Humidification: Introduction
Humidification issues are overlooked by many clinicians in the intensive care unit (ICU). Because the need to heat and humidify inspired gases during mechanical ventilation is unanimously accepted, this process is considered the basic, supportive standard of care, about which there is no real debate. Yet, considerable controversy has surrounded central issues concerning humidification such as the level of adequate humidification and how to provide it, the influence of humidification devices on the incidence of ventilator-associated pneumonia, and certain patients and clinical situations and their requirements, such as the need for humidification during noninvasive ventilation. This may account for important differences in the practice of humidification between countries.1,2 Fortunately, renewed interest has emerged over the past decade, as indicated by several clinical studies that have helped settle some controversies. This chapter will review the reasons for conditioning inspired gases by recalling the normal process of heating and humidifying air during spontaneous breathing, the physical principles of humidification, and the consequences of inappropriate conditioning. Devices to achieve this conditioning are covered and their advantages and potential drawbacks discussed. Finally, practical guidelines are provided.
Rationale
As mentioned in the next section, the upper respiratory tract is responsible for most of the conditioning of the inspired gases. Important features of this conditioning (reviewed by Irlbeck3) include heat, humidification, and filtration, in order to deliver to the lower respiratory tract a warm (32°C [89.6°F]), humid (95% relative humidity), and pathogen-free and particle-free gas. The last step is achieved in the lower respiratory tract. During invasive mechanical ventilation, the endotracheal tube bypasses the upper respiratory tract. This places the burden of supplying heat and humidity to the cold and dry medical gases on the lower respiratory tract, a task for which it is poorly suited.4
Anatomy and Physiology of the Upper Airway
The upper respiratory tract (nose, mouth, nasopharynx, oropharynx, laryngopharynx, and larynx, but mainly the nose) is responsible for most of the conditioning of inspired gas. Anatomic structure and physiologic function of the nose are intimately linked. The highly vascular mucosa of the nose is ciliated and rich in mucosal glands and goblet cells. Three curved bony plates on the lateral side of each nasal cavity (the superior, middle, and inferior concha or turbinate bones), covered with a mucous membrane, ensure the important function of satisfactorily conditioning inspired gases. Their large surface area and position in relationship with the air current enable sufficient contact with the inspired gas. This mucous membrane also lines the paranasal sinuses, trachea, and bronchi but not the pharynx, which does not take part in the air-conditioning process.
Physical Principles of Humidification
Humidity can be defined as the moisture content of the atmosphere and by extension, water present as vapor in a gas mixture. Vaporization indicates the change of a liquid (or a solid) to a gas or vapor. There is no strict difference between the terms gas and vapor, although gas is generally used to describe a substance that appears in the gaseous state under standard conditions of pressure and temperature, and vapor to describe the gaseous state of a substance that appears ordinarily as a liquid or solid. Such a change of state requires a certain amount of energy or heat to overcome the van der Waals forces that bind the molecules together in the liquid state. They then escape the liquid as individual molecules of vapor. This amount of heat, specific for each substance, is known as the latent heat of vaporization of the substance.
Importantly, liquids can change to gases at temperatures below their boiling points. Vaporization of a liquid below its boiling point is called evaporation, and can occur at any temperature when the surface of a liquid is exposed in an unconfined space. Molecules of liquid that leave the surface of the liquid (because of sufficient kinetic energy) turn to vapor. They do so with their latent heat; the loss of heat causes the liquid’s temperature to decrease. These molecules of vapor exert a certain amount of pressure known as vapor pressure. Vapor pressure is substance and temperature specific (i.e., each substance has a specific vapor pressure for each given temperature). At its boiling point, the vapor pressure of a liquid is equal to the atmospheric pressure. Therefore, vapor pressure of water at 100°C (212°F) is 760 mm Hg.
Measurements of humidity include absolute humidity and relative humidity. Absolute humidity is the mass of water vapor per unit volume of the gas mixture and is measured in milligrams of water per liter of gas (mg H2O/L). Importantly, at any given temperature, a gas may contain only up to a certain amount of water vapor, corresponding to its maximal capacity at that temperature. Any extra water vapor will condense back into a liquid state. Relative humidity indicates the ratio of the actual water vapor content of the gas mixture to its total capacity at the given temperature. The amount of water vapor a gas can contain is directly proportional to the temperature of the gas (Fig. 51-1). For example, air with 50% relative humidity contains 22 mg of H2O at 37°C (98.6°F), but only 8.5 mg of H2O at 20°C (68°F).
Physiologic Principles of Humidification
Physiologic humidification delivers inspired air at 37°C (98.6°F) and saturated with water vapor (100% relative humidity) to the lungs. Figure 51-1 indicates that in these conditions, the gas mixture contains 44 mg of H2O per liter. The principle of humidification lies in the heat and humidity gradient that exists between the inspired gas and the respiratory tract.
As indicated above, humidification occurs first and mainly in the nose, then in the trachea and up to the second-generation bronchi. The large surface area of the turbinates, in combination with their irregular shape and protrusion into the nasal cavity that create a turbulent flow, enables a rapid transfer of heat from the mucosa to the inspired air. This heat gain is sufficient to allow water to evaporate from the mucosa into the inspired gas, enabling its humidification. As a result, inspired gas is warmed and humidified and nasal mucosal temperature decreases from 37°C (98.6°F) to 31°C (87.8°F).
During expiration, the opposite occurs. The gas leaves the alveolus at 37°C (98.6°F) with 44 mg/L of H2O, and transfers heat to the cooler upper airway. As its temperature decreases, so does its maximal water content capacity. Condensation on the mucosa then occurs. At the end of expiration, approximately one-third of the heat and humidity has been transferred back to the mucosa.
Until the inspired gas has reached 37°C (98.6°F) with 44 mg/L water content, heat and water are lost from the respiratory tract. The point at which inspired gas reaches core temperature and 100% relative humidity is called the isothermic saturation boundary. It is usually situated in the second-generation bronchi. After this point, temperature and humidity of inspired gas remain constant, to ensure adequate gas exchange in the alveolus. Depending on climatic conditions, the isothermic saturation boundary may move up or down the respiratory tract.
Pathophysiology of the Upper Airway
Underhumidification results from the bypassing of the upper airway that follows tracheal intubation and from the absence or inadequacy of conditioning of the dry, compressed, medical gas. Delivery to the lower respiratory tract of insufficiently warmed and humidified gas displaces the isothermic saturation boundary further down the bronchial tree. Moisture and heat loss ensues, inducing structural damages of the respiratory tract that may lead to physiologic and functional impairments with clinical repercussions.
Moisture loss from the respiratory tract is, under normal conditions, a physiologic phenomenon (approximately 150 mL water) and contributes to the body’s insensible water loss. The loss may be much greater if minute ventilation is increased, or if the gradient between the water content of inspired and expired air is large or both. This situation is encountered during mechanical ventilation and was first described during general anesthesia. In one of the first comprehensive studies on this topic, Burton described the reduction of mucociliary function after several hours of dry gas ventilation. When heat and humidity were added, mucociliary function was restored.5 Numerous studies have been performed subsequently and exhaustively reviewed.4 The adverse effects of inadequate humidification comprise structural damages such as loss of ciliary function, cilia destruction, damage to mucus glands, cytoplasmic and nuclear degeneration, cellular desquamation, mucosal ulceration, changes in tracheal cytology, and loss of surfactant. Such structural damage has functional repercussions: destruction of the mucociliary escalator, increase in sputum viscosity, increased airway resistance, decreased pulmonary compliance, reduced functional capacity, and increased pulmonary shunting. Clinical consequences include retained secretions, mucus plugging, atelectasis, increased work of breathing, hypothermia, and hypoxemia.3 In clinical practice, the worst and most feared adverse effect of underhumidification is life-threatening endotracheal tube occlusion, which requires urgent endotracheal tube replacement (see section on Effectiveness and Outcome).
During general anesthesia, the amount of heat loss through the respiratory tract is very small in comparison with that of other sources (e.g., the operative field and skin). Two mechanisms may account for this loss. Heat is transferred from the respiratory mucosa to the inspired gases, and the amount depends on the patient’s minute ventilation, the temperature gradient between the respiratory tract and the inspired gas, and the latter’s specific heat. Given the very low specific heat of air, minimal heat is required to raise its temperature. The second and major mechanism by which heat is lost is vaporization (change of liquid water of the respiratory mucosa into vapor and its transfer to the inspired gas). Given the high specific heat of water, a significant amount of heat is required to raise the relative humidity of inspired gases. The amount of heat is further increased when the relative humidity of the inspired gas is very low (i.e., dry medical gas). These considerations may be important during prolonged surgery in infants and young children, keeping in mind that skin, operative site, and fluid administration are the major contributors to the heat loss.
Because heat and moisture exchangers (HMEs) act passively (see below), they cannot deliver excessive heat or water to the respiratory tract. Consequently, the adverse effects described below are only possible with heated humidifiers and aerosol humidifiers and are now seldom encountered.6
The volume of water that can be added to the airway mucosa depends on the state it is in. With heating and humidifying devices, water is in a molecular form (vapor) and is therefore unlikely to result in overhumidification, given the amount of water vapor contained in inspired gases. To significantly increase this amount would require excessive heating of the inspired gas (way above body temperature), thus causing thermal injury before overhydration. Effects of excess water have been reported in the past with the use of aerosol humidifiers,7 but clinicians are now well aware of these side effects.
Reports of lung injury secondary to excessive heat are rare6 and are related to the misuse of heated humidifiers.8
Methods of Humidification
Although many different types of humidifiers have been developed in the past, clinicians mainly use two types of devices to condition inspired gas during mechanical ventilation: heated humidifiers and HMEs.
The general working principle of heated humidifiers is to heat water contained in a humidification chamber that humidifies inspired gas passing through it (Fig. 51-2).9 Heating the water enables evaporation to occur, and the gas leaves the chamber saturated with water vapor. Evaporation depends on the surface area over which it occurs, the temperature of the liquid water, and the magnitude of the vapor pressure above the water surface. It can therefore be increased by augmenting the contact of inspired gas and water (larger humidification chamber), by raising the temperature of the liquid water, and by increasing the mass flow of gas above the water surface so as to decrease the vapor pressure. Once the inspired gas has left the humidifier, it cools along the breathing circuit before reaching the patient. Thus, the temperature one wished to achieve at the patient’s level will not be reached. There are two ways to help overcome this problem. Inspired gas can be heated in the chamber to above the temperature one wishes to deliver to a patient, to take into account the heat loss across the circuit. Abundant condensation, however, will occur inside the tubing, providing an ideal reservoir for bacterial colonization10,11 and will need to be drained, exposing health care workers to contaminated fluids and placing other patients at risk of cross-contamination.12 To prevent this condensation (that results from the decrease in the temperature of the gas whose maximum vapor-carrying capacity is therefore reduced), breathing circuits can be heated by placing electric heater wires either in the wall or in the lumen of the circuit.
New devices are now servocontrolled by means of temperature sensors placed at the exit of the humidification chamber and just before the catheter mount (or flex tube). Because only the temperature is monitored (and not the humidity), however, these devices may become faulty in certain circumstances (see below).13,14
HMEs have undergone considerable development since their first description in the mid-1950s.15 The basic principle of all HMEs lies in their capacity to retain heat and moisture during expiration and deliver these to the incoming dry medical gas during subsequent inspiration (Fig. 51-3).16 This passive function has been achieved by different mechanisms. Metal elements of the first condensers were replaced by disposable foam, plastic, or paper. Increased moisture output was further achieved by coating the condenser element with a hygroscopic chemical (calcium or lithium chloride), which chemically adsorbs expired water vapor that is then returned to the inspired gas. These HMEs were called hygroscopic condensers. Derived from the field of filtration, hydrophobic HMEs were also used to heat and humidify inspired gas. The very large surface area of pleated, water-repellent ceramic of the first hydrophobic HMEs along with its low thermal conductivity enabled an important temperature gradient to develop within the HME that favored heat and moisture retention. Finally hygroscopic and hydrophobic elements were used in a single HME to create a “combined” HME. Hygrometric performance of these various HMEs differ considerably,17 with hydrophobic HMEs exhibiting the lowest humidity outputs (with the attendant risk of endotracheal tube occlusion) and combined HMEs the highest, although recent measurements indicate that some purely hygroscopic HMEs provide levels of absolute humidity comparable to those of combined HMEs.18,19 New devices have been designed to boost the humidifying performance of HMEs,20 although their actual clinical benefit is uncertain.
Figure 51-3
A combined (hydrophobic and hygroscopic) heat and moisture exchanger. Dark arrows indicate the presence of dripping wet condensation in the flexible tubing (the bright reflection seen on the wall of the tubing) suggesting that adequate humidity is being delivered by the heat and moisture exchanger (see text for details). Note that the heat and moisture exchanger is positioned vertically above the endotracheal tube, thus limiting the amount of secretions refluxing from the tube to the heat and moisture exchanger.
Application
Based on the above principles, supplying heat and humidity to inspired gases is mandatory when the upper airway is bypassed during tracheal intubation. This supply must be provided as soon as the patient is connected to the ventilator, either by an HME or by a heated humidifier. Apart from certain specific situations (see “Practical Steps”), both HMEs and heated humidifiers serve equally well. Despite this, practices differ considerably among countries.1,21 Rationalizing the choice of a given humidifying device should help decrease costs in mechanical ventilation without impeding quality of care.
Effectiveness and Outcome
Several important aspects must be taken into account when analyzing the effectiveness and outcomes of inspired gas conditioning, including:
- The avoidance of endotracheal tube occlusion (the worst and most feared complication of inadequate gas conditioning). This aspect is directly linked to the performance of the humidifying device in terms of heat and humidity delivery;
- The avoidance of spreading microorganisms (especially multidrug-resistant organisms). This is directly linked to the humidifying device’s capacity to prevent contamination of the respiratory tubing (Fig. 51-4 and Fig. 51-5);
- The addition of minimal resistance and dead space;
- The practicability of use of the device;
- The minimal maintenance necessary to ensure optimal use of the device; and
- The minimal cost associated with the purchase and the long-term use of the device.
Using this list, one can define the characteristics of the ideal humidifier: a device that provides adequate levels of humidification whatever the ventilator and patient conditions; one that does so automatically; one that is safe to operate (i.e., is electric-shock free, with no or a limited number of connections that can become faulty); one that protects the environment from the patient’s pathogens; one that is easy to use; and one that requires low maintenance and is inexpensive.
The adequate level of humidification can be defined as a level at which there is no excessive heat or water loss by the respiratory tract. The difficulty arises when one tries to set the minimum value of absolute humidity a device should deliver. This may be useful when selecting and comparing different devices. Although ranges as wide as 25 to 35 mg H2O/L have been suggested in the past,22 the figure of 30 mg H2O/L is recommended today.24 That is, a clinician wishing to select an appropriate humidifying device should make sure that the device delivers at least 30 mg H2O/L of absolute humidity. A recent study indicates that this output is not achieved by all available HMEs.23
In the past, HMEs and heated humidifiers were not equivalent in terms of humidity output. Back in the late 1980s, several studies alerted clinicians to the risk of endotracheal tube occlusions associated with the use of HMEs.24–27 These studies, however, all used purely hydrophobic HMEs, whose measured performance displayed low values of absolute humidity.17,18,28,29
Although heated humidifiers indisputably deliver greater values of absolute humidity than HMEs17,28,29 (Fig. 51-6), there is to date no evidence of any benefit of these greater values in terms of clinical outcome, including endotracheal tube occlusion. Table 51-1 displays the incidence of endotracheal tube occlusion reported in recently published studies. It is important to note that: (a) endotracheal tube occlusion rates have drastically dropped in comparison with earlier studies;25–27 (b) endotracheal tube occlusion is also reported with the use of heated humidifiers; and (c) the incidence of endotracheal tube occlusion no longer appears to be greater with HMEs than with heated humidifiers. In one study, the incidence was greater with heated humidifiers than with HMEs.30
Figure 51-6
Values of absolute humidity delivered by several heat and moisture exchangers and one heated humidifier. The dotted horizontal line delineates the minimum value of humidity (30 mg H2
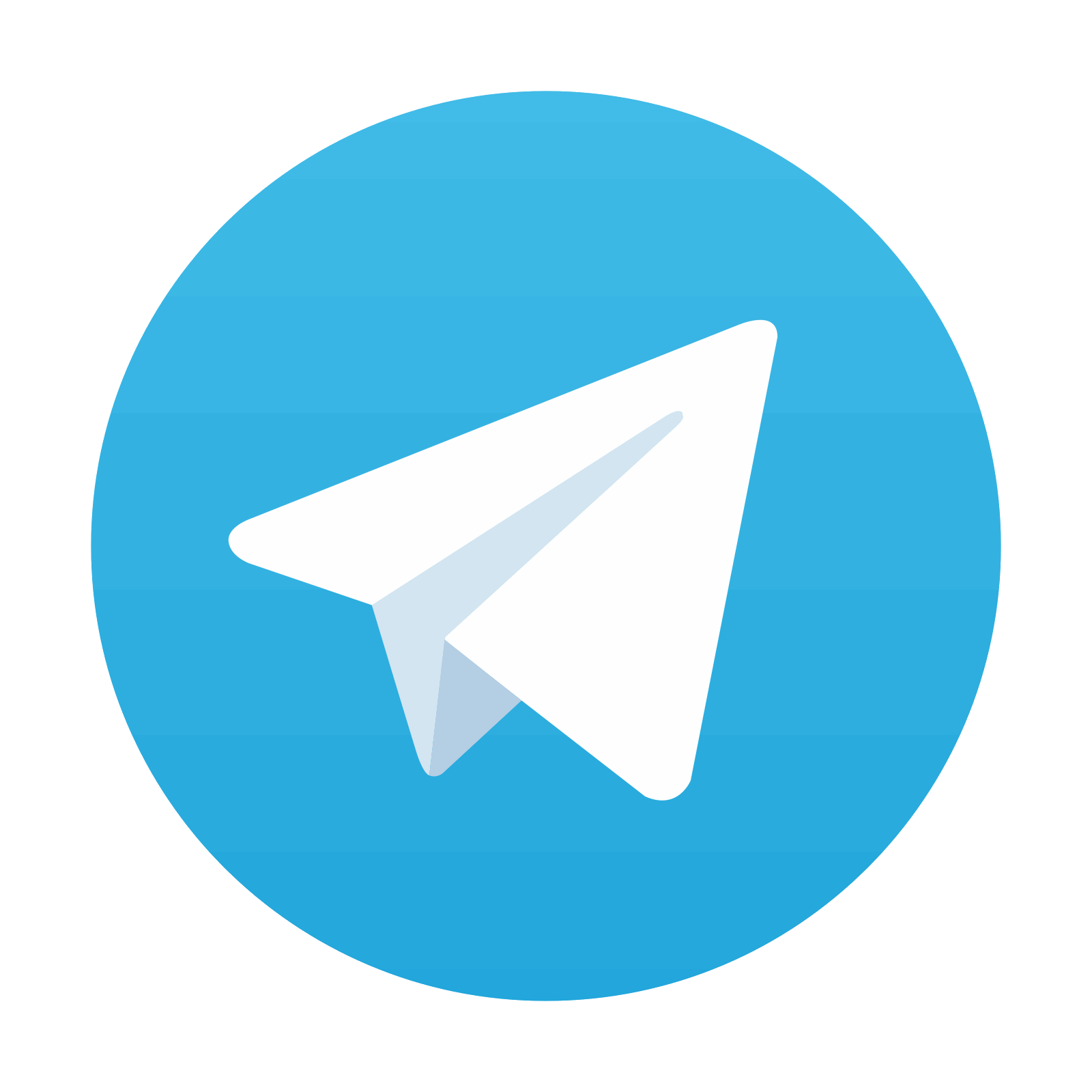
Stay updated, free articles. Join our Telegram channel

Full access? Get Clinical Tree
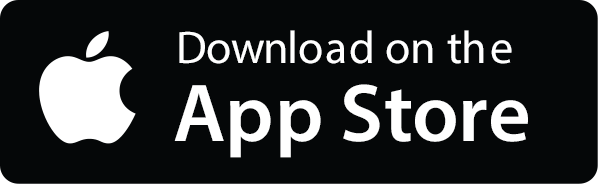
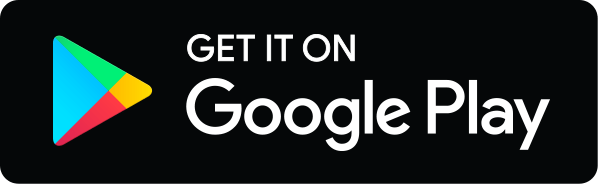
