Fig. 57.1
Patient with respiratory paralysis in the 1952 polio epidemic being manually ventilated with a Waters CO2 absorption system
Logistics now became the problem. First, supplies of cuffed tracheostomy tubes, suction apparatus, anaesthetic breathing systems, and gas cylinders had to be obtained. Second, patients whose lungs required ventilating had to be concentrated in the hospital area containing the requisite resources. Third, trained teams of internists, surgeons, and anaesthetists had to provide skilled care continuously. Fortunately, the World Health Organisation had started an anaesthesia training centre in Copenhagen in 1950, and a number of these trainee anaesthetists were drafted to help supervise patient care. More help was needed: people to aspirate tracheal secretions, and manually compress the anaesthetic reservoir bags 24 hours a day for two to three months. Copenhagen medical students volunteered and soon organized their 6-hour shifts. Such care was a heavy responsibility for such untrained personnel: they not only had to maintain artificial ventilation, but also had to monitor the patient’s condition and alert doctors to problems.
At the peak of the epidemic, the lungs of 70 patients were simultaneously receiving manual ventilation. From 28 August to 3 September, 335 patients were admitted to Blegdam with polio’48 per day. By November, the number of medical students became insufficient, so Lassen drafted dental students too. In total, 1,500 students put in 165,000 hours ventilating patients’ lungs. Mortality in the severely affected patients decreased from about 80% to approximately 25%, a remarkable achievement.
A Defining Moment
The Copenhagen epidemic was a defining moment in the history of controlled ventilation. First, it demonstrated that airway control, secretion removal, and intermittent positive pressure ventilation could save the lives of patients in respiratory failure. Second, it stimulated development of mechanical ventilators. Third, it demonstrated the value of concentrating trained staff and essential facilities in one area, leading Ibsen to set up the world’s first ICU in his hospital in 1953 [3]. Fourth, it convinced Poul Astrup, who had performed blood tests during the epidemic, that new techniques of determining acid-base balance were needed [4]. Fifth, it demonstrated a need for new methods of safely transporting seriously ill patients. And sixth, it led to the creation of the speciality of intensive care medicine.
The Immediate Aftermath of the Epidemic
European doctors responsible for the care of polio patients visited Copenhagen to learn and apply the new techniques. On returning home, many of these physicians enrolled engineers to build mechanical ventilators. They began to treat patients with other causes of respiratory failure, including a 16 year-old girl admitted to the Radcliffe Infirmary in Oxford in August 1953. Initially diagnosed as having polio, she was treated in a tank ventilator but developed swallowing difficulties and chest complications. W Ritchie Russell and John Spalding, two Oxford neurologists who had visited Copenhagen, ordered a tracheostomy and mechanical ventilation using the prototype Radcliffe ventilator. Within a few days she was only able to communicate by small movements of her eyes. Several physicians questioned the ethics of keeping her alive. Not Russell and Spalding.
Fortunately, the paralysis was due to acute toxic polyneuritis (Guillain-Barré syndrome) and not to poliomyelitis. The patient recovered and was discharged from hospital 6 weeks later. She became a nurse, worked in the respiration unit, married a farmer and had four children. Kudos came to the doctors looking after her, and society benefited because her story advanced the adoption of this form of treatment [5].
Another disease in which IPPV proved to be life-saving was tetanus (lockjaw). Tetanus may cause severe, sometimes fatal, muscle spasms. In 1952, Woolmer and Cates in the UK, abolished the spasms in an afflicted patient, using succinylcholine to relax the muscles and manually ventilating the patient’s lungs for five days until the spasms wore off [6]. In 1954, Lassenet al in Copenhagen and Honeyet al in Oxford, reported survival of patients with severe tetanus who received curare and whose lungs were ventilated mechanically [7,8]. This technique also saved newborn babies with severe tetanus in Capetown, South Africa [9] and a randomized controlled trial in Durban showed that neonatal mortality could be reduced from more than 80%, to 40% [10,11].
In 1955, Viking Björk and Carl-Gunnar Engström reported the use of the Engström ventilator to support patients breathing inadequately after thoracic surgery [12]. Three years later one of the pioneers of Swedish anaesthesia, Olof Norlander, and colleagues working in other hospitals, described the use of ventilator support to treat patients with other causes of respiratory failure [13].
In 1956, a 51-year-old Chicago railway worker was crushed and rolled in an 8 inch (20 cm) space: “Like pie-dough under a rolling pin his body diameter was reduced to the size of this space”. The patient was in shock with multiple rib and other fractures, air in both pleural cavities, crush injuries to the liver and genitourinary tract, and gastrointestinal paralysis. Attempts were made to stabilise the chest by applying traction to the ribs, but only when Trier Mørch (recently emigrated to Chicago from Copenhagen) connected his piston ventilator to the cuffed tracheostomy tube, did the patient’s condition improve. Intermittent positive pressure kept the lungs inflated, providing an internal splint while the rib fractures healed. IPPV was continued for 30 days. The patient left hospital 51 days later, without apparent respiratory impairment. This incredible story revolutionised the treatment of crushed chest injuries [14]. The successful outcomes described above encouraged the development of ICUs with dedicated facilities and specially trained staff [15–17]. These early studies demonstrated successful treatments in patients with diverse types of respiratory failure, and resulted in the creation of numerous general and specialised ICUs over the next two decades.
Technical Developments After Copenhagen
The Airway
The standard metal tracheostomy tube used in Copenhagen was modified with an extension allowing connection to the breathing system, and an inflatable rubber cuff was applied over the lower end of the tube to provide an airtight seal with the trachea (Fig. 57.2). However, the tracheostomy tube and its modifications brought new problems. Bypass of the warming and moistening normally supplied by the nose, caused drying of secretions that became difficult to aspirate. The cuff tended to slip off the tube and traction from the breathing system pulled the tube from the trachea. These problems led to development of right-angled rubber, and later plastic, tubes with integral cuffs.
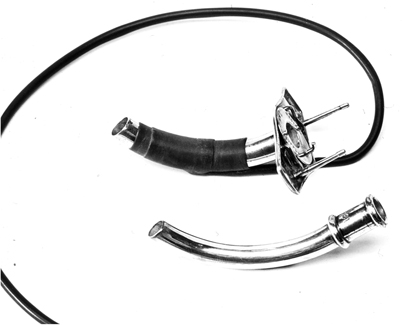
Fig. 57.2
Metal tracheostomy tube with detachable rubber cuff around outer tube and inner tube with extension to connect to ventilation system. Trier Mørch later used a similar tube but without a cuff. This enabled the patient to speak during inflation of the lungs
Humidification
Blockage of the tracheostomy tube from the drying of secretions prompted development of systems to warm and humidify the inspired gas. Marshall and Spalding in Oxford [18] examined possible methods and developed a simple hot water humidifier based on a biscuit tin with a thermostat and electric kettle element: this proved to be extremely effective but, since the inspired gas temperature varied with flow rate, it was necessary to monitor the inspired temperature close to the patient Y-piece. In 1971, a washing machine manufacturer in Auckland, New Zealand, produced the sophisticated Fisher and Paykel hot water humidifier that solved the problem [19]. Subsequently, heat and moisture exchangers were developed that could be connected to the tracheostomy tube and so function as an artificial nose.
Sterilization
In the 1960s, bacteria were found in the ventilator, humidifier and breathing systems. To overcome this problem, formaldehyde or ethylene oxide gas was recirculated around the ventilator circuit for 12 to 24 hours before use in a patient. The ventilator then had to be left running for another 24 hours to eliminate residual sterilizing agent. These problems initially forced manufacturers to design ventilators with autoclavable patient circuits (e.g. the Engström 300, and the Penlon Oxford ventilator). However, in the mid-1970s, improved low-volume bacterial filters that could be placed at the patient Y-piece became available, reducing the need for sterilization.
The AMBU Bag
In the hospital setting, manual ventilation with oxygen can normally be provided by a Waters circuit and expiratory valve with or without a CO2 absorber, but in 1956 there was a strike of gasoline truck drivers in Denmark that threatened hospital oxygen supplies. What was needed was a means of providing artificial ventilation with air. The anaesthetist, Henning Ruben, took up the challenge. Ruben had qualified as a dentist before the war, and utilised his talents as a ballroom dancer, champion fencer, magician and thought-reader to pay his way through college. In 1943, he had to escape from Copenhagen in a fishing boat, when the Nazi occupiers started to round up the Jews. The escapees lay under a false bottom in the fish hold and children were sedated with barbiturate suppositories to keep them quiet during the voyage to Sweden. It is said that Trier Mørch and a pharmacist friend developed a mixture of rabbit blood and cocaine that was sprinkled on the deck to abolish the sense of smell in the tracker dogs that searched the boats before departure to the fishing grounds. After the war, Ruben returned to Copenhagen, qualified in medicine and trained as an anaesthetist. He spent 6 months with Lucien Morris at the University of Iowa, and met James Elam. The two developed a lasting friendship and Ruben went on to demonstrate that neck extension, as practised in anaesthesia, provided a simple way of maintaining an airway in the unconscious patient.
Ruben developed numerous medical devices among which were a non-rebreathing valve and a portable suction apparatus. The latter was manufactured by the Danish company AMBU-TESTA, and it was this company that produced his next invention’a self-inflating bag. His first prototype consisted of a double-ended bag that was kept open by three bent bicycle wheel spokes, and was fitted with his non-rebreathing valve at one end and an air inlet valve at the other. Later, he filled the bag with lumps of foam rubber, the final version having a foam rubber lining to the bag (Fig. 57.3). Oxygen could be added to a reservoir attached to the air inlet. Many variants of this design have since been produced, and it has proved invaluable, not only in emergencies, but as a routine method of transporting patients with respiratory insufficiency [20].
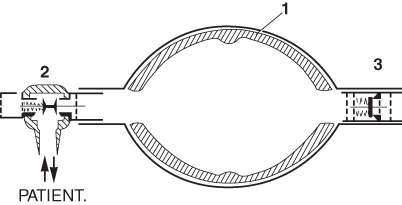
Fig. 57.3
The AMBU bag developed by Henning Ruben in 1956–1957.1 Foam lining.2 Ruben non-rebreathing valve.3 Inlet valve. Oxygen could be added to a reservoir attached to3. (Reproduced from Mushin WW et al. Automatic ventilation of the lungs, 3rd edition, 1980, p. 762, with permission from Wiley-Blackwell)
The Development of Mechanical Ventilators After the Epidemic
Space limitations preclude a comprehensive description of ventilator development after the Copenhagen epidemic. This section is restricted to significant developments in Scandinavia, the UK and the US, although similar developments took place in many European countries, and the British Commonwealth. Readers desiring more information should refer to standard works [21–24]. Here I summarize the evolution of ventilators from the simple mechanical pumps of the 1950s, to the sophisticated computer-controlled machines of today, and I describe how increasing knowledge of the physiology of mechanical ventilation and the pathophysiology of respiratory failure, influenced design considerations.
Many early ventilators were used in both the operating room and ICU. Those that utilised a non-rebreathing circuit, required a fresh gas flow equal to the patient’s minute volume [25]. Others were connected to a CO2 absorber circuit and so required a smaller flow of fresh gases. From the 1970s onwards, the introduction of microprocessor-controlled ventilators facilitated the development of new techniques of respiratory support in the ICU, but since these techniques were not needed in the operating room, anaesthesia ventilators remained relatively simple. Most anaesthesia ventilators now compress a bag or bellows in a rigid chamber connected to a standard CO2 absorption circuit, so that low fresh gas flows can be used. This minimises environmental pollution with anaesthetic vapours and gases, and cuts the cost of expensive agents. There has however, been a recent trend to use sophisticated piston type ventilators to cope with the ventilatory problems introduced by the insufflation of CO2 into the abdominal cavity and the extreme changes of body position required for laparoscopic surgery.
Ventilator Specifications
By 1950, it was known that that inflation of the lungs, whether by application of positive pressure to the airway or subatmospheric pressure to the chest wall, impeded venous return and so could impair cardiac output. It was also known that high inflation pressures could damage the lung. These findings suggested that: peak airway pressures should not exceed 25–30 cm H2O; the duration of inspiration should be less than the duration of expiration; and airway pressure should be zero during expiration. Machines were designed to produce a normal range of tidal volumes and breathing frequencies with an inspiratory:expiratory time ratio of 1:2, and most ventilators incorporated a pressure relief valve set to 30 cm H2O. These early machines ventilated normal lungs satisfactorily, but could fail to produce adequate ventilation when lung compliance decreased or airway resistance increased.
Because of the concern that the application of IPPV might decrease cardiac output, many early ventilators could also generate a subatmospheric pressure during expiration. However, it was soon found that a negative phase had little effect on the circulation and often impaired gas exchange, so cardiac output was optimized by infusing vasoconstrictor drugs or by increasing blood volume. Heart filling pressures were initially monitored by a central venous catheter but from the 1970s onwards, the Swan-Ganz (pulmonary artery) catheter was increasingly used in critically ill patients. Although this catheter enabled left heart filling pressures to be monitored, its use was associated with a number of complications and there is no firm evidence to indicate that it reduces mortality in severely ill patients [26]. Indeed, some authors suggest that the use of the catheter increases morbidity and mortality [27].
Until the late 1950s, PCO2 could only be measured by the tedious Van Slyke analysis and the use of rather crude pH electrodes. Thus, ventilators were usually set to provide a minute volume that was 20–30% more than the norms calculated from the Radford or Herzog ventilation nomograms[28,29]. These nomograms were designed for patients with normal lungs and it would be expected that patients with diseased lungs would require more ventilation. But how much more? Because of this uncertainty, patients with respiratory failure were hyperventilated until apnea occurred, apnea being the sign that ventilation was sufficient. Apnea also ensured that the patient did not fight the ventilator. If ventilatory drive still prevented synchronisation with the ventilator, apnea was produced by the administration of opioids or muscle relaxants. The introduction of the rebreathing technique for measurement of PCO2, the Astrup interpolation technique, and the development of O2 and CO2 electrodes in the late 1950s, revolutionised treatment and encouraged the attempt to produce normal blood gas values. In patients with severe lung disease, this often resulted in the use of high inflation pressures. Peak airway pressures increased further in the early 1970s, when it became known that the application of a positive end-expiratory pressure could improve gas exchange in the lung by preventing alveolar collapse during expiration. It soon became apparent however, that these high pressures (up to 70 cm H2O) produced lung damage, leading to the development of techniques designed to minimise such harmful effects. These will be discussed later.
Post-1952 European Ventilators
Several of the physicians visiting Copenhagen during and after the 1952 epidemic, recruited local engineers to build their own mechanical ventilators, to provide long term respiratory support. Many were primitive. For example, the Radcliffe ventilator, introduced in 1953, was based on the Oxford Inflating bellows developed in the Nuffield Department of Anaesthetics. Oxygen was added to air in a T-piece reservoir and the mixture sucked into a weighted bellows by a mechanism driven by an electric motor. The weights then forced the gas into the lungs through a pressure-operated non-rebreathing valve. The motors were powered by mains electricity or a 12-volt car battery, and 8 ventilation rates could be selected with a bicycle gear change and a hand-operated clutch. The latter allowed the ventilator to be powered by turning a gramophone handle in case of power failure. The non-rebreathing valve situated close to the patient sometimes failed when wet, and was later replaced by cam-operated valves in the machine. Since the Radcliffe ventilator was cheap, simple, robust and could be maintained by any bicycle mechanic, it was widely used in the UK and developing countries for over 30 years [30]. In 1962, the ventilator was modified so that it could be used both in the operating room and ICU. This flexibility resulted in its widespread use throughout the UK and Commonwealth [31]. The Radcliffe was classified as apressure generator since the weights on the bellows produced a constant pressure during inspiration; the tidal volume depended on the compliance (stiffness of the lungs), the airway resistance and the duration of inspiration. Since the lung characteristics could change rapidly it was essential to monitor the expired volume at regular intervals.
Other ventilators, often powered by compressed gases or an electrically driven piston or bellows, produced a fixed pattern of flow (flow generators). In these machines (e.g. Engström, Blease, Smith-Clarke), changes in lung compliance or airway resistance resulted in changes in airway pressure, though tidal volume was maintained. Since a knowledge of ventilator characteristics is essential for effective therapy, the Cardiff anaesthesia department physicist, WW Mapleson, utilised these and other major differences in functional characteristics (such as methods of initiating or controlling the duration of inspiration and expiration) to produce a classification of ventilator mechanisms that remains valid to this day [32]. In the course of his investigations, Mapleson found that many ventilators did not perform as predicted and over the next 20 years British and International Standards Organisations developed test lungs and procedures that revealed deficiencies in design and improved safety standards [33].
One man who did understand ventilator mechanics was Carl-Gunnar Engström, a doctor working in a Swedish infectious diseases hospital during the 1949 and 1950 polio epidemics [34]. He invented one of the world’s most successful mechanical ventilators (Fig. 57.4). With remarkable percipience, he recognised that patients died because of inadequate ventilation and designed an intermittent positive pressure ventilator that delivered breaths of controllable volume and frequency. This ventilator was used successfully in Copenhagen towards the end of the 1952 epidemic, and the Swedish government then ordered several machines, no doubt anticipating the Swedish polio epidemic in 1953, where they demonstrated their effectiveness. The machine proved lifesaving in other conditions including respiratory insufficiency due to skull trauma, brain tumour, and barbiturate intoxication. It was also adapted to provide controlled ventilation during anaesthesia with either a non-rebreathing or partially closed circuit. Though the precision Swedish engineering made this machine and its successors expensive, they were and are widely used throughout Europe and other parts of the world.
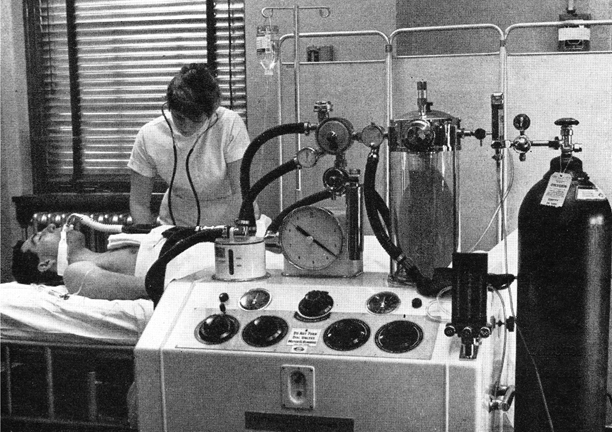
Fig. 57.4
Engström 150 ventilator in a Swedish Hospital. The warmed water humidifier is on the left and the gas meter recorded expired volume breath-by-breath. (From Safar P, Respiratory Therapy. by kind permission of the Publishers, F A Davis Company)
The British answer to this machine was the Smith-Clarke, designed by the ex-chief engineer of the Alvis motor company. Like the Rolls Royce car, the ventilator came with a mahogany box containing an oilcan, but the operator had to supply his own engine driver’s cap to fully enjoy oiling the cams. The Cape ventilator was the popular successor to this machine and incorporated an efficient, low-volume, hot-water humidifier. The Cape-Waine version of this ventilator was designed for use in anaesthesia with CO2 absorption [35].
In the UK, two other ventilator systems were commonly used during anaesthesia. In one, the ventilator was part of a non-rebreathing system, the fresh gas being led into a weight- or spring-loaded bellows and intermittently discharged into the patient. In the Howells and Manley ventilators the inspiratory and expiratory valves were pneumatically controlled whereas in the Barnet they were controlled by solenoids. These ventilators sat comfortably on the lower shelf of the ubiquitous Boyle’s anaesthetic machine, were relatively cheap, safe and simple to operate (Fig. 57.5). Some models could also be used in intensive care [36,37].
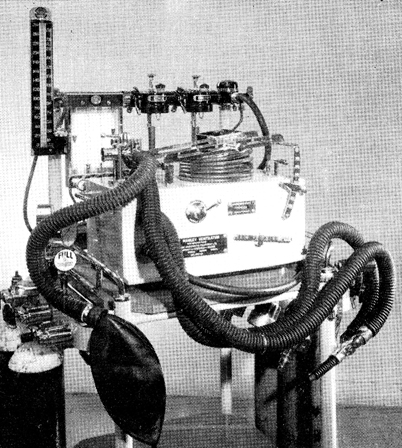
Fig. 57.5
The Manley ventilator that normally sat on the bottom shelf of the Boyle’s anaesthetic machine. (Courtesy of the author.)
The second type of ventilator system used during anaesthesia in the UK was based on the Bain (Mapleson D) breathing system. The anaesthetic gas mixture was delivered to the patient via a narrow bore tube situated concentrically within a light-weight 22 mm corrugated tube and the ventilator was attached to a fitting that replaced the Bain expiratory valve by means of a 1.5 m length of corrugated tube. This prevented dilution with the gases delivered by the ventilator. Interestingly, when tidal volume was set to 10 ml/kg body weight, CO2 levels could be controlled by adjusting the fresh gas flow, an inflow of 70 ml/kg body weight providing normocapnia [38,39]. An advantage of this system was that the delivery tubes could be lengthened so that the ventilator could be situated some distance from the patient (for example during magnetic resonance imaging), and it could be used with almost any ventilator. It was commonly used with the Pneupac, a simple, compact, fluid logic ventilator that was originally designed for resuscitation. There were four models, the one designed for anaesthesia having controls for flow rate and inspiratory and expiratory times [40]. This compact system was eventually displaced by the new generation of anaesthetic machines, fitted with integral ventilators and rapid response gas monitors that enabled low gas flows to be used safely, thus conserving expensive volatile agents and reducing pollution of the environment with anaesthetic gases and vapours.
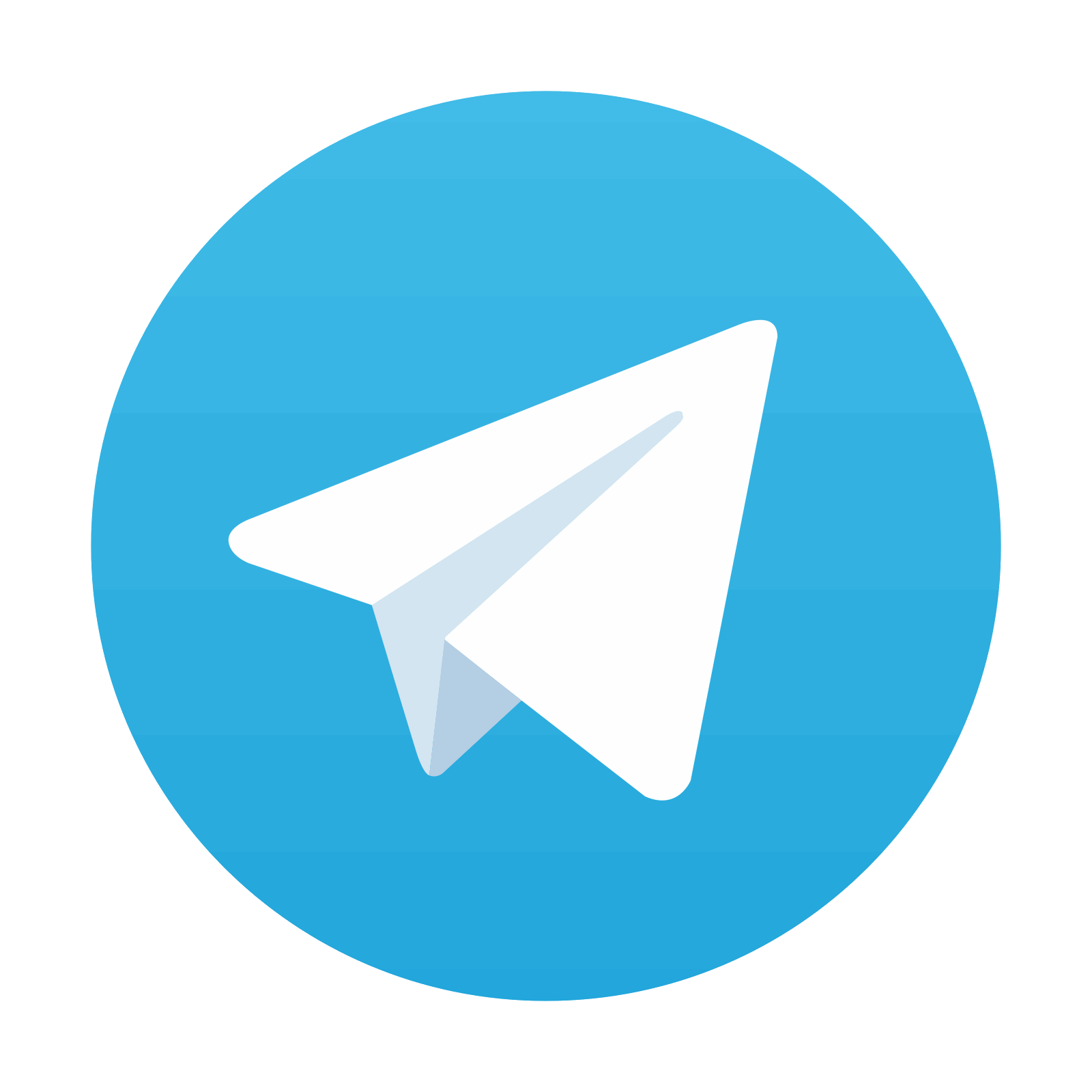
Stay updated, free articles. Join our Telegram channel

Full access? Get Clinical Tree
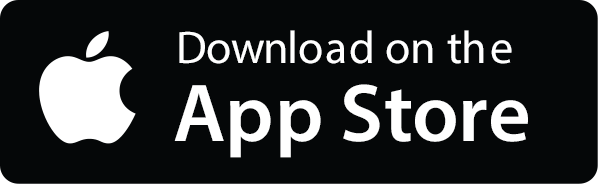
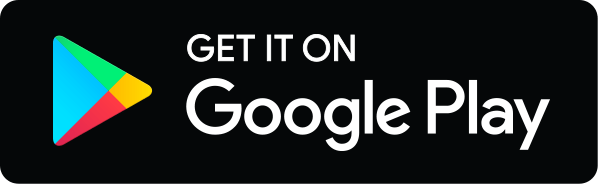