Chapter Outline
VIGILANCE AND MONITORING PERFORMANCE
Research on Vigilance in Anesthesia
ROLE OF STANDARDS IN ANESTHESIA EQUIPMENT DESIGN
Problem of Physical Integration
Computers in Integrated Monitoring Systems
Data Management in Integrated Displays
Design Considerations for Integrated Displays
Modern Integrated Operating Room Monitor
Overview
This chapter discusses several areas in which the interface between human and machine—or more accurately, between anesthesiologist and anesthesia equipment—plays a crucial role in patient outcomes. At first glance, the topics may seem unrelated. However, vigilance, alarms, and integrated monitoring systems are, in fact, closely interrelated.
The administration of anesthesia is predominantly a complex monitoring task and, as such, requires sustained vigilance. Unfortunately, humans are not very good at monitoring because we are error prone, and our vigilance is susceptible to degradation by a variety of human, environmental, and equipment factors. Designers of anesthetic equipment therefore have attempted to aid the anesthesiologist by incorporating devices and systems that augment vigilance and clinical performance. Alarms intended to notify the operator of potentially critical situations are effective only if properly designed and implemented. Although many modern anesthesia delivery devices are physically integrated and generally contain systems for gas delivery, monitoring, alarms, and sometimes record keeping, many of the promised benefits of full-scale integration (e.g., “smart” alarms, decision aids) are as of yet unfulfilled. The successful implementation of comprehensive integrated anesthesia workstations will require further technologic advances as well as a more complete understanding of the task of administering anesthesia and the factors that affect performance of the anesthesiologist in this complex human/machine environment. Research to elucidate these “performance-shaping factors” in anesthesia has been under way for a number of years and is beginning to bear fruit. Chapter 24 discusses the role that the field of human factors should play in modern anesthesia practice.
Anesthesia Mishaps
In 1985, as many as 3000 preventable incidences of anesthesia-related death or brain damage were estimated to occur in the United States each year. Although some believe that anesthesia has become safer, many more surgical procedures are now being performed; thus the absolute number of adverse events may not be appreciably decreasing. In addition, patients undergoing surgery today may be older and sicker than they were 10 or 20 years ago, a consequence of population demographics and economically driven changes in the American health care delivery system—a fact that might delay, but will not prevent, the use of surgical therapy. A French group found a 10-fold reduction in anesthesia-related deaths between 1979 and 1999. Finally, studies suggest that the incidence of “potentially serious” clinical events is actually quite common in anesthetized patients, but relatively few evolve into adverse patient outcomes.
A number of investigators have suggested that human error is a major contributor to the occurrence of anesthetic mishaps. For example, clinician errors, such as making an accidental gas flow change or a “syringe swap,” accounted for up to 70% of anesthetic mishaps in two early studies. In a study by Keenan and Boyan, 75% of intraoperative cardiac arrests were attributed to preventable anesthetic errors; an analysis of incidents under monitored anesthesia care concluded that nearly half of the claims could have been prevented by better monitoring. Holland suggested that inadequate patient observation was a contributing factor in one third of perioperative patient deaths.
In the intensive care unit (ICU), clinical errors often may be associated with failed communication between care providers. On the other hand, as is discussed in detail later in this chapter, the majority of adverse anesthesia incidents are probably the result of systemic factors over which the anesthesia provider has little or no control, such as device designs that predispose to human error.
Even under ideal conditions, performance on complicated tasks is rarely perfect. In complex systems involving both humans and machines, human error is almost always a factor in degraded or faulty performance. For example, the percent of accidents that resulted from air crew error was reported to be greater than 50%. Accidents usually are caused by the cumulative effect of a number of events rather than one isolated incident. Why do highly trained and experienced individuals make errors? What factors influence the occurrence of these errors? What can be done to decrease their incidence or to mitigate their negative outcome? Research has only begun to provide adequate answers to these important questions.
Human Error
Errors are a normal component of human cognitive function and play an important role in learning. Most errors do not result in damaging consequences, but when an error results in an unacceptable outcome, it often is called an accident. An error is most likely to deteriorate into a damaging situation when conditions prevent the appropriate corrective responses. Errors committed by anesthesiologists can have catastrophic consequences if not corrected, yet Cooper and colleagues showed that most critical events in anesthetic practice were discovered and corrected before a serious mishap occurred. Therefore it is crucial to understand the determinants of recovery from anesthetic errors; factors such as sleep deprivation, miscommunication, or equipment problems can increase the potential for error as well as preclude effective recovery.
Two types of error are slips and mistakes ( Table 23-1 ). Both of these can take the form of errors of omission (omitting a task step or even an entire task) or errors of commission (incorrect performance). Slips are most likely to occur during activities for which one is highly trained and that are therefore performed outside active conscious thought. Drug syringe swaps, a commonly described anesthetic critical event, are a type of slip. Errors of omission can occur when unexpected distractions interrupt a well-established behavioral sequence. Errors of commission occur when automated schema, or preprogrammed subroutines, are inappropriately called into play by specific stimuli without conscious processing. Individuals have a tendency to revert to a high-frequency (well-learned) response in such situations, particularly when under stress. In fact, experts may be more likely than novices to make these kinds of errors.
Type of Error | Description |
---|---|
Mistake | Inappropriate intention or action, often the result of a lack of training or knowledge |
Slip | Appropriate intention or action at inappropriate time |
Mode error | Erroneous classification of the situation |
Description error | Ambiguous or incomplete specification of intention |
Capture error | Correct schemata at incorrect time, often because of task overlap |
Faulty activation | Activation of inappropriate action or failure to activate appropriate action or triggering |
Data-driven error | Automatic actions inappropriate for the situation but called into play by ongoing action sequences |
Fixation errors | Failure to revise actions with changing conditions, “cognitive lockup” |
Confirmation bias ∗ | Tendency to seek confirming data for existing hypotheses |
Representational Errors | Faulty mental model of the system and its function or malfunction |
∗ Errors may also occur as a result of a variety of others types of cognitive bias, such as availability, representativeness, similarity, framing, and anchoring.
In a study of anesthesia residents working a comprehensive anesthesia simulation environment, DeAnda and Gaba documented 132 unplanned incidents (i.e., not part of the simulation script but rather created by the subject) during 19 simulations, at a rate of nearly two per case. Human error accounted for 86% of the incidents, whereas equipment failure only accounted for 3%. Of the incidents that resulted from human error, nearly one fourth were the result of so-called fixation errors, which occurred when the subject was unable to focus on the most critical problem at hand because of persistent, inappropriate attention or actions directed elsewhere. The overall incidence of human error observed during simulated anesthesia was similar to that suggested by Cooper and colleagues for anesthesiologists in the operating room (OR). This study is important because it substantiates the frequent occurrence of error in anesthesia and validates the use of simulation to study the types and causes of critical incidents in anesthesia. Clinical decision making also can be adversely affected by a number of other types of cognitive biases, such as confirmation bias, inappropriate overconfidence, false attribution, the availability and representativeness of heuristics, and anchoring and framing.
In contrast to slips and fixation errors, mistakes are technical or judgmental errors. Thus mistakes are due to inadequate or incorrect information, poor decision-making skills or inappropriate strategies, inadequate training, lack of experience, or insufficient supervision or backup.
People are more likely to make errors when they are mismatched to the task or the system is not user friendly. Factors that can influence error commission include skill level, attitude, inexperience, stress, poor supervision, task complexity, and inadequate system design ( Table 23-2 ). The topic of human error in anesthesia has been covered in some detail elsewhere.
Cause of Error | Representative Example |
---|---|
Human Factors | |
Task complexity | Not ventilating when coming off cardiopulmonary bypass |
Lack of training or experience | Rapid administration of vancomycin or protamine |
Stress | Drug syringe or ampoule swap during critical situation |
Ill health | Under the influence of prescribed or recreational substances |
Environmental Factors | |
Noise/miscommunication | Misheard surgeon, gave wrong antibiotic |
Workplace constraints | Circuit disconnect from moving equipment or personnel |
Equipment and System Factors | |
Poor equipment design | Lightbulb goes out on laryngoscope during a procedure |
False and/or noisy alarms | Failure to recognize critical situation after disabling alarms |
Mismatch of human/machine functions | Failure to detect ongoing event while manually recording vital signs |
At least some of what, on first glance, appears to be human error often can be traced back to poorly designed human/machine interfaces. In fact, Norman suggests that “the real culprit in most errors or accidents involving complex systems is, almost always, poor design.” Poor operational design can substantially increase the risk of system failure as a result of operator error. Factors related to system-induced error include boredom attributable to overautomation, overreliance on automated devices, and poor team coordination. Good operating practice is essential but not sufficient for minimizing system risk: 1) the design of the system must be fundamentally sound, 2) it must be properly constructed and implemented, 3) the operators must be thoroughly familiar with the system, and 4) ongoing quality control must ensure that system use is appropriate over the full range of possible conditions. This applies to the anesthesia workspace and must be considered when introducing new anesthesia equipment to this unique environment.
Vigilance and Monitoring Performance
Vigilance has been equated with “sustained attention.” Attention requires alertness, selection of information, and conscious effort; alertness indicates the receptivity of the individual to external information ( Table 23-3 ). Mackworth, the father of vigilance research, defined vigilance as “a state of readiness to detect and respond to certain specified small changes occurring at random intervals in the environment.” Early research was stimulated by the errors of radar operators who performed the task of detecting barely perceivable events at infrequent and aperiodic intervals for extended periods.
Term | Definition |
---|---|
Perception | To attain awareness or understanding, usually via the senses |
Attention | A conscious effort to remain alert and to perceive and select information |
Vigilance | A state of readiness to detect and respond to changes in the monitored environment; a state of “sustained attention” |
Monitoring | A vigilance task involving the observation of one or several data streams in order to detect specified changes that often occur at random intervals |
Vigilance | A state of clinical awareness whereby dangerous conditions are anticipated or recognized |
Judgment | The formation of an opinion or evaluation based on available information |
Cognition | The act or process of knowing, including both awareness and judgment |
Decision making | The act of choosing between alternative diagnoses or possible actions based on judgments |
Situation awareness | A coherent mental model or picture of the current state of a complex dynamic system, including an understanding of prior conditions and the implications of ongoing processes to future states |
The presence of “vigilance” in the official seal of the American Society of Anesthesiologists (ASA) underscores the perceived importance of careful attention to details and detection of subtle signs out of the ordinary. Thus, in a broader sense, anesthetic vigilance might be viewed as a state of clinical awareness in which dangerous conditions are anticipated or recognized. Monitoring , by definition, is a vigilance task; the administration of anesthesia is a complex monitoring task. The anesthesiologist must continuously evaluate the patient’s medical status while assessing the effects of anesthesia and surgical intervention. Memory tasks, decision making, and vigilance are the most vulnerable to compromise under the stressful work conditions often experienced in the OR. Although monitoring during quiescent periods of the maintenance phase of anesthesia appears to closely resemble the classic vigilance tasks studied in the laboratory, anesthesia practice commonly involves more complex situations that require divided attention, prioritization, and “situation awareness,” skills that fall outside the classical definition of vigilance.
A large number of laboratory studies have demonstrated a decline in monitoring performance over time, called the vigilance decrement . This performance decline typically is complete within the first 30 minutes of a monitoring session. The vigilance decrement seems to arise primarily as a function of the necessity of attending to a relatively infrequent signal for a prolonged length of time.
Psychologists and engineers have studied vigilance for many years. Investigators in fields outside medicine, most notably aviation, have applied this information to understanding performance on complex monitoring tasks. Studies have identified environmentally induced factors and human/machine interface variables that can impair vigilance and performance in air traffic control, train driving, automobile driving, and nuclear power plant control. The armed forces consider the potential impact of such factors at the earliest stages of the design of new weapons systems.
In most complex monitoring tasks, increased task complexity or task duration generally results in impaired performance ( Fig. 23-1 ). A major factor in the effect of additional tasks on performance appears to be what personal resources, perceptual or cognitive, are required for each new task, and whether those resources are already taxed. Other factors known to impair vigilance include noise, environmental pollution, fatigue, sleep deprivation, and boredom. Performance also may be impaired if the individual is under stress, is in poor health, or uses drugs. Personality factors, training, and experience also affect performance, and performance on complex monitoring tasks can be strongly affected by environmental or task variables.

Research on Vigilance in Anesthesia
In one of the first ergonomic studies of anesthesia, Drui and colleagues used time-motion analysis to examine how anesthesiologists spent their time in the OR. The practice of anesthesia was divided into a number of discrete activities, and the frequency and sequence of each activity were measured. One principal finding was that anesthesiologists directed their attention away from the patient 42% of the time. Subsequent time-motion studies have corroborated and expanded these early results.
Subsequent studies have examined the effects of the level of provider experience and of new technologies, such as electronic anesthesia record keeping, on the workload and vigilance of residents administering anesthesia. Others have investigated anesthesiologists’ vigilance to auditory and visual alarm cues and to changes in clinical variables in both the laboratory and during actual procedures. For example, Weinger and colleagues demonstrated that novice anesthesia residents were slower to detect the illumination of an alarm light placed within their monitoring array ( Fig. 23-2 ). The response rate was further impaired during periods of high workload, such as during the anesthetic induction.

Well-controlled studies are essential to understand the nature of anesthesia vigilance and monitoring performance. Studies should be designed to use techniques and procedures that have been repeatedly validated by investigators in other fields. Chapter 24 describes in more detail current research in anesthesia related to clinical performance.
Factors that Affect Vigilance
A wide range of factors can affect vigilance and clinical performance in anesthesia ( Box 23-1 ). The following discussion provides a perspective on how a variety of everyday occurrences in the OR have the potential to significantly impair vigilance, potentially leading to increased risk of critical events and, as a result, anesthetic morbidity or death. The following sections address some of the more important performance-shaping factors organized into environmental, human, and equipment categories.
Environmental Factors
- •
Noise
- •
Temperature and humidity
- •
Environmental toxicity
- •
Ambient lighting
- •
Workspace constraints
Human Factors
- •
Human error and cognitive biases
- •
Fatigue
- •
Sleep deprivation (acute and chronic)
- •
Circadian effects and shift work
- •
Boredom
- •
Substance use/abuse
- •
State of health and stress
- •
Aging
- •
Training and experience
- •
Psychosocial factors
- •
Personality factors
Task and Information Factors
- •
Primary task load
- •
Secondary task intrusion
- •
Interruptions and distractions
- •
Misinformation
- •
Alarms and warnings
Interpersonal and Team Communications
System and Equipment Factors
- •
System-induced errors (e.g., latent errors)
- •
Equipment failure
- •
Equipment-induced errors
- •
Faulty mental models of equipment design/function
- •
Clumsy automation
Environmental Factors
Noise and Music
The noise level in an OR can be quite high. In the early 1970s, Shapiro and Berland measured noise levels associated with specific tasks in the OR during several typical surgical procedures and found that the noise in an OR “frequently exceeds that of a freeway.” These findings appear to still be valid; continuous background noise in the modern OR may range from 75 to 90 dB ( Table 23-4 ). High-noise events include mechanical ventilation, suction, music, and conversation. Noise levels up to 118 dB can occur, notably during the operation of high-speed gas-turbine drills; suction tips with trapped tissue yielded up to 96 dB. High noise levels create a positive feedback situation; noisy rooms require louder alarms and louder voices, which contribute to the noise, and so on.
Sound | Decibels |
---|---|
Jet ventilator | 120 |
Monitor alarm sounding(all alarms at highest setting) | 91 |
Humidifier (temperature probe not plugged in) | 86 |
Tourniquet (disconnect alarm) | 84 |
Anesthesia machine (loss of oxygen supply) | 84 |
Anesthesia machine (circuit disconnect) | 78 |
Infusion pump (inclusion alarm) | 77 |
Surgical instruments clanking against each other in a metal basin | 75 |
Monitor alarm sounding (all alarms; at standard setting) | 74 |
Electrocautery unit (return fault) | 74 |
Intercom | 72 |
Tonsil tip suction | 70 |
Pulse oximeter tone (maximum volume) | 66 |
Surgeon’s conversation (at patient’s ear) | 66 |
“Background” music at patient’s ear | 65 |
The effects of noise on performance depend on the type of noise and the task being performed. In addition, other environmental and human factors can interact with noise to affect task performance. Noise levels similar to those found in ORs detrimentally affect short-term memory tasks and also may mask task-related cues and cause distractions during critical periods. Difficult tasks that require high levels of perceptual and/or information processing are negatively affected by noise. Long-term exposure to high noise levels produces physiologic changes consistent with stress. Exposure to loud noise activates the sympathetic nervous system, a situation that may augment the effects of other performance-shaping factors and result in impaired decision making during critical incidents.
There is little doubt that background noise interferes with effective verbal communication. It is critical for the anesthesiologist to be able to hear clearly what other members of the OR team are saying. When multiple tasks are required, the presence of background noise may bias attention toward the dominant task. Although loud noise is clearly disruptive and can impair auditory vigilance—for instance, the ability to hear changes in pulse oximeter tone or to detect and identify alarms—studies have found a beneficial effect on complex task performance in the presence of lower levels of background (white) noise.
Several studies have suggested that the presence of familiar background music could improve vigilance. A positive effect of preferred background music on surgeons’ mood and laboratory task performance was described. However, the validity of this study’s findings were questioned, with no data yet available on the impact of the surgeon’s preferred music on the anesthesiologist’s monitoring performance. What if the surgeon wanted to listen to country music, for example, and the anesthesiologist did not like that type of music? Swamidoss and colleagues studied the effects of background music on the performance of 30 anesthesia providers using a screen-based computerized anesthesia simulator. They found no significant differences in level of anxiety, time to recognition and correction of critical incidents, or autonomic responses whether subjects listened to their “most enjoyed music,” “least enjoyed music,” or “no music” at all. However, the number of subjects studied was small, and the applicability of these results to the actual OR environment remains to be examined.
Temperature
Uncomfortable environmental temperatures, a common situation in many ORs, can impair vigilance. Although there appears to be significant variability in the effects of temperature on performance depending on the experimental situation (i.e., other environmental, task, and subject variables), as a general rule, temperatures that promote general fatigue decrease performance. Extremely cold temperatures have a deleterious effect on some cognitive tasks, primarily because of the distraction of the cold environment and the associated decrease in manual dexterity. These effects often show up as increased errors and memory deficits. Studies in the industrial workplace suggest that when temperatures fall outside a preferred range (17° to 23° C), workers are more likely to exhibit unsafe behaviors that could lead to occupational injury. Temperatures in some adult ORs can be as low as 7° C, and those in pediatric ORs may approach 30° C. The negative effects of temperature probably are augmented by other factors that enhance fatigue or impair performance.
Environmental Toxicity: Exposure to Vapors
Voluminous literature has been published on the effects of trace anesthetic vapors on anesthesiologist performance. The early studies of Bruce and colleagues reported that exposure to 550 ppm N 2 O and 14 ppm halothane led to a significant decrease in performance on complex vigilance tasks. However, their study used as subjects Mormon dental students, who may have been uniquely sensitive to the effects of the anesthetic gases. Smith and Shirley subsequently showed that acute exposure to trace anesthetic gases in amounts commonly seen in an unscavenged OR had no effect on performance in naive volunteers. It thus appears that impaired vigilance related solely to trace anesthetic gases is probably not a problem in the modern, well-scavenged OR. A subsequent, well-controlled crossover study of anesthesiologists showed no differences in either mood or cognitive ability when working in a scavenged OR compared with working in an ICU (i.e., with no trace gases). However, other noxious smells or the need to wear bulky and uncomfortable protective gear could have an adverse impact on clinical performance.
Individual Factors
Fatigue
Fatigue is caused by hours of continuous work or work overload, whereas boredom is believed to be a function of insufficient work challenge or understimulation. The two, nonetheless, often co-occur. Extreme fatigue results in objectively measurable symptoms of exhaustion and a psychological aversion to further work. There is marked individual variability in the response to factors or situations that can produce fatigue. The continued ability to perform skilled physical or mental tasks in the face of worsening fatigue strongly depends on psychological factors, including motivation. Although some extremely fatigued individuals can be induced to perform, the quality and wisdom of continued work under these circumstances is questionable, certainly so in situations in which human lives may be at stake.
Few fatigue studies have used physicians as subjects. Those that have typically involved sleep loss and, primarily because of poor methodology, raised more questions than they answered. Fatigue and sleep loss often are covariants in studies that examine continuous, long work schedules; in turn, both are modulated by circadian processes. Because the effects of these variables interact, it is difficult to separate the relative contribution of each factor to the performance decrement observed.
Individuals subjected to excessive work, fatigue, or inappropriate shift schedules show degraded performance, impaired learning and thought processes, irritability, memory deficits, and interpersonal dysfunction. Fatigued subjects pay less attention to peripherally located instruments and are inconsistent in their response to external stimuli. When coping with task demands, they exhibit less control over their own behavior and tend to select more risky alternatives (shortcuts). If sufficiently motivated, fatigued subjects can attain relatively normal performance on tasks of short duration, but they find it difficult to sustain performance on vigilance or monitoring tasks of long duration. Adding sleep loss or shift work accentuates fatigue-induced performance decrements.
Sleep Deprivation
A large body of research supports the contention that sleep deprivation and circadian rhythm disturbances can dramatically impair performance on monitoring tasks. Although sleep deprivation and fatigue are similar in some of their performance-shaping effects and certainly are interactive, they are different processes. A single night of sleep loss can produce measurable performance decrements, especially on skilled cognitive tasks. Impairment can be seen shortly after task initiation—within 20 to 35 minutes in some situations. However, in most sustained work activities, major decrements usually occur after 4 hours and again after 18 hours. Dawson and Reid showed that the magnitude of cognitive psychomotor impairment after remaining awake for 24 hours was roughly equivalent to that produced by acute inebriation (a blood alcohol level of 0.10%) ( Fig. 23-3 ).

Although wide individual differences exist in the amount of daily sleep required, studies that involve 1 or more days without sleep consistently reveal progressive decreases in reaction time and increases in response variability ( Box 23-2 ). Work rate is appreciably slowed, particularly when subjects are required to make choices. In vigilance tasks, omission errors increase, whether or not visual or auditory signals are presented. Sleep loss impairs active use of working memory, particularly when the sleep loss precedes learning. Sleep-deprived workers fail to appropriately allocate attention, set task priorities, or sample for sources of potentially faulty information. Because many of these skills are essential for optimal anesthesia care, this suggests that sleep loss could be extremely detrimental to clinical performance.
Decreases in reaction time
Increases in response variability
Decreases in work rate
Difficulty making choices
Increases in omission errors
Impaired working memory
Failure to appropriately allocate attention
Difficulty setting task priorities
Failure to evaluate potential faulty information
Haslam’s early field work indicated that some sleep (2 to 3 hours per day) is better than none at all, at least for soldiers involved in military exercises. For example, in studies of sleep-deprived soldiers, a 2-hour nap was insufficient and a 3-hour nap permitted maintenance of previous levels of already impaired performance; a full 4-hour nap was required before baseline performance was restored. Taking a short nap (<2 hours) at the circadian low point produces greater cognitive impairment than the same length nap taken at the peak of the circadian cycle. In addition, if a sleep-deprived subject is permitted to nap, a period of “sleep inertia” will follow the nap, during which the subject will exhibit a low level of arousal as well as significantly impaired vigilance and performance. Sleep inertia may persist for up to 2 hours.
In one of the first studies of the effects of sleep loss on physician performance, Friedman and colleagues showed that the ability of sleep-deprived medical interns to detect cardiac arrhythmias was significantly compromised compared with well-rested interns. In a well-designed study of 30 first-year medical residents, Hart and colleagues demonstrated mild but significant disturbances in memory, decision making, and motor execution in on-call residents deprived of normal sleep (2.7 ± 2.2 hours slept) compared with those who got a full night’s rest (7.9 ± 1.3 hours). Unfortunately, many studies designed to assess the impact of sleep deprivation on the ability of physicians, typically house staff, to perform clinical duties have generally had serious methodologic flaws. One well-regarded study examined 20 interns in critical care units and compared their normal schedules (most worked more than 80-hour weeks) with an intervention period limiting them to 16-hour shifts. During limited work hours, the subjects slept significantly more and had half the attention failures, as defined by electrooculography, than in the control periods. In a similar study, the rate of serious medical errors, as determined by independent observers, was significantly reduced. Percutaneous injuries (needle sticks) were also significantly lower in interns who were not working extended hours. In one examination of complication rates after performing nighttime procedures, no differences were found unless the physician had sleep opportunities of less than 6 hours.
The issue of decreased clinical performance of house officers and other physicians as a result of overwork and sleep deprivation gained media attention in the late 1980s. Thereafter, state legislatures, academic institutions, and accrediting bodies implemented restrictions on house staff work schedules; however, there are still no restrictions on the work schedules of fully licensed practicing physicians or nurse anesthetists. As American health care evolves toward capitated managed care, and the financial incentives associated with extended work hours diminish, it will be interesting to see whether more anesthesia providers will voluntarily reduce their work schedules.
Circadian Changes and Shift Work
Periodic, rhythmic fluctuations in bodily processes, including performance and work efficiency, have been well documented. More than 50 neurophysiologic and psychological rhythms that potentially influence human performance have been identified. Most studies of rhythmic changes in efficiency have focused on cycles of approximately 1 day, called circadian processes . An individual’s normal rhythm can be significantly influenced by environmental conditions, illness, time zone changes, and altering shift schedules. During normal awake times, circadian-related fluctuations in performance can range from 14% to 43% ( Box 23-3 ).
Circadian-related fluctuations in performance
Inability to adjust to time changes
Difficulty establishing a normal diurnal rhythm
Sleep deprivation
Exacerbation of other performance-shaping factors
Health problems
Social and interpersonal problems
Increased incidence of on-the-job errors and accidents
In rapidly changing schedules of regular work hours (e.g., routinely and frequently changing from day shift to night shift), performance rhythm amplitudes show variations as great as 50%. The rate and amount of adjustment to shift changes or extended workdays vary among individuals. However, in a study of nurses doing shift work, some individuals were never able to adjust. In general, shift workers exhibit greater sleep, social, and health problems. Efficiency of permanent night shift workers is at least 10% less than that of comparable day shift coworkers, and minor accidents and task errors occur most frequently during night shifts and early morning hours. On the other hand, fully acclimated night shift workers have a realigned circadian cycle such that their best performance occurs during their normal night shift. Swing shift workers seem to have the most difficulty establishing a normal diurnal rhythm. Because adjustment to shift work takes several days, if shift rotations are required, the rotation should be clockwise—that is, with progressively later shifts—and never less than 2 weeks per shift. The implications for anesthesia is that middle-of-the-night procedures would be optimally performed by anesthesia providers assigned to at least 2 weeks of only night shift duty.
Alterations in the normal circadian rhythms cause changes in arousal as well as in other mental and physical functions. These changes play a major role in the effects on task performance of acute sleep loss, napping, and recovery from disruptions in normal sleep schedule. Phase shifts are introduced by sleep interruption. Some circadian functions are altered, and normal rhythms may be disrupted, even after multiple brief interruptions in an otherwise full night’s sleep. The peak and minimal performance times normally expected by the individual are similarly shifted. This can lead to a false sense of competence during “normal working hours” following acute sleep loss. For example, an anesthesiologist who has been working most of the previous night, after recovering from sleep inertia in the early part of the next morning, may feel remarkably awake, perhaps even euphoric. Yet studies have documented degraded performance on complex tasks in such situations (see Fig. 23-3 ). By mid afternoon, dramatic decreases occur in arousal and the feelings of well-being that accompany parallel performance decrements. That evening, the anesthesiologist will probably have difficulty falling asleep, especially if an afternoon nap was taken. In fact, sleep-wake cycles can be disturbed for up to 36 hours, and the anesthesiologist may remain more error prone during this recovery phase.
Performance During Extended Duty Shifts by Anesthesia Personnel
Howard and colleagues have suggested that anesthesia residents are chronically sleep deprived. Using standard sleep study methodology, they demonstrated that undisturbed Stanford anesthesia residents, even if they had not been on call for 2 full days, had daytime sleep latencies comparable to those of patients with narcolepsy. Furthermore, these residents often denied falling asleep during the sleep tests despite objective electroencephalographic (EEG) evidence to the contrary. Post-call anesthesia residents demonstrate significant decrements on laboratory psychomotor vigilance tests.
Cao and colleagues examined the task performance and workload of anesthesia residents performing OR procedures in the middle of the night when on call. Five senior residents performed routine general anesthesia cases at night (between 10 pm and 6 am ) during a 24-hour on-call shift. The same residents were studied again during daytime cases (between 9 am and 5 pm ), which were prospectively matched to the nighttime cases with respect to type and duration of surgery and ASA status. The intraoperative activities of each resident, resolved into 37 task categories, were recorded by a trained observer. At night, more time was spent on observation, and each episode lasted longer. During maintenance, residents spent more time during the night observing their monitors and less time doing manual tasks. Subjective workload was significantly higher at night than during the day. There were no differences, however, in response to a vigilance probe.
These data suggest that in the sleep-deprived state, anesthesia residents perceive a higher workload and are less efficient, suggesting a potential for impaired performance. These results are consistent with the need at night for greater perceptual and cognitive resources to process data and accomplish tasks, although additional experiments will be required to substantiate this hypothesis.
Anesthesia trainees and practitioners may have significant decrements in psychomotor vigilance and reaction times after sleep deprivation. Stanford University anesthesia residents participated in a randomized, controlled crossover study designed to examine the effects on clinical performance of sleep deprivation and fatigue during realistic simulated OR cases. In the “highly fatigued” condition, subjects were kept awake for at least 25 hours prior to a morning experiment. In the comparison well-rested condition, the same resident obtained an extra 2 hours of sleep every night for 1 week before the experiment. The simulated 4-hour routine laparoscopy cases used in the study were designed to accentuate any effects of sleep deprivation. Interestingly, analysis of preliminary data suggest that, through the intraoperative use of a variety of countermeasures, anesthesia residents are able to overcome substantial fatigue and sleep loss to perform almost as well as during routine anesthesia cases. Unfortunately, the effects of sleep deprivation on clinical performance during serious, acute critical events have not yet been examined in either simulated or real cases.
It appears that work schedule can, under some circumstances, be an important factor affecting intraoperative vigilance and performance. Significant individual differences are seen in the response to acute or chronic sleep loss, and each anesthesiologist must be cognizant of his or her own limitations. Individuals must recognize that it is neither unprofessional nor weak to admit sleepiness or fatigue when on the job, and personnel must attempt to either make time to recuperate or seek a clinical replacement.
Breaks
Common sense suggests that relief from a prolonged monitoring task should enhance subsequent performance. Both anecdotal reports and laboratory studies have indicated that people prefer self-paced tasks and will take a break when needed. Short breaks have been shown to alleviate fatigue and increase employee satisfaction and productivity in machine-paced jobs. For worker-controlled sedentary jobs, short breaks or a change in activity increases performance and relieves boredom. However, little experimental evidence supports the widely held belief that performance will improve after a break from a prolonged complex monitoring task such as administering anesthesia.
The optimal frequency and duration of breaks is still unknown for most occupations. Warm has recommended that monitoring tasks be limited to sessions of less than 4 hours. Breaks have been required by many union contracts, as well as by legislation in some countries, particularly for occupations in which impaired worker performance could endanger worker or public safety, such as with transportation workers.
In a study of critical incidents associated with intraoperative exchanges of anesthesia personnel, Cooper and colleagues identified 90 incidents that occurred during a break. Twenty-eight of these incidents were deemed favorable (the relieving anesthesiologist discovered and corrected a potentially dangerous preexisting situation), and only 10 incidents were considered unfavorable (the relieving anesthesiologist “caused” the critical incident). In some of the remaining incidents, the problem was perpetuated by the relieving anesthesiologist. Unfortunately, because of the possibility of biased reporting, the relative frequency with which relief results in favorable versus unfavorable outcomes cannot be determined from this type of study. On the other hand, of the 1089 total critical incidents studied, Cooper and colleagues did not identify a single relief-related incident that resulted in significant morbidity or mortality. Nevertheless, the detection of problems during a break probably depends on a systematic and comprehensive review of the anesthetic course by the relieving anesthesiologist. Because of this, it has been recommended that specific relief-exchange protocols be developed and strictly adhered to ( Box 23-4 ). Several professional organizations are currently developing standardized anesthesia handover protocols and checklists.
- 1.
The relieving anesthesiologists must establish familiarity with:
- •
Patient’s preoperative status
- •
Course of the anesthetic
- •
Course of the surgical procedure
- •
Overall anesthetic plan
- •
Arrangement of the equipment, apparatus, drugs, and fluids
- •
- 2.
The two anesthesiologists must communicate relief plans with the surgical team.
- 3.
The original anesthesiologist must not leave the room until the relieving anesthesiologist is in control of the situation and has all of the necessary information to continue with the anesthetic.
- 4.
The original anesthesiologist should not leave the room if the patient is unstable or the anesthetic is not likely to remain in a steady-state condition for at least 5 to 10 minutes.
- 5.
Care must be taken to communicate all special information that is not recorded or may not be readily evident.
- 6.
Under normal circumstances, the relieving anesthesiologists should not appreciably alter the course of preexisting anesthetic management.
- 7.
Before resuming control, the original anesthesiologist should carefully go through these same steps with the relieving anesthesiologist.
- 8.
If the relieving anesthesiologist is to finish the case, special care should be taken to explain the anesthetic plan, including the “to do” list, “to watch for” precautions, and any special issues.
Boredom
Boredom is a problem of information “underload,” insufficient work challenge, and understimulation. Boredom typically results from the need to maintain attention in the absence of relevant task information and may be most likely to occur in semiautomatic tasks that prevent the mind from wandering but are not fully mentally absorbing. Substantial differences exist among individuals as to what types of activities they find boring. Nevertheless, boredom appears to be a major problem in many complex real-life tasks. For example, boredom may be a contributing factor to human error in driving a locomotive and in piloting a prolonged routine flight in high-performance and commercial aircraft.
The maintenance phase of most routine anesthetics is a period of very low workload and infrequent task demands. This low workload may result in a low arousal state, which can lead to impaired performance. In laboratory experiments, increased effort in the presence of boredom is necessary to suppress distracting stimuli and a generalized feeling of fatigue. The addition of other performance-shaping factors, such as fatigue and sleep deprivation, may augment the negative impact of boredom. Boredom may be minimized by altering the sequence of tasks or by adding tasks to a monotonous job. Dividing attention among several tasks (time sharing) will in some circumstances improve monitoring performance. Psychological studies suggest that some individuals may be more “boredom prone” but that behavioral interventions can improve these individuals’ vigilance.
Observation of experienced anesthesia providers has revealed that, during times of low workload, many add additional tasks to their routine. These secondary tasks include clinically relevant functions, such as rechecking the composition or organization of the anesthesia workspace. Alternatively, it is common to observe anesthesiologists reading, listening to music, attending to personal hygiene, or conversing with their intraoperative colleagues about matters unrelated to patient care. The choice of secondary tasks is probably less important than how those tasks are integrated with the primary tasks of caring for the patient and how easily and quickly the secondary tasks are set aside when anesthesia workload increases. From a broad perspective, if the anesthesia task environment is optimized to minimize boredom and yet not be as continuously busy as to be stressful, the highest consistent levels of vigilance and performance will be attained.
Reading in the Operating Room
The anesthesia community has discussed the appropriateness of the apparently common practice of the anesthesiologist reading while caring for anesthetized patients. Because no objective data exist on the effects of intraoperative reading on patient outcomes, the opinions espoused are largely based on personal beliefs and morals (what is “right”).
Most of the time during the administration of an anesthetic, many patient care tasks must be performed, and the diligent anesthesia provider will prioritize and undertake these tasks appropriately. Under this circumstance, if reading occurs, it will only be during “idle time,” when no tasks other than general patient monitoring are required. In task analysis studies, the anesthesiologist has been shown to be idle up to 40% of the time during routine cases. This idle time appears to provide a reserve, or spare capacity, that can be called into play during high workload or critical periods, when additional cognitive and physical resources must be rapidly deployed to optimize patient care. Studies suggest that more experienced providers perform tasks more efficiently, report lower workload, and have more spare capacity at a given level of task performance than do less experienced providers. It could be speculated that reading during low workload periods could help prevent boredom, sleepiness, and decreased vigilance; however, this hypothesis must be tested in rigorous scientific studies.
Few studies have defined the actual incidence of boredom or of reading in the OR. Some years ago, 57 of 105 anesthesia providers at one institution responded to a questionnaire that included questions on this topic. Almost 90% of those who responded admitted to occasional episodes of “extreme” boredom. To relieve their boredom while in the OR, 29% of the respondents read. Reading was the most common technique to relieve intraoperative boredom; other strategies included “thinking about things,” “conversing,” and “busying oneself with manual tasks.” When asked specifically, “How often do you read while administering anesthesia,” 19% of the respondents stated that they read “frequently,” 46% said they “sometimes” read, and 33% “rarely” read. Only one respondent claimed that he or she never read in the OR. When they did read, two thirds of the respondents almost always read anesthesia-related material.
Interestingly, despite its common occurrence, 49% of the respondents believed that reading detracted from anesthesia vigilance, 21% believed that reading enhanced vigilance, and 30% were ambivalent. These data are from a Southern California training institution in which intraoperative reading is permitted; it is unclear how these data might generalize to other facilities.
In one institution, trained observers monitored the anesthesia provider in 172 cases and categorized the tasks observed, also noting whether reading was observed. Reading was seen in 35% of cases, almost universally during the maintenance phase. During periods of higher workload, reading was absent, suggesting a “titration” of reading to balance workload.
Laboratory studies suggest that a discrete time-sharing ability can be separated from other vigilance skills and that it can be taught. However, anesthesia providers are not given any formal training in time-sharing techniques, although resource allocation and divided attention skills are probably learned on a more informal basis. Tremendous individual variability likely exists in the impact of reading on anesthesia vigilance. For some anesthesia providers, intraoperative vigilance could thus be enhanced by reading during low-workload periods, but in others, the ability to detect acute events may be impaired.
It is also important to be cognizant of the sociopolitical and medicolegal implications of intraoperative reading. Reading will clearly have an adverse impact on performance if it detracts from the anesthesiologist’s ability to do the primary tasks of the job, attend to the surgeons’ and others’ requests, or to respond to new task demands. However, even in the absence of a demonstrable negative impact, reading may simply “look bad” and may give the appearance of inattention and boredom, when it may have the opposite effect. However, in the most recent work on intraoperative reading, no evidence of a change in provider response times to random visual stimuli (as an indicator of vigilance) was demonstrable.
More timely is the issue of distraction from computers, smart phones, or other personal electronics. Although much can be extrapolated from the literature on reading, attention is now turned to the effects that texting and other activities may have on attention. Handheld devices, simultaneously distracting and useful, are becoming ubiquitous. They are also being adapted to “push” information to clinicians as a part of the patient care and monitoring infrastructure.
Stress and Performance
Sources of stress that affect performance can be found in the work environment and may be social and physical or related to the tasks involved and their mental load and pacing; individual stressors may be health related or may arise from job matching and personality. Stress is a broad term. Depending on its type and magnitude, it can result in either degraded or enhanced performance. A subject’s performance will be significantly influenced by interaction with the environment and its associated stressors, the work to be performed in this environment, and the level of incentive for performing.
Many personal interactions in the OR can adversely affect performance, such as dealing with the ostensibly difficult surgeon or uncooperative nurse. Other, outside factors can also influence an anesthesiologist’s performance, such as financial worries or a recent fight with a spouse. Such domestic stressors have been shown to increase the likelihood of accidents, although being in a bad mood (“induced state negative affect”) does not prolong reaction times.
Stressful environmental conditions impair vigilance, especially in situations of conflict. The level of stress can be assessed by measuring either physiologic or psychological parameters. The physiologic correlates of stress generally correspond to sympathetic nervous system activity. Studies have used heart rate, skin conductance, respiratory rate, beat-to-beat variability in heart rate, T-wave peak amplitude on electrocardiogram (ECG), changes in voice characteristics, and catecholamine excretion to assess stress levels during performance of complex tasks. With increasing workload levels during simulated and real flights in high-performance aircraft, pilots who exhibited physiologic signs of stress had more false alarms and exhibited more disorganized task patterns. Thus increased workload or mental stress produces increases in sympathetic nervous system activity that can lead to deleterious physiologic changes.
The physiologic response of the anesthesiologist to the stress of giving anesthesia may be a crucial variable, yet it has received little attention. In an early study, Toung and colleagues measured the heart rate of anesthesiologists during anesthetic inductions and found a 60% increase over baseline heart rate in first-year residents at the time of intubation ( Fig. 23-4 ). More experienced clinicians showed less of an increase. Subsequently, these investigators showed that prior medical training, even if not anesthesia related, was associated with a diminished stress response to the administration of anesthesia. Repeated exposures to a specific situation result in diminished endocrine (stress) responses if the subject has learned to cope with the situation. A correlation between clinical workload and the heart rate of anesthesia residents has since been documented, and in another study, the investigators replicated Toung’s findings and demonstrated that more emergent procedures generated a greater physiologic stress response. Kain et al documented increases in heart rate and blood pressure of anesthesiologists monitored during their work, especially during induction, although these changes were described as “clinically insignificant.” A more recent study of multiple workload measures found consistent increases in heart rates and workload, both self-reported and observed, during anesthetic induction and emergence. The induction-associated tachycardia was greatest in junior trainees. Comparison of teaching and nonteaching cases also produced evidence that clinical teaching increased the workload of the instructor and reduced measures of vigilance.

State of Health
Physicians, like the patients they care for, develop both physical and mental illnesses. The topic of the “impaired” physician has been gaining increasing attention among professional societies, consumer groups, and government regulators. For example, how can these various constituencies be assured that an anesthesiologist infected with HIV does not have neuropsychologic impairment that adversely affects his or her ability to safely administer anesthesia? What should be done with practicing anesthesia providers who are elderly or infirm or those who have been involved in a previous anesthesia mishap? These are complex issues that require more discussion than is possible in this overview.
As individuals age, physiologic and neurologic changes can adversely impact vigilance and task performance. Ample “reserve” and years of experience allow most older anesthesiologists to continue to perform at a high level under most circumstances. However, the older practitioner may be more susceptible to the performance-degrading effects of sleep deprivation, fatigue, and stress. In fact, a retrospective analysis by Travis and Beach suggests that older anesthesiologists are proportionally overrepresented in the National Practitioner Data Bank for malpractice claims, although in general, older physicians do not seem to have higher rates of claims. Many older anesthesiologists do recognize their limitations and may restrict their practice to routine daytime work. Some also choose to retire rather than continue to work at night.
Anxiety is a major stress factor that can affect job performance. Anxiety adversely affects attention and working memory, and stress-related memory failures probably cause the difficulties in planning and decision making observed in stressed individuals. In addition, the inability to cope with anxiety and stress is likely an important contributing factor in the development of mental illness and substance abuse.
Burnout is a phenomenon first described in the 1970s by Freudenberger and subsequently quantified into components of emotional exhaustion, depersonalization, and reduced sense of personal accomplishment. In a recent study by Hyman and colleagues of perioperative clinicians, they found that residents in anesthesiology had higher burnout scores than attending anesthesiologists or nurses, whereas gender was not predictive of burnout. Riad found that anesthesiologists had significantly higher self-reported components of work-related exhaustion than ophthalmologists or ancillary staff.
Dedication to the “calling” of medicine can produce maladaptive behaviors as well. The phenomenon of presenteeism refers to the attendance of workers at the workplace when they are ill and not functioning optimally. In health care, it has been described in ill physicians whose attendance at the hospital caused an increased risk of infection in the patients they had been acculturated to try to help.
Substance Use and Abuse
Data previously suggested that 1% to 2% of practicing physicians are addicted to drugs, and up to 8% may be classified as alcoholics. However, the rates of substance abuse among physicians are not significantly different than in the general population, although anesthesiologists may be at higher risk for drug abuse than other physicians. Whereas the abuse of controlled substances and alcohol is obviously detrimental to job performance, a variety of other, ostensibly more innocuous drugs—such as caffeine, antihistamines, and nicotine—can also affect vigilance.
Small doses of caffeine, such as that found in a typical caffeinated soft drink, can have a positive effect on vigilance and task performance. Yet one study suggested that even among regular coffee drinkers, caffeine ingestion can magnify the physiologic consequences of stress. Antihistamines have been associated with performance decrements on simulated tasks, although nonsedating antihistamines may be without significant performance-degrading effects. Phenothiazines, and perhaps other antiemetics, also can impair performance on complex tasks.
It is well known that alcohol ingestion markedly impairs both vigilance and psychomotor performance. In fact, pilot simulator-based training studies have documented significant impairment in performance at blood alcohol levels as low as 20 to 35 mg/dL (0.02% to 0.035%), well below what would be considered legally drunk. Perhaps as important, the effects of a hangover from alcohol can also significantly affect performance, even in the absence of the perception of impairment by the affected individual. A study of performance in a laparoscopic simulator by both novices and experienced surgeons showed that hangover effects from the night before persisted until at least 4 pm the following day. The implication of these findings is that individuals should wait at least 14 hours after alcohol consumption before performing such complex tasks as flying an aircraft, performing surgery, or administering anesthesia.
Marijuana intoxication impairs performance, and use of the drug has been implicated as a causative factor in several railroad and airline accidents. In addition, like alcohol, marijuana intoxication is associated with a hangover condition that may impair performance, even after 24 hours and in the absence of an appreciation by the subjects of their impairment.
Personality
Subjects with different personality types will perform differently on various vigilance tasks. In fact, individual psychological or physiologic differences may be the most important confounding factors in the performance of vigilance tasks. For example, for some tasks, the incidence of error may be better predicted on the basis of individual personality traits, such as emotional stability, than on the nature of the particular task. Individual preferences for particular living, working, and sleeping schedules may have a substantial genetic contribution. Thus working or sleeping at times diametrically opposed to the individual’s biologically rooted personality characteristics leads to performance inefficiency and fatigue. Important factors in predicting adjustment to on-call duties may include the person’s adaptability to changes in normal sleeping schedule and the ability to overcome drowsiness.
The disruptive physician is a term recently applied to a subset of professionals whose behavior is at the extreme end of a normal distribution. As a result of fatigue, anxiety, stress, substance abuse, or any combination of the above, these physicians are at highest risk for patient complaints as well as medical liability claims. Early identification of these at-risk providers is increasingly important because many will respond favorably to counseling and behavior modification.
Training and Experience
Training and experience are clearly important to ensuring a high level of performance on complex tasks. Aviation accident rates directly correlate with flight experience. Individual practice patterns may significantly influence anesthetic morbidity, and one study demonstrated a clear inverse relationship between amount of surgical training and actual complication rates ( Fig. 23-5 ). Although physician experience may not linearly correlate with patient outcomes, additional training and experience may compensate for the negative effects on performance from stress or increased workload. Gaba and DeAnda showed that more experienced residents were better able to correct simulated untoward intraoperative events yet had no faster detection times than residents with 1 year less of training. However, individual differences, perhaps in experience or education, appeared to be much more important than amount of training. Weinger and colleagues showed that during routine anesthesia cases, novice residents exhibited higher workload and decreased vigilance and were less efficient than their more experienced counterparts. Although experience may mitigate some of the adverse effects of other performance-shaping factors, such as fatigue and boredom, it is by no means a complete remedy.

Interpersonal and Team Factors
The anesthesiologist is an integral member of the OR team. In other highly complex tasks involving teamwork, such as commercial aviation, the team has generally been together for a long time and is well practiced. Team communication involves unspoken expectations, traditions, assumptions regarding task distribution and chain-of-command hierarchies, and individual emotional and behavioral components. Alterations in any of these factors can impair effective team function, and unspoken assumptions about goals, duties, and roles may differ among the three main stakeholders in the OR environment. Failures in adequate communication among care providers may contribute significantly to the occurrence of clinical errors in the ICU. Not only were more than a third of all errors reported associated with presumably flawed verbal communication between nurse and physician, errors also seemed to occur more commonly following changes of nursing shifts, suggesting a contribution as well of faulty nurse-nurse communication. Familiar and constant composition of OR teams correlates positively with efficiency and quick turnovers. The added burden of teaching trainees contributes significantly to anesthesiologists’ workload and reduces responsiveness to events. These findings suggest that the anesthesiologist who is confronted with a new surgeon, OR nurse, or anesthesia resident should be sensitive to the “new interpersonal environment” and exercise extra vigilance by making a special effort to communicate clearly and unambiguously, particularly in stressful situations. Communication may prove even more difficult when some or all of the team members are subjected to other stressors, such as fatigue or sleep deprivation. In addition, overall OR team performance can be adversely affected by dysfunctional interpersonal interactions among team members.
Workload and Task Characteristics
The specific characteristics of the task itself will interact with other performance-shaping factors. An example of how workload or task requirements can influence performance comes from a study of 12 relatively inexperienced private pilots asked to perform a series of flight maneuvers on a simulator under increasingly difficult conditions until performance failure occurred. Under high-workload conditions, the subjects tended to decompose maneuvers into smaller, more manageable tasks. The subjects also omitted portions of the tasks that were not essential to maintain a minimum level of performance (i.e., safely flying the aircraft). Often the omission of a task component is unintentional, such as a lapse of memory during a routine but important procedure. Task omissions that could have dire consequences in real life become more common in sleep-deprived individuals.
In most complex monitoring tasks, increased task complexity generally impairs performance. Human senses can be particularly inaccurate, especially in dynamic situations. A major factor in the effect of an additional task on performance appears to be what personal resources (perceptual, cognitive, output modalities) are required for the new task and whether those resources are already taxed. Researchers have proposed a curvilinear relationship among performance, workload, and skill ( Fig. 23-6 ). This relationship appears to apply to the task of administering anesthesia.

In a study of pilot performance under various workload conditions, it was shown that with increasing workload, subjects tended to stare longer at the primary (i.e., more important) instruments. In addition to attending less frequently to their secondary instruments ( load shedding ), when pilots did gaze at these instruments, it was for a longer time. The presumption was that with increasing workload, the subjects required more processing time to perceive the information available from each instrument. The performance of more experienced pilots was less strongly influenced by workload. In a comparison of resident performance, Cao et al suggested that the same increased fixation shown by pilots is manifested by residents who were fatigued and relatively task-overloaded. Thus, as task complexity increases in a busy anesthetic case, this same phenomenon may be manifested in poor record keeping, sloppy anesthetic routine, or lapses of vigilance. This is supported by task-analysis studies. In addition, Lambert and Paget found that intraoperative teaching during “inappropriate” times markedly detracted from patient monitoring. Teaching as an independent risk factor was emphasized in one study showing a higher workload, using multiple measures, and a longer alarm response latency in teaching versus nonteaching cases.
Equipment and System Factors
The equipment the anesthesiologist encounters in the OR can be characterized in two ways: those devices primarily for the delivery of substances (gases, drugs, and fluids) and those that permit the clinician to monitor the outcome of substance delivery and the physiologic state of the patient. These two types of equipment increasingly share common attributes (e.g., microprocessor control) along with their associated problems. Although the percentage of anesthesia mishaps primarily as a result of equipment failures appears to be relatively small, the contribution of poor equipment design, maintenance, or performance to user error may be significant. Thus it seems appropriate to wonder about what hidden factors might have contributed to a catastrophe but were not elicited by the “accident” investigation. For example, did distracting alarms contribute to the end result? Or, more importantly, did poor equipment design more or less subtly influence the outcome? One study suggested that the use of transesophageal echocardiograpy during coronary artery bypass graft procedures may, under some circumstances, impair vigilance for other clinical events ( Fig. 23-7 ).

Poor Equipment Design
It has been suggested that almost all human error is due to either inadequate or inappropriate equipment or system design. Equipment problems can manifest in many arenas, including failures of design, device performance, user and service manuals, and maintenance. At least two factors make the design of OR equipment particularly difficult. First, the OR places appreciable physical and environmental stresses on equipment. Second, it is sometimes difficult to elicit from users precise or optimal design requirements for OR equipment. Block and colleagues described the installation of one of the first computerized monitoring systems specifically designed for the OR. Despite doing a careful survey of anesthesiologist users to determine their needs and preferences before designing the system, after the system was built, these users decided that they really wanted something different. This change may not reflect capriciousness as much as it does changing experience and evolving expectations. Nonetheless, it makes the designer’s task more difficult. At the very least, in the design and manufacture of new equipment, continuous direct contact between the designer and the user is essential.
Artifact remains a serious problem and can be a function of poor device design. For example, inadequate shielding of ECG cables can lead to motion artifact. If the software cannot handle this artifact, the displayed heart rate will either be absent, or worse yet, incorrect. Computing and displaying incorrect values can be disastrous; the clinician could easily withhold or institute therapy inappropriately or be unable to follow the course of therapy. Substantial improvement has been made in artifact management software over the years. For example, the incidence of pulse oximeter artifact has decreased considerably, such that it is no longer of significant concern to those who use automated anesthesia record-keeping devices.
Poor design can be particularly frustrating because it often is difficult to deal with after the fact. Poor design can be hardware or software related. The former may show up as disorganized, excessive, or inappropriate control mechanisms. Software-related design problems include confusing displays or problems navigating between display screens. Combined hardware and software problems include poor handling of artifact, disruptive display-control relationships, or irrational alarms.
Optimal design of complex microprocessor-based equipment requires a delicate balance between developing a device that is too complicated for the operator to understand versus one that becomes deceptively simple. If there are too many displays, or if the displays are confusing, performance may be suboptimal, and errors can result during crisis situations. The complexity of the Aegis radar display system (Lockheed Martin, Bethesda, MD), and some inherent design flaws, was a major cause of the accidental downing of a commercial airliner by the USS Vincennes . On the other hand, well-intended attempts to simplify a device can produce equally poor results. Cook and colleagues describe a humidifier that had been redesigned from a manual device to an automatic one. The clinicians liked the newer device because it was “simpler.” Yet research showed that the users did not understand the device’s underlying operation. For example, they did not know the procedure necessary to reactivate the device after an alarm, nor did they know that the heating elements were turned off after an alarm. To reset the device after an alarm, users simply turned it off and on again. This device was not intuitive in its design or functionality.
Each individual component of a system may be well thought out, but if the system design as a whole is faulty, the result will be unsatisfactory. Often the design is appropriate for one venue but is transferred to another without taking into account the unique attributes of the new environment. For example, most of the original “integrated” monitors were designed for use in the ICU and provided trend displays using a scale of 6 or more hours. For most anesthetic procedures, the resulting display was far too compressed. The needs of users in the OR can be substantially different than those in the ICU.
The ultimate performance of the equipment is the most important consideration. Poor performance may be related to faults with design, implementation, construction, inappropriate use, or inadequate supporting equipment. Mosenkis suggests that excellent design should allow clinicians to use a device correctly the first time they interact with it, preferably without reading the manual, whereas to use that device well, practice may be required. He goes on to assert that health care providers use medical devices as they do automobiles: they expect that a new device will work more or less the same as equivalent older devices. This cognitive model of how medical devices should work can only be supported through user-centered design and by standardization.
The use of simulation may facilitate many of the design goals required for safer and more user-friendly equipment. By testing several designs under almost-real clinical situations, manufacturers will be able to efficiently determine which design, if any, is the most appropriate. Simulation can also be used for training: the clinician will be able to safely and more quickly learn how to use a device, even under the most demanding conditions.
User Manuals/Documentation
The user theoretically depends on the manual for many purposes: becoming acquainted with a device during its initial use, learning its finer points, and troubleshooting. Unfortunately, many manuals and other documentation for medical devices are poorly written, confusing, or incomplete. All too often, the immediately essential or desired information is impossible to find. As a result of these shortcomings, most “user” manuals are simply not used by clinicians. From a practical standpoint, however, most people simply do not bother reading instruction manuals except perhaps to deal with a critical or particularly confusing condition; for example, if they cannot determine how to turn on the device.
On-line real-time help systems can now easily be incorporated into microprocessor-controlled medical devices. For example, an Internet connection can provide ready access to details needed for device operation, troubleshooting, maintenance, or repair. Current implementations typically include text, line drawings, and images. However, digital animations may be a more appropriate method to demonstrate how to calibrate, set up, or troubleshoot a device in real time.
Another consideration is education of anesthesiologists in the use of their equipment, and whether that education is the responsibility of the manufacturer, the hospital, the Food and Drug Administration (FDA), the clinicians themselves, or perhaps some professional society, such as the Anesthesia Patient Safety Foundation (APSF) or the Society for Technology in Anesthesia. Regardless, the technical education of anesthesia providers is sadly deficient at all stages, from the student to the experienced clinician. Until this education problem is addressed, the interaction between the user and complex machines will remain suboptimal, and equipment-induced or system-induced errors will occur that will be inappropriately blamed on the user. Recent efforts by the APSF and the Association for the Advancement of Medical Instrumentation to develop standardized processes for advanced equipment education for clinicians may bear fruit.
Equipment Maintenance
Anesthesia machines in particular require continual maintenance, which must be undertaken by experienced personnel. Maintenance training has traditionally been accomplished at the factory and usually requires several weeks. Optimally, maintenance should either be performed by a representative of the manufacturer or by a well-trained independent maintenance group (see also Chapter 32 , Chapter 33 ).
Maintenance errors often follow the same pattern as other types of errors: a chain of events, each one by itself insufficient to cause a disaster, contributes to the adverse outcome. In one of the most notorious maintenance errors, which occurred decades ago, the OR medical gas lines were switched. A hospital maintenance worker repaired the oxygen hose during the night so he would not disrupt the OR routine. What he initiated in good faith is a classic example of a chain of mishaps. First, an oxygen connector was attached to one end of each of two hoses, and a nitrous oxide connector was attached to the other end. Second, both hoses were black, rather than color coded; third, the maintenance worker carefully and neatly twisted the hoses around each other. In these days before the pulse oximeter, the first patient to be anesthetized with this machine died. The error was soon realized during the procedure on the second patient, whereupon the anesthesia machine was completely disconnected and the patient was ventilated with an independent, portable source of oxygen. The notoriety this case achieved was responsible both for an increased awareness of the possibility of OR gas switches and for the realization that continuous measurement of inspired oxygen is necessary. It has also inspired a generation of studies and simulations to train for proper responses to unexplained hypoxia. Sadly enough, gas switches still do occur, but hypoxic mixtures usually are detected by now-mandated oxygen analyzers. One notable exception is the case of a cylinder of carbon dioxide being used in place of a cylinder of nitrous oxide with attendant morbidity from hypercarbia.
Equipment Obsolescence
Anesthesia machines are designed to be long lasting and rugged, and manufacturers have largely accomplished this goal. Thus a large number of older machines are still in service, which presents a variety of problems: 1) integral parts wear out and do not function as intended, 2) components do not perform up to today’s standards, which can change relatively rapidly, 3) functions available on modern machines are absent, and 4) some components may be dangerous. Examples of dangerous components still found, albeit rarely, on older anesthesia machines in developing countries include the Copper Kettle (Puritan-Bennett; Covidien, Mansfield, MA) and in-circuit vaporizers and vaporizers without an interlock mechanism. Carbon dioxide (CO 2 ) absorber bypass mechanisms are also still in use in many countries, offering the ability to replace absorbent during a case but also allowing CO 2 accumulation if left on unintentionally. One report detailed the misconnection of an obsolete Bain circuit scavenging outlet to the fresh gas supply.
There will always be out-of-date machines, and it is not cost-effective to replace them all at once. Furthermore, some might argue that an experienced anesthesiologist is safer using customary equipment, instead of a technologically overwhelming “next-generation” workstation. On the other hand, when suddenly confronted with an old piece of equipment during an emergency anesthetic, a less experienced anesthesiologist trained only on modern equipment is placed in a difficult situation that is potentially life-threatening to the patient.
In this era of reduced health care resources and cost consciousness, issues arise when hospitals and anesthesia providers discuss the replacement of old anesthesia machines. Older anesthesia machines should be replaced if they do not meet American Society of Testing and Materials (ASTM) standards, are not easily upgradable, or have poor service records (see also Chapter 30 and Box 30-6 ).
Role of Standards in Anesthesia Equipment Design
For a number of years, a dedicated group of clinicians, device manufacturers, regulatory agencies, consultants, and other interested parties has been developing voluntary national and international medical device standards to help ensure safe medical practice. One example is the international effort to standardize small-bore connectors to reduce the risk of often-fatal misconnections of gas- and fluid-containing tubing. These standards also help ensure that the devices and their integration will be similar enough to each other to permit clinicians to use different devices from different manufacturers with minimal confusion. The American contributions to medical equipment standards development are sponsored by the nonprofit ASTM, the American National Standards Institute (ANSI), and the Association for the Advancement of Medical Instrumentation (AAMI). The international standards development process includes a joint working group between the International Organization for Standardization (ISO) and the International Electrotechnical Commission (IEC) on integrated monitors, anesthesia workstations, medical device alarms, and connectors.
Standards will continue to be a driving force in the integration of the anesthesia workstation, monitoring systems, and other medical equipment in the clinical work environment. The active involvement of anesthesiologists in the standards-making process is essential to ensure that it results in effective, safe, and easy-to-use devices (see also Chapter 34 ).
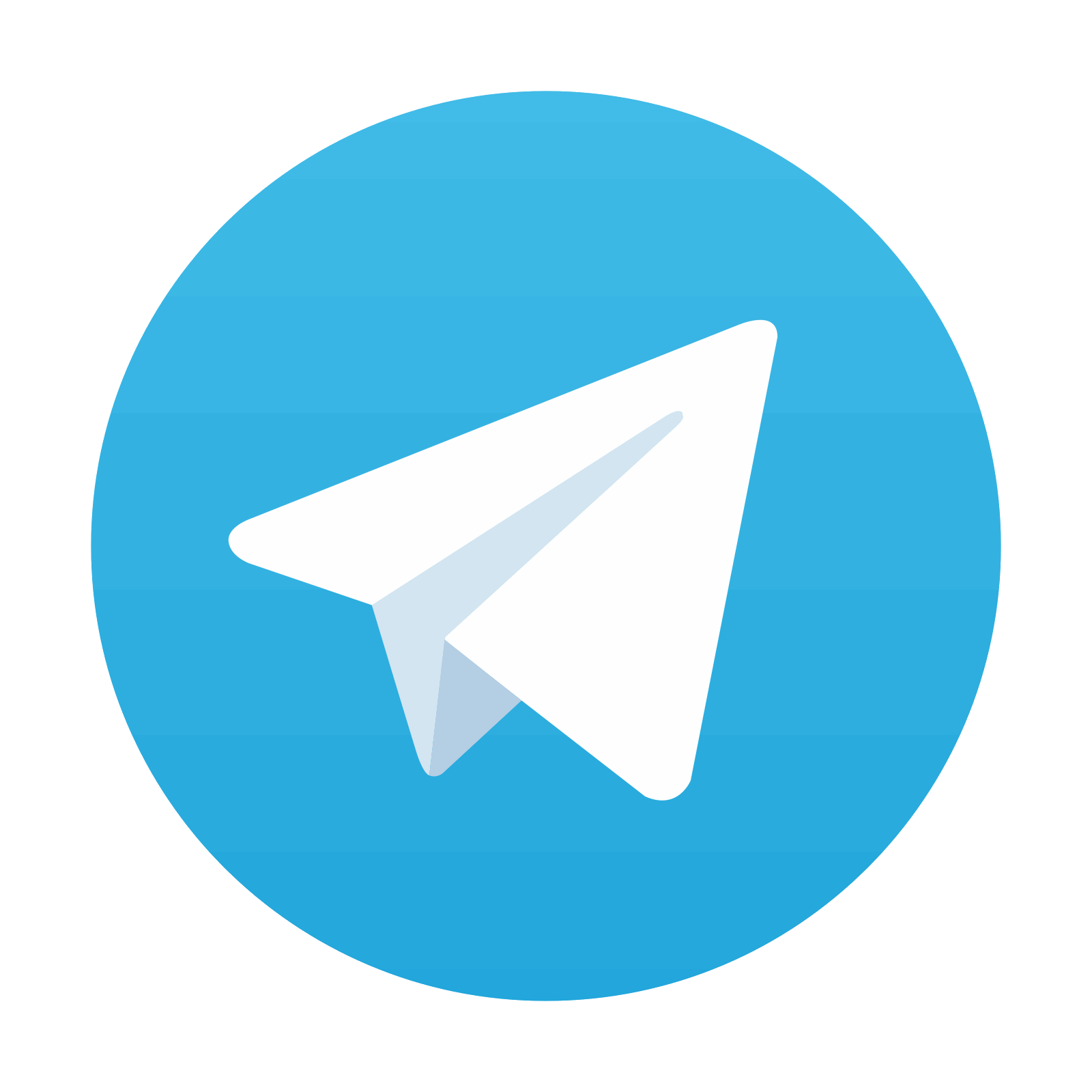
Stay updated, free articles. Join our Telegram channel

Full access? Get Clinical Tree
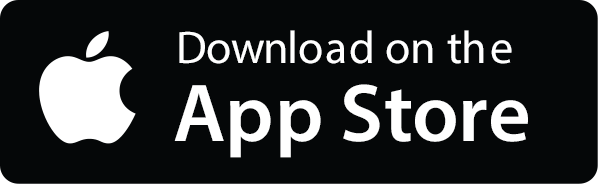
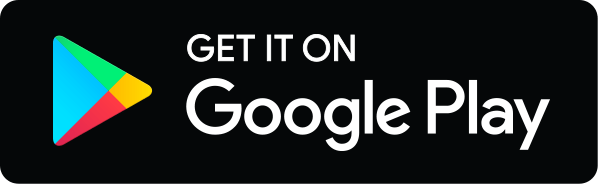