Humans are in constant contact with xenobiotics. In addition to its critical role in absorbing nutrients, the gastrointestinal (GI) tract forms the initial functional barrier between ingested material and the body. An understanding of the structure, physiology, and innervation of the GI tract is critical to the toxicologic concepts of absorption, motility, and toxic insult. This chapter discusses the normal role of the GI tract and its relationship to toxicology. Anatomic, pathologic, and microbiologic principles are discussed, including the role of the GI tract in the metabolism of xenobiotics. Types of GI pathologies and their clinical manifestations are discussed, with examples when appropriate.
The luminal GI tract is divided into 5 distinct structures: oral cavity and hypopharynx, esophagus, stomach, small intestine, and colon (Fig. 18–1). These environments differ in luminal pH, epithelial cell receptor types, and endogenous flora. The transitional areas between these distinct organs have specialized epithelia and muscular sphincters with specific functions and vulnerabilities. Knowing the anatomy of these transition zones is particularly important for the localization and management of foreign bodies. The functions of the pancreas and liver are closely integrated with those of the luminal organs. The pancreas is discussed here; the liver and its metabolic functions are discussed in Chaps. 11 and 21.
The visceral structures of the GI tract are composed of several layers, including the epithelium, lamina propria, submucosa, muscularis layers, and serosa (the last of which is only present in intraperitoneal organs). As the transition is made throughout the GI tract, differences in luminal pH, epithelial cell receptors, muscularity, and endogenous flora are encountered, affecting the absorption and metabolism of individual xenobiotics.
The epithelium, the innermost layer of the GI tract, is the most specialized cell type in the intestine and is composed of epithelial, endocrine, and receptor cells. The basal surface of epithelial cells faces the lamina propria, and the apical surface faces the lumen. They are further specialized for specific functions of secretion or absorption. Additionally, the epithelial cells form part of the mucosal immune defense, where they detect the presence of microbial pathogens and downregulate the immune system in the presence of nonpathogenic or probiotic microbes. The major barrier to penetration of xenobiotics and microbes is the GI epithelium, a single-cell-thick membrane. The cell membrane is a semipermeable lipid bilayer that contains aqueous pores through which certain materials pass, depending on size and molecular structure. The membrane is not continuous because it consists of individual epithelial cells; however, these cells are joined to each other by structures known as tight junctions. The tight junctions have a gap of about 8 Å, which allows passage only of water, ions, and low-molecular-weight substances.
The muscle layer found beneath the lamina propria is made up of the muscularis mucosa, the circular muscles, and the longitudinal muscles. Contraction of the muscularis mucosa causes a change in the surface area of the gut lumen that alters secretion or absorption of nutrients. Whereas depolarization of circular muscle leads to contraction of a ring of smooth muscle and a decrease in the diameter of that segment of the GI tract, depolarization of longitudinal muscle leads to contraction in the longitudinal direction and a decrease in the length of that segment. The function of the muscle layers is integrated with the enteric nervous system to provide for a coordinated movement of luminal contents through the GI tract to maximize absorption and maintain the gastrointestinal microbiota. This integration facilitates the flow of chyme (undigested food) via a coordinated sequence of muscular contractions and relaxations, leading to segmentation of luminal contents, peristaltic movements, and unidirectional flow through the intestine.
The GI tract is innervated by the autonomic nervous system via both extrinsic and intrinsic pathways (Fig. 18–2). The extrinsic innervation permits communication among the brain, spinal cord, and chemoreceptors and mechanoreceptors located in the gut. Parasympathetic stimulation in the extrinsic pathway tends to be excitatory (“rest and digest”) and is carried via the vagus, splanchnic, and pelvic nerves to the myenteric and submucosal plexuses. In contrast, increased sympathetic tone (“fight or flight”) inhibits digestive and peristaltic activity via fibers that originate in the thoracolumbar cord and terminate in the myenteric and submucosal plexuses. The intrinsic innervation of the GI tract, or enteric nervous system, constitutes a reflex arc that modulates both peristalsis (via the myenteric plexus) and secretion (via the submucosal plexus) in response to parasympathetic and sympathetic tone.
An elaborate mucosal immune system has evolved to protect the GI tract from pathogens and modulate functional gastrointestinal diseases.35,44 Mucosal immunity consists of an afferent limb, which recognizes a pathogen and induces the proliferation and differentiation of immunocompetent cells, and an efferent limb, which coordinates and affects the immune response. The afferent system includes the lymphoid follicles and specialized M-cells found therein that promote transit of antigens to antigen-presenting cells.37 Once sensitized, immune cells undergo a complicated process of clonal expansion and differentiation, which occurs in mucosal and mesenteric lymphoid follicles, and in extraintestinal sites. Immunocompetent cells then return to the intestine and other mucous membranes and are scattered diffusely within the epithelial and lamina propria compartments.
The normal endogenous flora in the GI tract includes more than 400 species of bacteria along with a small number of fungi and viruses. The intestinal mucosa is normally colonized by large numbers of nonpathogenic anaerobic strains of Bacteroides spp, Eubacterium spp, Clostridium spp, as well as aerobic strains of Escherichia coli, Proteus spp, Enterobacter spp, Serratia spp, Lactobacillus spp, and Klebsiella spp.19 The concentration of luminal bacteria varies by site, from lowest in the proximal small intestine to highest in the large intestine. En masse, these bacteria are more metabolically active than the liver. Endogenous bacteria occupy unique niches related to host physiology, environmental pressures, and microbial interactions, which result in long-term stability. Following a course of ciprofloxacin, the gut microbiome of healthy volunteers demonstrated a marked shift and loss of biodiversity within days, but began to return to the initial state within a week of cessation.13 Indeed, the cumulative total of microbial species in the enteric system (or enterotype) is remarkably consistent across countries and continents.3
The endogenous flora has multiple metabolic functions. A primary function in the colon is the salvage of malabsorbed carbohydrates by fermentation and production of short-chain fatty acids, a preferred substrate for colonic epithelial cells. This increased metabolic efficiency is best demonstrated when mice grown in germ-free conditions require a higher caloric intake to maintain weight.48 This has led to some conjecture about the link between human flora and obesity.16
Bacterial metabolism significantly affects the disposition of enteral compounds.23 For example, the bacterial metabolism of digoxin contributes to its steady-state concentrations, and antibiotic treatment reduces or eradicate the intestinal flora, predisposing to digoxin toxicity.10 Bacterial contribution to vitamin K metabolism is also demonstrated, necessitating dose adjustments of warfarin during and after antibiotic therapy. The metabolic activity of intraluminal bacteria is exploited in some treatment strategies. For example, sulfasalazine, used in the treatment of ulcerative colitis, is created through the linkage of 5-aminosalicylic acid to sulfapyridine. The azo bonds of the nonabsorbable sulfasalazine are broken by bacterial azoreductases, permitting the absorption of active metabolites in the colon at the site of inflammation.41
The normal flora of the gut consists of probiotics—live, nonpathogenic bacteria and fungi that are also used as prophylactic or therapeutic xenobiotics. These bacteria compete for and displace pathogenic bacteria, directly modulate intestinal immune function, and exert a trophic effect on the GI tract. They appear to serve to decrease traveler’s diarrhea, suppress antibiotic-induced diarrhea, and reduce inflammation in ileal pouches after colectomy in patients with ulcerative colitis.17,20,22
There are 3 groups of regulatory peptide hormones that act on target cells within the GI tract. These are released from endocrine cells in the GI mucosa into the portal circulation and enter the systemic circulation to affect target cells. Gastrin, cholecystokinin (CCK), secretin, and glucose-dependent insulinotropic polypeptide (GIP; formerly gastric inhibitory peptide) are considered the primary GI hormones. Somatostatin and histamine comprise the paracrines, hormones that are released from endocrine cells and diffuse over short distances to act on target cells located in the GI tract. Vasoactive intestinal peptide (VIP), gastrin-releasing peptide (GRP), and enkephalins make up the last group known as neurocrines—substances that are synthesized in neurons of the GI tract, move by axonal transport down the axon, and are then released by action potentials in the nerves. The effects of these regulators are summarized in Table 18–1.7
Substance | Site of Secretion or Release | Stimulus for Secretion/Release | Actions |
---|---|---|---|
Endocrines | |||
Gastrin | G cells of gastric antrum | Small peptides and amino acids Stomach distention Vagal stimulation (via GRP) Inhibited by H+ in stomach (negative feedback) | ↑ Gastric H+ secretion ↑ Growth of gastric mucosa |
Cholecystokinin | I cells of duodenum and jejunum | Small peptides and amino acids Fatty acids and monoglycerides | ↑ Contraction of gallbladder and relaxation of sphincter of Oddi ↑ Pancreatic enzyme and HCO3− secretion ↑ Growth of exocrine pancreas and gallbladder ↓ Gastric emptying (allows more time for digestion and absorption) |
Secretin | S cells of duodenum | H+ in duodenal lumen Fatty acids in duodenal lumen | ↓ Small intestinal H+ ↑ Pancreatic HCO3– secretion ↑ Biliary HCO3− secretion ↓ Gastric H+ secretion ↑ Growth of exocrine pancreas |
GIP | K cells of small intestine | Fatty acids, amino acids, oral glucose | ↑ Insulin release ↓ H+ secretion by gastric parietal cells |
Motilin | Epithelial cells of duodenum | Periodic (not responsive to food) | ↑ Propulsion of smooth muscle |
Glucagonlike peptide 1 | L cells of small intestine | Meal intake | ↑ Insulin secretion ↓ GI motility |
Ghrelin | P/D1 cells of stomach | Fasting | ↑ Gastric emptying Stimulates feeding |
Paracrines | |||
Somatostatin | D cells throughout GI tract | H+ in GI tract lumen Inhibited by vagal stimulation | ↓ Release of all GI hormones ↓ Gastric H+ secretion ↓ Pancreatic secretions ↓ Splanchnic blood flow |
Histamine Nitric oxide | Mast cells of gastric mucosa Enteric nerves | Vagal stimulation gastrin Cellular inflammation | ↑ Gastric H+ secretion ↑ Epithelial secretion Vasodilation Smooth muscle relaxation |
Neurocrines | |||
VIP | GI tract neurons | H+ in duodenal lumen | Smooth muscle relaxation ↑ Pancreatic HCO3− secretion ↑ Fluid and electrolyte secretion from intestinal epithelium and bile duct cholangiocytes ↓ Gastric H+ secretion |
Gastrin-releasing peptide | Vagus nerves that innervate G cells | Vagal stimulation | ↑ Gastrin release from G cells |
Enkephalins (met-enkephalin, leu-enkephalin) | GI tract mucosa and smooth muscle neurons | ↑ Contraction of GI smooth muscle ↓ Intestinal secretion of fluid and electrolytes |
Xenobiotic effects on GI regulation have intended and unintended consequences. Somatostatins reduce splanchnic blood flow and portal blood pressure, leading to their use as therapeutics for bleeding esophageal varices. Erythromycin agonizes motilin receptors, leading to increased GI propulsion, cramping, and diarrhea.
The main functions of the mouth and oropharynx are chewing, lubrication of food with saliva, and swallowing. Saliva initiates digestion of starch by α-amylase (ptyalin), triglyceride digestion by lingual lipase, lubrication of ingested food by mucus, and protection of the mouth and esophagus by dilution and buffering of ingested foods.
Saliva production is unique in that it is increased by both parasympathetic and sympathetic activity. Parasympathetic stimulation, via cranial nerves VII and IX, acts on muscarinic cholinergic receptors on acinar and ductal cells, increasing saliva production via vasodilation and increasing transport processes. Parasympathetic pathways are stimulated by food in the mouth, smells, conditioned reflexes, and nausea, and are inhibited by sleep, dehydration, and fear. Sympathetic stimulation originates from preganglionic nerves in the thoracic segments T1 to T3. When β-adrenergic receptors are triggered by norepinephrine, production of saliva increases but at a rate less than that of parasympathetic stimulation.11 It is theorized that the hypersalivation, or sialorrhea, associated with clozapine is related to agonism of parasympathetic muscarinic receptors or antagonism of adrenergic α receptors (resulting in unopposed β-adrenergic receptor–mediated vasodilation).6 Conversely, dysfunction of saliva production, via the anticholinergic side effects of other antipsychotics, leads to dry mouth, or xerostomia.
The esophagus is a distensible muscular tube that extends from the epiglottis to the gastroesophageal junction. The lumen of the esophagus narrows at several points along its course, first at the cricopharyngeus muscle, then midway down alongside the aortic arch, and then distally where it crosses the diaphragm. The upper esophageal sphincter (UES) and lower esophageal sphincter (LES) are physiologic high-pressure regions that remain closed except during swallowing. Although the LES is a functional segment without anatomic features, the UES is marked by the presence of striated muscle.
The wall of the esophagus reflects the general structural organization of the GI tract noted previously, consisting of mucosa, submucosa, muscularis propria, and adventitia. The mucosal layer has 3 components. The nonkeratinizing stratified squamous epithelial layer faces the lumen; provides protection for underlying tissue; and houses several specialized cell types such as melanocytes, endocrine cells, dendritic cells, and lymphocytes. The lamina propria is the nonepithelialized portion of the mucosa, and the muscularis mucosa, a layer of longitudinally oriented smooth muscle bundles, is the third component.
The submucosa consists of loose connective tissue, and submucosal glands secrete a mucin-containing fluid via squamous epithelium–lined ducts, which facilitates lubrication of the esophageal lumen. The muscularis propria consists of an inner circular and outer longitudinal coat of smooth muscle; this layer also contains striated muscle fibers in the proximal esophagus that are responsible for voluntary swallowing.
The esophagus has no serosal lining. Only small segments of the intra-abdominal esophagus are covered by adventitia, a sheathlike structure that also surrounds the adjacent great vessels, tracheobronchial tree, and other structures of the mediastinum.
The esophagus provides a conduit for food and fluids from the pharynx to the stomach, and the sphincters generally prevent reflux of gastric contents into the esophagus. Normal transit of food involves coordinated motor activity, including a wave of peristaltic contraction, relaxation of the LES (facilitated by nitric oxide and VIP), and subsequent closure of the LES (facilitated by several hormones and neurotransmitters such as gastrin, acetylcholine, serotonin, and motilin). Xenobiotics alter muscle tone in various segments of the esophagus, altering function. Caffeine is associated with relaxation of the LES and decreased peristalsis, increasing incidence of acid reflux.34 Calcium channel blockers, nitroglycerin, β-adrenergic antagonists and phosphodiesterase inhibitors all relax esophageal smooth muscle, decreasing the pathologically elevated esophageal tone common to patients with achalasia.9 Because of the rapid transit time of swallowed substances xenobiotics through this portion of the GI tract, digestion does not take place, and passive diffusion of xenobiotics from the food into the bloodstream is prevented.11,59
The stomach is a saccular organ covered entirely by peritoneum that has a capacity greater than 3 L. The stomach is divided into 5 anatomic regions: the cardia, fundus, corpus or body, antrum, and pyloric sphincter. The gastric wall consists of mucosa, submucosa, muscularis propria, and serosa. The interior surface of the stomach is marked by coarse rugae, or longitudinal folds. The mucosa is composed of a superficial epithelial cell compartment and a deep glandular compartment. The mucous glands of the cardia, fundus, and body secrete mucus and pepsinogen. Oxyntic, or acid-forming, glands located in the fundus and body contain parietal, chief, and endocrine cells. The parietal cells contain vesicles that house hydrochloric acid–secreting proton pumps and secrete intrinsic factor, a substance necessary for the ileal absorption of vitamin B12. Chief cells secrete the proteolytic proenzyme pepsinogen, which is cleaved to its active form, pepsin, upon exposure to the low luminal gastric pH of 3 to 4. Pepsin is subsequently inactivated in the duodenum when the pH increases to 6.0. The endocrine, or enterochromaffinlike (ECL), cells found in the mucosa of the body of the stomach produce histamine, which increases acid production and decreases gastric pH by stimulating H2 receptors on the parietal cells. Somatostatin and endothelin, both modulators of acid production, are also produced in ECL cells (Fig. 49–4).
Hydrochloric acid is secreted when cephalic, gastric, and intestinal signals converge on the gastric parietal cells to activate proton pumps and release hydrochloric acid in an ATP-dependent process. During the cephalic phase, or the preparatory phase of the brain for eating and digestion, acetylcholine is released from vagal afferents in response to sight, smell, taste, and chewing. Acetylcholine stimulates the parietal cells via muscarinic receptors, resulting in an increase in cytosolic calcium and activation of the proton pump. Antral G cells of the stomach, produce and release gastrin in response to luminal amino acids and peptides. Gastrin activates receptors within parietal cells, leading to a similar increase in cytosolic calcium. Additionally, gastrin and vagal afferents induce the release of histamine from ECL cells, which stimulates parietal cell H2 receptors. Lastly, the intestinal phase is initiated when food containing digested protein enters the proximal small intestine and involves gastrin and several other polypeptides in the secretion of hydrochloric acid from the stomach59 (Fig. 18–3).
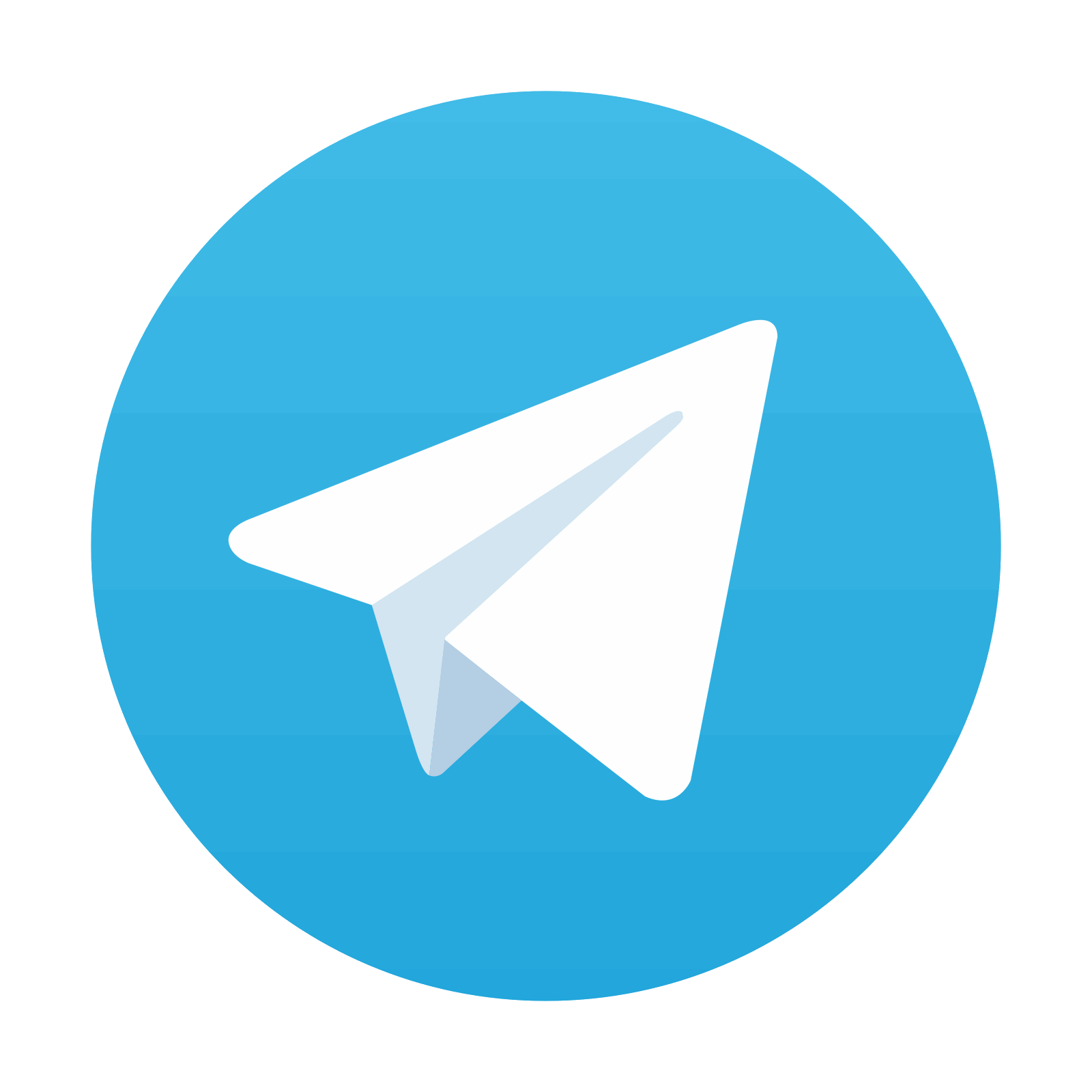
Stay updated, free articles. Join our Telegram channel

Full access? Get Clinical Tree
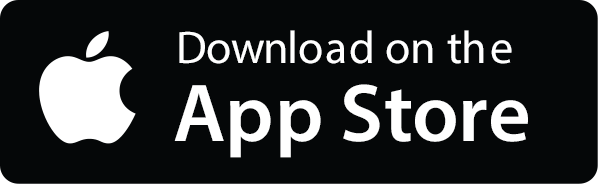
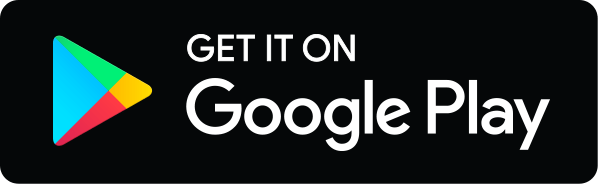