressure, but in this context it is more commonly referred to as the mass of water in a given volume of air (in milligrams per liter). Evaporation occurs at a constant temperature until a critical mass of water in the air is reached and the air is fully saturated. The pressure of the water vapor at this point is the saturated vapor pressure (SVP) for water, which increases rapidly with increases in temperature ( Fig. 7-1 ).

At a given temperature, relative humidity is the relationship of the absolute humidity to SVP, expressed as a percentage. Air at its SVP has 100% relative humidity. Relative humidity equals the mass of water vapor for a given volume of air for a given temperature, and SVP is the mass of water vapor required to fully saturate that volume. For example, fully saturated air at 20° C contains 17 mg/L water and at 37° C contains 44 mg/L water.
As air is heated, its SVP increases. If no further water mass is added, absolute humidity remains the same while relative humidity decreases. For example, air in a typical operating room (OR) at 20° C contains 10 mg/L of water and has approximately 60% relative humidity. If fully saturated air at 20° C is heated to 37° C without adding further water mass, it is said to have 38.6% relative humidity (17 mg/L ÷ 44 mg/L). If fully saturated air is cooled, it will reach its dew point, at which air can no longer support the mass of water vapor, and water undergoes condensation.
Measurement of Humidity
Instruments that measure humidity are referred to as hygrometers. They typically measure relative humidity. If temperature and SVP are known, absolute humidity can be calculated.
Hair Hygrometer
The hair hygrometer makes use of a hair, or a synthetic membrane with similar properties, that lengthens as relative humidity increases. As the hair lengthens, it moves a pointer to create an analog display of relative humidity. If the temperature is known, absolute humidity may be calculated. Hair hygrometers typically are used in operating theatres and neonatal incubators. Hair hygrometers are most accurate at a relative humidity of 30% to 90%; they have a slow response and are of limited use where humidity changes rapidly.
Wet and Dry Bulb Hygrometer
The wet and dry bulb hygrometer consists of two mercury thermometers: one reads a true ambient temperature, and the other has a water-soaked wick around the bulb that, when exposed to a moving stream of air, produces a cooling effect from the loss of the latent heat of vaporization. With dry ambient air, evaporation is increased, resulting in a greater apparent temperature drop. The difference in the temperature measured between the thermometers (ΔT) is related to the rate of evaporation, which depends on ambient humidity. Relative humidity can be calculated from a table.
Regnault’s Hygrometer
Regnault’s hygrometer is also known as a dew point hygrometer. The original design consisted of a silver tube that contained ether. As air bubbles through, it cools the ether and causes condensation on the silvered exterior of the tube. The formation of condensation indicates the dew point and the SVP at that temperature. The ratio of SVP at dew point to SVP at ambient temperature gives the relative humidity, which may be derived from a chart.
Electric Hygrometer
Electric hygrometers may use an actively cooled, polished metal mirror. Condensation will form on it when ambient gas meets its dew point, indicating SVP. This point can be detected as a result of the change in its reflective properties or a change in another characteristic, such as its electrical conductivity. Other electric hygrometers use substances that change in resistance or capacitance with water vapor pressure. They typically are heated to a set temperature above dew point to avoid temperature dependency. Their small size potentially allows inclusion within the breathing circuit.
Physiology of Humidification
The upper airway extends from the external nares to the major bronchi. The function of this organ system is to condition inspired gas so that it is warm, humidified, and relatively free of foreign material when it reaches the alveoli. It also has expiratory functions of conservation of heat and humidity, and it facilitates clearance of particulate matter.
Humidification in the Airway
Turbulent flow of inspired gas around the turbinates results in maximal contact of inspired gas with a large mucosal surface area. Particles of all sizes become trapped in the mucous layer; smaller particles may make contact with the mucous layer because they occupy a relatively large apparent space, due to Brownian motion. The large mucous surface area allows efficient transfer of heat and moisture. The majority of heating and humidification has occurred by mid trachea, to approximately 34° C with an absolute humidity of 34 to 38 mg/L (95% to 100% relative humidity). As gas travels distally in the airway and is heated to body temperature, it is further humidified to an absolute humidity of 44 mg/L (100% relative humidity). Transfer of water occurs by evaporation, which is the major energy-requiring process. A lesser amount of energy is required to warm inspired gas by conduction and convection. During the process of heat and moisture exchange, the temperature of the nasal mucosa is reduced to 31° C; consequently, gas cools and loses its capacity to hold water vapor during expiration. Condensation occurs and releases latent heat of vaporization, rehydrating and rewarming the mucosa. Expired air is typically around 32° C to 34° C and 100% relative humidity (38.2 to 42.7 mg/L H 2 O). The upper airway effectively forms a countercurrent HME, retaining much of the heat and moisture it imparts to inspired gas to condition it for the alveoli. Under typical physiologic conditions in an adult, the daily net loss from this system is approximately 250 mL of water and 250 kcal.
The point in the airway at which inspired gas reaches 37° C and 100% relative humidity is known as the isothermic saturation boundary (ISB). This usually lies just below the carina but may vary according to the volume, humidity, and temperature of inspired gas. When the upper airway is bypassed by an endotracheal tube, this boundary is shifted distally. Even under this nonphysiologic condition, the ISB is still achieved before the respiratory bronchioles; this retains a stable humidity in the functional residual capacity.
In cold ambient temperatures, cool inspired air has little capacity to hold water vapor and has a low absolute humidity. The upper airway is required to transfer large amounts of heat and moisture. However, the ISB remains at approximately the same position as the gradients of heat and moisture exchange increase down the airway. In warm ambient temperatures, little heat energy is expired to warm inspired gases. Heat and moisture loss to inspired gas is an important part of body thermoregulation. This potential source of heat loss may be effectively lost in hot, humid conditions and to an extent by mouth breathing. Increased minute volume during exercise may result in high heat and water losses, especially in cold, dry environments. Cool inspired gas may trigger bronchospasm, especially in those with asthma, although the mechanism for this is still poorly understood.
Mucociliary Elevator
The nasopharynx, pharynx, and larynx have squamous epithelia and therefore contribute little to humidification. The mucous membranes of the nose, trachea, and bronchi have many mucus glands, and it is here that the majority of humidification takes place. These glands keep the upper airway mucosa moist by direct secretion and transudation of fluid.
Respiratory mucus is formed within goblet cells in response to irritation of the tracheal surface. Mucus is secreted in 1- to 2-μm droplets that absorb water, swell, and coalesce to form a 10-μm film around respiratory cilia. It lies in two layers: the superficial layer is a viscous fluid that contains lipids, glycoproteins, and proteoglycans that trap inspired particles. The deep layer is a more acidic, watery, periciliary layer that contains antibacterial compounds secreted from surface epithelium and glands that, when activated, result in recruitment of inflammatory cells, such as neutrophils and macrophages.
Respiratory cilia are the basic units of the mucociliary escalator. Each ciliated cell has approximately 200 cilia, 5- to 6-μm long projections revealed in cross-section to be formed by a central pair of microtubules surrounded by nine pairs of tubules. Movement is achieved by microtubules sliding against each other by dynein adenosine triphosphatase linking arms. At their tips they have three to seven short, 25- to 35-nm long projections that effectively grip the superficial mucous layer. Cilia beat at approximately 1000 strokes/min in a coordinated fashion, propelling mucus cephalad at a rate of 12 to 15 mm/min. Each stroke has two phases, a rapid forward stroke, in which the side “claws” grip the superficial mucous layer and propel it forward, and a recovery stroke, in which the cilia swing slowly back into the starting position through the deep periciliary layer ( Fig. 7-2 ).

Particulate matter transported to the upper airway may be cleared by coughing, which involves rapid inspiration followed by brief closure of the glottis. During this time, elastic recoil and active expiratory contraction increase intrathoracic pressure. When the glottis opens, explosive expiration at over 10 L/sec causes shearing and expectoration of secretions. Effective coughing requires an adequate peak expiratory flow rate and adequately hydrated mucus secretions.
Mucociliary Clearance
Mucociliary clearance may be reduced as a result of abnormal mucus production or cilial activity. In cystic fibrosis, the abnormal transmembrane conductance protein is a chloride ion channel. This results in reduced chloride and water content in the mucus, making it abnormally viscous and thereby reducing clearance and encouraging bacterial colonization. In other inflammatory airway diseases, hypertrophy of mucus glands may result in viscous secretions and a reduced periciliary watery layer that may inhibit the ciliary recovery stroke.
Reduced cilia activity may be congenital in conditions such as primary ciliary diskinesia, in which ciliary ultrastructure is abnormal. The time taken for effective ciliary clearance is approximately doubled in cigarette smokers. Pathogens such as Pseudomonas aeruginosa and Haemophilus influenzae reduce beat frequency. In addition, ventilated critically ill patients may have reduced mucociliary transport as a result of loss of cilia.
Consequences of Underhumidification
In the presence of inadequate ambient humidity, prolonged excessive mucosal water loss results in viscous mucus that inhibits ciliary movement, especially the recovery stroke in the depleted deep, watery layer. Mucus flow is significantly reduced below a relative humidity of 50% at 37° C (absolute humidity 22 mg/L). Dry membranes have a reduced capacity to humidify inspired gas, and the ISB moves distally, exposing more distal airways to risk of injury. Ciliary loss, mucosal inflammation, epithelial ulceration, and necrosis may eventually occur. Hyperviscous secretion may obstruct bronchi or endotracheal tubes, and encrustation may occur and cause sputum retention, infection, atelectasis, reduced functional capacity, ventilation/perfusion (V/Q) mismatch, and reduced compliance. In addition, ventilation with dry gases may exacerbate hypothermia, especially in neonates and children.
Consequences of Overhumidification
When inspired gases are delivered at approaching or exceeding body temperature and fully saturated, the normal humidifying and heating actions of the upper airway cannot occur and may even be reversed. Condensation of water occurs in the airway with consequent reduced mucosal viscosity and risk of water intoxication. Inefficient mucociliary transport results, along with increased airway resistance, risk of pulmonary infection, surfactant dilution, atelectasis, and V/Q mismatch. Hot, humid gases may cause tracheitis by direct thermal injury to the epithelium.
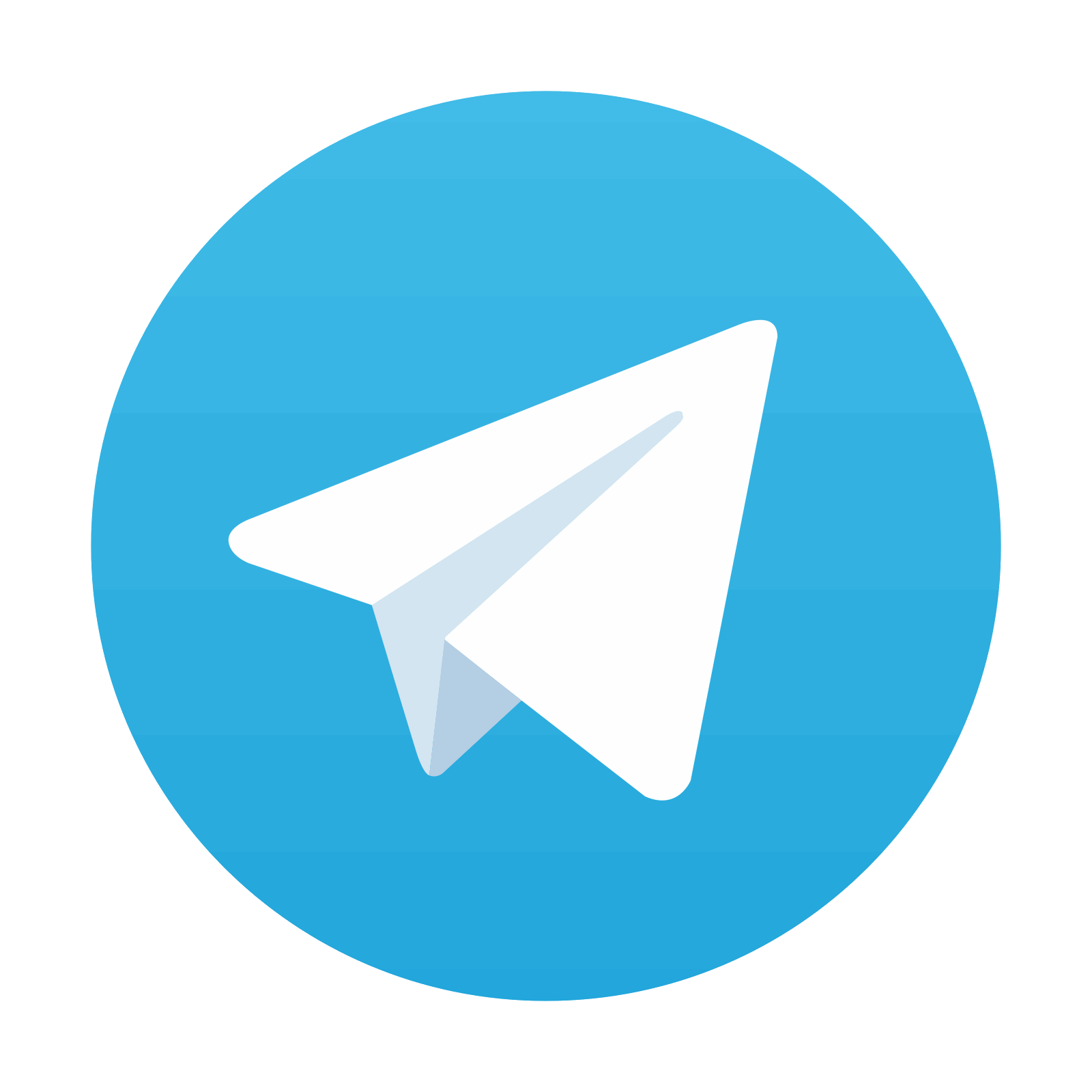
Stay updated, free articles. Join our Telegram channel

Full access? Get Clinical Tree
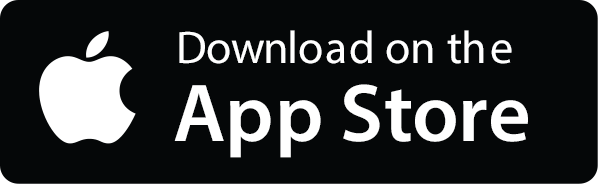
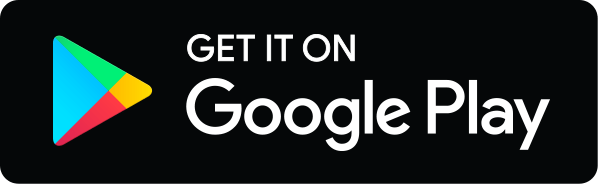