Epidural and Spinal Anesthesia
Christopher M. Bernards
Lucy S. Hostetter
Key Points








Related Matter
Dermatomes
Subarachnoid Block
Continuous Spinal
Hanging Drop
Paresthesias Spinal
![]() Figure 34-1. Posterior (A) and lateral (C) views of the human spinal column. Note the inset (B), which depicts the variability in vertebral level at which the spinal cord terminates. |
There are no absolute indications for spinal or epidural anesthesia. However, there are clinical situations in which patient preference, patient physiology, or the surgical procedure makes central neuraxial block the technique of choice. There is also evidence that these techniques may improve outcome in selected situations. Spinal and epidural anesthesia have been shown to blunt the “stress response” to surgery,1 to decrease intraoperative blood loss,2,3 to lower the incidence of postoperative thromboembolic events,2,3,4,5 and to decrease morbidity and mortality in high-risk surgical patients.6,7 In addition, both spinal and epidural techniques can be used to extend analgesia into the postoperative period, where their use has been shown to provide better analgesia than can be achieved with parenteral opioids.8 In addition, central neuraxial analgesia has become an indispensable technique to provide analgesia to nonsurgical patients. Thus, these techniques are an indispensable part of modern anesthetic practice, and every anesthesiologist should be adept at performing them.
Anatomy

Vertebrae
The spine consists of 33 vertebrae (7 cervical, 12 thoracic, 5 lumbar, 5 fused sacral, and 4 fused coccygeal; Fig. 34-1). With the exception of C1, the cervical, thoracic, and lumbar vertebrae consist of a body anteriorly, two pedicles that project posteriorly from the body, and two laminae that connect the pedicles (Fig. 34-2). These structures form the vertebral canal, which contains the spinal cord, spinal nerves, and epidural space. The laminae give rise to the transverse processes that project laterally and the spinous process that projects posteriorly. These bony projections serve as sites for muscle and ligament attachments. The pedicles contain a superior and inferior vertebral notch through which the spinal nerves exit the vertebral canal. The superior and inferior articular processes arise at the junction of the lamina and pedicles and form joints with the adjoining vertebrae. The first cervical vertebra (“atlas”) differs from this typical structure in that it does not have a body or a spinous process.
The five sacral vertebrae are fused together to form the wedge-shaped sacrum, which connects the spine with the iliac wings of the pelvis (Fig. 34-1). The fifth sacral vertebra is not fused posteriorly, giving rise to a variably shaped opening known as the sacral hiatus. Occasionally, other sacral vertebrae do not fuse posteriorly, giving rise to a much larger sacral hiatus. The sacral cornua
are bony prominences on either side of the hiatus and aid in identifying it. The sacral hiatus provides an opening into the sacral canal, which is the caudal termination of the epidural space. The four rudimentary coccygeal vertebrae are fused together to form the coccyx, a narrow triangular bone that abuts the sacral hiatus and can be helpful in identifying it. The tip of the coccyx can often be palpated in the proximal gluteal cleft, and by running one’s finger cephalad along its smooth surface, the sacral cornu can be identified as the first bony prominence encountered.
are bony prominences on either side of the hiatus and aid in identifying it. The sacral hiatus provides an opening into the sacral canal, which is the caudal termination of the epidural space. The four rudimentary coccygeal vertebrae are fused together to form the coccyx, a narrow triangular bone that abuts the sacral hiatus and can be helpful in identifying it. The tip of the coccyx can often be palpated in the proximal gluteal cleft, and by running one’s finger cephalad along its smooth surface, the sacral cornu can be identified as the first bony prominence encountered.
![]() Figure 34.2. Detail of the lumbar spinal column and epidural space. Note that the epidural veins are largely restricted to the anterior and lateral epidural space. |
Identifying individual vertebrae is important for correctly locating the desired interspace for epidural and spinal blockade. The C7 and T1 spinous processes are the most prominent and are easily palpated while running the hand down the back of the neck. The twelfth thoracic vertebra can be identified by palpating the twelfth rib and tracing it back to its attachment to T12. A line drawn between the iliac crests crosses the body of L5 or the L4 to L5 interspace.
Ligaments
The vertebral bodies are stabilized by five ligaments that increase in size between the cervical and lumbar vertebrae (Fig. 34-2). From the sacrum to T7, the supraspinous ligament runs between the tips of the spinous processes. Above T7, this ligament continues as the ligamentum nuchae and attaches to the occipital protuberance at the base of the skull. The interspinous ligament attaches between the spinous processes and blends posteriorly with the supraspinous ligament and anteriorly with the ligamentum flavum. The ligamentum flavum is a tough, wedge-shaped ligament composed of elastin. It consists of right and left portions that span adjacent vertebral laminae and fuse in the midline to varying degrees.7,8 The ligamentum flavum is thickest in the midline, measuring 3 to 5 mm at the L2 to L3 interspace of adults. The distance between the ligamentum flavum and the spinal meninges is also greater in the midline, measuring 4 to 6 mm at the L2 to L3 interspace.9 As a result, midline insertion of an epidural needle is least likely to result in unintended meningeal puncture. The anterior and posterior longitudinal ligaments run along the anterior and posterior surfaces of the vertebral bodies.
Epidural Space

Epidural Fat
The most ubiquitous material in the epidural space is fat, which is principally located in the posterior and the lateral epidural space (Fig. 34-3).10 Interestingly, the epidural fat appears to have clinically important effects on the pharmacology of epidurally and intrathecally administered drugs. For example, using a pig model, Bernards et al.12 showed that there is a linear relationship between an opioid’s lipid solubility and its terminal elimination half-time in the epidural space, its mean residence time in the epidural space, and its concentration in epidural fat. In addition, net transfer of opioid from the epidural space to the intrathecal space was greatest for the least lipid-soluble opioid (morphine) and least for highly lipid-soluble opioids (fentanyl, sufentanil). In effect, increasing lipid solubility resulted in opioid “sequestration” in epidural fat, thereby reducing the bioavailability of drug in the underlying subarachnoid space and spinal tissue.
Epidural fat also appears to play a role in the pharmacokinetics of epidurally administered local anesthetics. Specifically, sequestration in epidural fat likely explains why a highly lipid-soluble local anesthetic like etidocaine is only approximately equipotent with lidocaine in the epidural space despite the fact that etidocaine is roughly seven times more potent than lidocaine in vitro. Because of its much greater lipid solubility, etidocaine is more likely than lidocaine to be sequestered in epidural fat, thereby reducing the amount of drug available to produce block in the spinal nerve roots and spinal cord. Consistent with this hypothesis, Tucker and Mather13 showed that after administering 80 mg of etidocaine and 50 mg of lidocaine into the epidural space of sheep, the amount of etidocaine still present in epidural fat 12 hours later was more than 100 times greater than the amount of lidocaine. Thus, sequestration in epidural fat appears to play an important role in the pharmacokinetics of local anesthetics just as it does for epidural opioids.
Meninges
The spinal meninges consist of three protective membranes (dura mater, arachnoid mater, and pia mater), which are continuous with the cranial meninges (Fig. 34-4).
Dura Mater
The dura mater is the outermost and thickest meningeal tissue. The spinal dura mater begins at the foramen magnum where it fuses with the periosteum of the skull, forming the cephalad border of the epidural space. Caudally, the dura mater ends at approximately S2, where it fuses with the filum terminale. The dura mater extends laterally along the spinal nerve roots and becomes continuous with the connective tissue of the epineurium at approximately the level of the intervertebral foramina. The dura mater is composed of randomly arranged collagen fibers and elastin fibers arranged longitudinally and circumferentially.14 The dura mater is largely acellular except for a layer of cells that forms the border between the dura mater and the arachnoid mater. Despite the lack of cellular elements, the inner edge of the dura mater is highly vascular, which likely results in the dura mater being an important route of drug clearance from both the epidural space and the subarachnoid space.15
There is controversy regarding the existence and clinical significance of a dorsal midline connective tissue band, the plica mediana dorsalis, running from the dura mater to the ligamentum flavum. Anatomic studies using epiduroscopy and epidurography have demonstrated the presence of the plica mediana dorsalis and have led to speculation that this tissue band may on occasion be responsible for difficulty in inserting epidural catheters and for unilateral epidural block.16,17 However, using cryomicrotome sections to investigate the epidural space, Hogan10 failed to find evidence of a substantial connection between the dura mater and the ligamentum flavum. He speculated that the injection of either air or contrast required for the earlier studies may have compressed epidural contents (e.g., fat) and produced an artifact mimicking a connective tissue band. In addition, Hogan18 has shown in a clinical study that there is no significant impediment to spread of injectate across the midline. Thus, the plica mediana dorsalis does not appear to be clinically relevant with respect to clinical epidural anesthesia.
The inner surface of the dura mater abuts the arachnoid mater. There is a potential space between these two membranes called the subdural space (Fig. 34-4). Occasionally, a drug intended for either the epidural space or the subarachnoid space is injected into the subdural space.19 Subdural injection has been estimated to occur in 0.82% of intended epidural injections.20 The radiology literature suggests that the incidence of subdural injection during intended subarachnoid injection for myelography may be as high as 10%.21
Arachnoid Mater
The arachnoid mater is a delicate, avascular membrane composed of overlapping layers of flattened cells with connective tissue fibers running between the cellular layers. The arachnoid cells are interconnected by frequent tight junctions and occluding junctions. These specialized cellular connections likely account for the fact that the arachnoid mater is the principal anatomic barrier for drugs moving between the epidural space and the spinal cord.22
In the region where the spinal nerve roots traverse the dura and arachnoid membranes, the arachnoid mater herniates through the dura mater into the epidural space to form arachnoid granulations. As with the cranial arachnoid granulations, the spinal arachnoid granulations serve as a site for material in the subarachnoid space to exit the central nervous system (CNS). Although some have postulated that the arachnoid granulations are a preferred route for drugs to move from the epidural space to the spinal cord, the available experimental data suggest that this is not the case.23
The subarachnoid space lies between the arachnoid mater and the pia mater and contains the cerebrospinal fluid (CSF). The spinal CSF is in continuity with the cranial CSF and provides an avenue for drugs in the spinal CSF to reach the brain. In addition, the spinal nerve roots and rootlets run in the subarachnoid space.
Pia Mater
The spinal pia mater is adherent to the spinal cord and is composed of a thin layer of connective tissue cells interspersed with collagen. Trabeculae connect the pia mater with the arachnoid mater and the cells of these two meninges blend together along the trabeculae. Unlike the arachnoid mater, the pia mater is fenestrated in places so that the spinal cord is in direct communication with the subarachnoid space. The pia mater extends to the tip of the spinal cord where it becomes the filum terminale, which anchors the spinal cord to the sacrum. The pia mater also gives rise to the dentate ligaments, which are thin connective tissue bands extending from the side of the spinal cord through the arachnoid mater to the dura mater. These ligaments serve to suspend the spinal cord within the meninges.
Cerebrospinal Fluid
CSF is a complex solution made up of 99% water and containing an array of molecules including electrolytes, proteins, glucose,
neurotransmitters, neurotransmitter metabolites, cyclic nucleotides, amino acids, among many others. The CSF volume is approximately 100 to 160 mL in adult humans and it is produced at the rate of 20 to 25 mL/hr. Consequently, the entire CSF volume is replaced roughly every 6 hours.
neurotransmitters, neurotransmitter metabolites, cyclic nucleotides, amino acids, among many others. The CSF volume is approximately 100 to 160 mL in adult humans and it is produced at the rate of 20 to 25 mL/hr. Consequently, the entire CSF volume is replaced roughly every 6 hours.
According to the traditional view of CSF hydrodynamics, CSF is produced by plasma ultrafiltration in the choroid plexus within the cerebral ventricles. It then “flows” or “circulates” unidirectionally through the subarachnoid space to finally be absorbed by the arachnoid villi in the superior sagittal sinus and/or into lymphatics via perineural sheaths of cranial and spinal nerves. Several recent investigations, however, contradict this widely held view. Bulat et al.24 hypothesized that CSF is formed by water filtration across arterial capillary walls in the CNS. The filtration of water from arterial capillaries generates an osmotic counterpressure that drives water reabsorption into cerebral microvessels (i.e., venous capillaries and postcapillary venules). Using radiolabeled water, they demonstrated that CSF does not flow through subarachnoid spaces and that the bulk removal of CSF occurred primarily via absorption into cerebral microvessels. Klarica et al.25 further demonstrated that occlusion of the aqueduct of Sylvius does not alter isolated cerebral ventricle pressures, suggesting that the formation and absorption of CSF are in balance with one another.
In addition, the development of cine-magnetic resonance imaging and cine-computed tomography techniques have also shown that CSF does not circulate, rather it oscillates in the cephalocaudal axis with a frequency equal to the heart rate.26,27 CSF oscillates because cerebral expansion during systole displaces CSF caudally into the spinal canal and cerebral contraction during diastole causes the CSF displaced into the spinal canal to retreat back into the cranial vault. Net CSF movement is estimated at 0.04% per oscillation.
The clinical significance of this understanding of CSF motion is that CSF cannot be relied on to distribute drugs in the subarachnoid space. This is of little importance in single-shot spinal anesthesia because the kinetic energy of the injection and the baricity of the solution serve to distribute the drug. However, the lack of significant net CSF motion explains why drug distribution during the very slow infusions used for chronic intrathecal analgesia results in very limited drug distribution.28
Spinal Cord
In the first-trimester fetus, the spinal cord extends from the foramen magnum to the end of the sacrum. Thereafter, the vertebral column lengthens more than the spinal cord so that at birth the spinal cord ends at about the level of the third lumbar vertebra. In the adult, the caudad tip of the spinal cord typically lies at the level of the first lumbar vertebra. However, in 30% of individuals the spinal cord may end at T12, while in 10% it may extend to L3 (Fig. 34-1).29 A sacral spinal cord has been reported in an adult.29 Flexion of the vertebral column causes the tip of the spinal cord to move slightly cephalad.

just beyond the intervertebral foramen where they exit to join the sympathetic chain ganglia.
The spinal nerves and their corresponding cord segments are named for the intervertebral foramen through which they run. In the cervical region, the spinal nerves are named for the vertebra forming the caudad half of the intervertebral foramen; for example, C4 emerges through an intervertebral foramen formed by C3 and C4. In the thoracic and lumbar regions, the nerve roots are named for the vertebrae forming the cephalad half of the intervertebral foramen; for example, L4 emerges through an intervertebral foramen formed by L4 and L5. Since the spinal cord usually ends between L1 and L2, the thoracic, lumbar, and sacral nerve roots run increasingly longer distances in the subarachnoid space to get from their spinal cord segment of origin to the intervertebral foramen through which they exit. Those nerves that extend beyond the end of the spinal cord to their exit site are collectively known as the cauda equina (Fig. 34-1).
Technique
Spinal and epidural anesthesia should be performed only after appropriate monitors are applied and in a setting where equipment for airway management and resuscitation are immediately available. Before positioning the patient, all equipment for spinal block should be ready for use; for example, local anesthetics mixed and drawn up, needles uncapped, skin antiseptic solution available, and so on. Preparing all equipment ahead of time will minimize the time required to perform the block and thereby enhance patient comfort.
Needles
Spinal and epidural needles are classified by the design of their tips (Fig. 34-6). The Whitacre, Eldor, Marx, and Sprotte spinal needles have a “pencil-point” tip with one or two (Eldor) aperture(s) on the side of the shaft proximal to the tip. The Greene, Atraucan, and Quincke needles have beveled tips with cutting edges. The pencil-point needles require more force to insert than the bevel-tip needles but provide a better tactile “feel” of the various tissues encountered as the needle is inserted. In addition, the bevel has been shown to cause the needle to be deflected from the intended path as it passes through tissues while the pencil-point needles are not deflected.30 Epidural needles have a larger diameter than spinal needles to facilitate the injection of fluid or air when using the “loss of resistance” technique to identify the epidural space. In addition, the larger diameter allows for easier insertion of catheters into the epidural space. The Tuohy epidural needle has a curved tip to help control the direction that the catheter moves in the epidural space. The Hustead needle tip is also curved, although somewhat less than the Tuohy needle. The Crawford needle tip is straight, making it less suitable for catheter insertion.
The outside diameter of both epidural and spinal needles is used to determine their gauge. Larger gauge (i.e., smaller diameter) spinal needles are less likely to cause postdural puncture headaches (PDPH), but are more readily deflected than smaller gauge needles. Epidural needles are typically sized 16G to 19G and spinal needles 22G to 29G. Spinal needles smaller than 22G are often easier to insert if an introducer needle is used. The introducer is inserted into the interspinous ligament in the intended direction of the spinal needle and the spinal needle is then inserted through the shaft of the introducer. The introducer prevents the spinal needle from being deflected or bent as it passes through the interspinous ligament.30 Needles of the same outside diameter may have different inside diameters. This is important because inside diameter determines how large a catheter can be inserted through the needle and determines how rapidly CSF will appear at the needle hub during spinal needle insertion. All spinal and epidural needles come with a tight fitting stylet. The stylet prevents the needle from being plugged with skin or fat and, importantly, prevents dragging skin into the epidural or subarachnoid spaces, where the skin may grow and form dermoid tumors.
Sedation
If the patient desires, light sedation is appropriate before placement of spinal or epidural block. Generally, the patient should not be heavily sedated because successful spinal and epidural anesthesia requires patient participation to maintain good position, evaluate block height, and to enable communication with the anesthesiologist should a paresthesia occur when the needle contacts neural elements. In addition, patient cooperation is
required to properly evaluate an epidural test dose; sedation with as little as 1.5 mg of midazolam plus 75 μg of fentanyl has been shown to reduce the reliability of patient reports of subjective symptoms of intravenous (IV) local anesthetic injection.31 Once the block is placed and adequate block height assured, the patient can be sedated as deemed appropriate.
required to properly evaluate an epidural test dose; sedation with as little as 1.5 mg of midazolam plus 75 μg of fentanyl has been shown to reduce the reliability of patient reports of subjective symptoms of intravenous (IV) local anesthetic injection.31 Once the block is placed and adequate block height assured, the patient can be sedated as deemed appropriate.
Spinal Anesthesia
Position
Careful attention to patient positioning is critical to successful spinal puncture. Poor positioning can turn an otherwise easy spinal anesthetic into a challenge for both the anesthesiologist and the patient. Spinal needles are often inserted with the patient in the lateral decubitus position and this technique is described in detail later. However, both the prone jackknife and sitting positions offer advantages under specific circumstances. The sitting position is sometimes used in obese patients because it is often easier to identify the midline with the patient sitting. In addition, the sitting position allows one to restrict spinal block to the sacral dermatomes (saddle block) when using hyperbaric local anesthetic solutions. Spinal block is generally performed in the prone jackknife position only when this is the position to be used for surgery. The use of hypobaric local anesthetic solutions with the patient in the prone jackknife position produces sacral block for perirectal surgery.
In the lateral decubitus position, the patient lies with the operative side down when using hyperbaric local anesthetic solutions and with the operative side up when using hypobaric solutions, thus assuring that the earliest and most dense block occurs on the operative side. The back should be at the edge of the table so that the patient is within easy reach. The patient’s shoulders and hips are both positioned perpendicular to the bed to help prevent rotation of the spine. The knees are drawn to the chest, the neck is flexed, and the patient is instructed to actively curve the lumbar spine outward toward the anesthesiologist. This will spread the spinous processes apart and maximize the size of the interlaminar foramen. It is useful to have an assistant who can help the patient maintain this position. Using the iliac crests as a landmark (a line drawn between the iliac crests crosses the body of L5 or the L4 to L5 interspace), the L2 to L3, L3 to L4, and L4 to L5 interspaces are identified and the desired interspace chosen for needle insertion. Interspaces above L2 to L3 are avoided to decrease the risk of hitting the spinal cord with the needle. Some find it helpful to mark the spinous processes flanking the desired interspace with a skin marker. This obviates the need to reidentify the intended interspace after the patient is prepared and draped.
The skin is prepared with an appropriate antiseptic solution and draped. All antiseptic solutions are neurotoxic, and care must be taken not to contaminate spinal needles or local anesthetics with the antiseptic solution. Chlorhexidine–alcohol antiseptic prevents colonization of percutaneous catheters better than does 10% povidone–iodine. Consequently, the American Society of Regional Anesthesia currently recommends chlorhexidine for skin antisepsis prior to regional anesthesia procedures.a How one drapes is a matter of personal preference, but clear plastic fenestrated drapes offer the important advantage of permitting visualization of the entire back, which makes it easier to identify a rotated or inadequately flexed spine.
Midline Approach

The spinal needle or introducer needle is inserted in the middle of the interspace with a slight cephalad angulation of 10 to 15 degrees (Fig. 34-7). The needle is then advanced, in order, through the subcutaneous tissue, supraspinous ligament, interspinous ligament, ligamentum flavum, epidural space, dura mater, and finally arachnoid mater. The ligaments produce a characteristic “feel” as the needle is advanced through them, and the anesthesiologist should develop the ability to distinguish a needle that is advancing through the high-resistance ligaments from one that is advancing through lower-resistance paraspinous muscle. This will allow early detection and correction of needles that are not advancing in the midline. Penetration of the dura mater often produces a subtle “pop” that is most easily detected with the pencil-point needles. Detection of dural penetration will prevent inserting the needle all the way through the subarachnoid space and contacting the vertebral body. In addition, learning to detect dural penetration will allow one to insert the spinal needle quickly without having to stop every few millimeters and remove the stylet to look for CSF at the needle hub.
Once the needle tip is believed to be in the subarachnoid space, the stylet is removed to see if CSF appears at the needle hub. With small diameter needles (26G to 29G) this generally
requires 5 to 10 seconds, but may require ≥1 minute in some patients. Gentle aspiration may speed the appearance of CSF. If CSF does not appear, the needle orifice may be obstructed by a nerve root and rotating the needle 90 degrees may result in CSF flow. Alternatively, the needle orifice may not be completely in the subarachnoid space and advancing an additional 1 to 2 mm may result in brisk CSF flow. This is particularly true of pencil-point needles, which have their orifice on the side of the needle shaft proximal to the needle tip. Finally, failure to obtain CSF suggests that the needle orifice is not in the subarachnoid space and the needle should be reinserted.
requires 5 to 10 seconds, but may require ≥1 minute in some patients. Gentle aspiration may speed the appearance of CSF. If CSF does not appear, the needle orifice may be obstructed by a nerve root and rotating the needle 90 degrees may result in CSF flow. Alternatively, the needle orifice may not be completely in the subarachnoid space and advancing an additional 1 to 2 mm may result in brisk CSF flow. This is particularly true of pencil-point needles, which have their orifice on the side of the needle shaft proximal to the needle tip. Finally, failure to obtain CSF suggests that the needle orifice is not in the subarachnoid space and the needle should be reinserted.
If bone is encountered during needle insertion, the anesthesiologist must develop a reasoned, systematic approach to redirecting the needle. Simply withdrawing the needle and repeatedly reinserting it in different directions is not appropriate. When contacting bone, the depth should be immediately noted and the needle redirected slightly cephalad. If bone is again encountered at a greater depth, then the needle is most likely walking down the inferior spinous process and it should be redirected more cephalad until the subarachnoid space is reached. If bone is encountered again at a shallower depth, then the needle is most likely walking up the superior spinous process and it should be redirected more caudad. If bone is repeatedly encountered at the same depth, then the needle is likely off the midline and walking along the vertebral lamina (Fig. 34-7).
When redirecting a needle it is important to withdraw the tip into the subcutaneous tissue. If the tip remains embedded in one of the vertebral ligaments, attempts at redirecting the needle will simply bend the shaft and will not reliably change needle direction. When using an introducer needle, it also must be withdrawn into the subcutaneous tissue before being redirected. Changes in needle direction should be made in small increments because even small changes in needle angle at the skin may result in fairly large changes in position of the needle tip when it reaches the spinal meninges at a depth of 4 to 6 cm. Care should be exercised when gripping the needle to ensure that it does not bow. Insertion of a curved needle will cause it to veer off course.
If the patient experiences a paresthesia, it is important to determine whether the needle tip has encountered a nerve root in the epidural space or in the subarachnoid space. When the paresthesia occurs, immediately stop advancing the needle, remove the stylet, and look for CSF at the needle hub. The presence of CSF confirms that the needle encountered a cauda equina nerve root in the subarachnoid space and the needle tip is in good position. Given how tightly packed the cauda equina nerve roots are, it is surprising that all spinal punctures do not produce paresthesias. If CSF is not visible at the hub, then the paresthesia may have resulted from contact with a spinal nerve root traversing the epidural space. This is especially true if the paresthesia occurs in the dermatome corresponding to the nerve root that exits the vertebral canal at the same level that the spinal needle is inserted. In this case, the needle has most likely deviated from the midline and should be redirected toward the side opposite the paresthesia. Occasionally, pain experienced when the needle contacts bone may be misinterpreted by the patient as a paresthesia and the anesthesiologist should be alert to this possibility.
Once the needle is correctly inserted into the subarachnoid space, it is fixed in position and the syringe containing local anesthetic is attached. CSF is gently aspirated to confirm that the needle is still in the subarachnoid space and the local anesthetic slowly injected (≤0.5 mL/sec). After completing the injection, a small volume of CSF is again aspirated to confirm that the needle tip remained in the subarachnoid space while the local anesthetic was deposited. This CSF is then reinjected and the needle, syringe, and any introducer removed together as a unit. If the surgical procedure is to be performed in the supine position, the patient is helped onto his or her back. To prevent excessive cephalad spread of hyperbaric local anesthetic, care should be taken to ensure that the patient’s hips are not raised off the bed as they turn.
Once the block is placed, strict attention must be paid to the patient’s hemodynamic status with blood pressure and/or heart rate supported as necessary. Block height should also be assessed early by pin prick or temperature sensation. Temperature sensation is tested by wiping the skin with alcohol, and may be preferable to pin prick because it is not painful. If, after a few minutes, the block is not rising high enough or is rising too high, the table may be tilted as appropriate to influence further spread of hypobaric or hyperbaric local anesthetics.
Paramedian Approach
The paramedian approach to the epidural and subarachnoid spaces is useful in situations where the patient’s anatomy does not favor the midline approach, such as inability to flex the spine or heavily calcified interspinous ligaments. This approach can be used with the patient in any position and is probably the best approach for the patient in the prone jackknife position.
The spinous process forming the lower border of the desired interspace is identified. The needle is inserted approximately 1 cm lateral to this point and is directed toward the middle of the interspace by angling it approximately 45 degrees cephalad with just enough medial angulation (approximately 15 degrees) to compensate for the lateral insertion point. The first significant resistance encountered should be the ligamentum flavum. Bone encountered prior to the ligamentum flavum is usually the vertebral lamina of the cephalad vertebra and the needle should be redirected accordingly. An alternative method is to insert the needle perpendicular to the skin in all planes until the lamina is contacted. The needle is then walked off the superior edge of the lamina and into the subarachnoid space. The lamina provides a valuable landmark that facilitates correct needle placement; however, repeated needle contact with the periosteum can be painful.
Lumbosacral Approach
The lumbosacral (or Taylor) approach to the subarachnoid and epidural spaces is simply a paramedian approach directed at the L5 to S1 interspace, which is the largest interlaminar space. This approach may be useful when anatomic constraints make other approaches unfeasible. The patient may be positioned laterally, prone, or sitting, and the needle inserted at a point 1 cm medial and 1 cm inferior to the posterior superior iliac spine. The needle is angled cephalad 45 to 55 degrees and just medial enough to reach the midline at the level of the L5 spinous process. As with the paramedian approach, the interspinous ligament is bypassed and the first significant resistance felt should be the ligamentum flavum.
Continuous Spinal Anesthesia

The technique is similar to that described for “single-shot” spinal anesthesia except that a needle large enough to accommodate
the desired catheter must be used. After inserting the needle and obtaining free-flowing CSF, the catheter is simply threaded into the subarachnoid space a distance of 2 to 3 cm. It is often easier to insert the catheter if it is directed cephalad or caudad instead of laterally. If the catheter does not easily pass beyond the needle tip, rotating the needle 180 degrees may be helpful or another interspace may be used. Once the catheter is advanced beyond the needle tip, the catheter should not be withdrawn back into the needle shaft due to the risk of shearing the catheter off into the subarachnoid space.
the desired catheter must be used. After inserting the needle and obtaining free-flowing CSF, the catheter is simply threaded into the subarachnoid space a distance of 2 to 3 cm. It is often easier to insert the catheter if it is directed cephalad or caudad instead of laterally. If the catheter does not easily pass beyond the needle tip, rotating the needle 180 degrees may be helpful or another interspace may be used. Once the catheter is advanced beyond the needle tip, the catheter should not be withdrawn back into the needle shaft due to the risk of shearing the catheter off into the subarachnoid space.
A variety of catheters and needles are available for continuous spinal anesthesia. Commonly, 18G epidural needles and 20G catheters are used. However, needles and catheters this size carry a higher risk of PDPH, especially in young patients. Because of this risk, smaller needle and catheter combinations have been developed with catheters ranging in size from 24G to 32G. Although smaller catheters decrease the risk of PDPH, they have also been associated with multiple reports of neurologic injury, specifically, cauda equina syndrome (see “Complications”). For this reason, the United States Food and Drug Administration has advised against using any catheter smaller than 24G for continuous spinal anesthesia.
Epidural Anesthesia
For the novice, correct placement of an epidural needle can be technically more challenging than spinal needle placement because there is less room for error. However, with experience, epidural needle placement is often easier than spinal needle placement because the larger gauge needles used for epidural anesthesia are less likely to be deflected from their intended path and they produce much better tactile feel of the interspinous and flaval ligaments. In addition, the loss of resistance technique provides a much clearer end point when entering the epidural space than does the subtle “pop” of a spinal needle piercing the dura mater.
Patient preparation, positioning, monitors, and needle approaches for epidural anesthesia are the same as for spinal anesthesia. Unlike spinal anesthesia, epidural anesthesia may be performed at any intervertebral space. However, at vertebral levels above the termination of the spinal cord, the epidural needle may accidentally puncture the spinal meninges and damage the underlying spinal cord. To prevent accidental meningeal puncture, the anesthesiologist must learn to identify the interspinous ligaments and the ligamentum flavum by their feel. In addition, epidural needles must be advanced slowly and, most importantly, under control.
After proper positioning, sterile skin preparation, and draping, the desired interspace is identified and a local anesthetic skin wheal is raised at the point of needle insertion. Because epidural needles are relatively blunt, it is sometimes helpful to pierce the skin with a ≥18G hypodermic needle before inserting the epidural needle. For epidural anesthesia using the midline approach, the epidural needle is inserted through the subcutaneous tissue and into the interspinous ligament. The interspinous ligament has a characteristic “gritty” feel, much like inserting a needle into a bag of sand. This is especially true of younger patients. If the interspinous ligament is not clearly identified, then one should be suspicious that the needle is not in the midline. After engaging the interspinous ligament, the needle is advanced slowly through it until an increase in resistance is felt. This increased resistance represents the ligamentum flavum.

A glass syringe or a specially designed low-resistance plastic syringe is filled with 2 to 3 mL of saline and a small (0.1 to 0.3 mL) air bubble. The syringe is attached to the epidural needle and the plunger is pressed until the air bubble is visibly compressed. If the needle tip is properly embedded within the ligamentum flavum, it should be possible to compress the air bubble without injecting fluid. In this way the air bubble serves as a gauge of the appropriate amount of pressure to exert on the syringe plunger. If the air bubble cannot be compressed without injecting fluid, then the needle tip is most likely not in the ligamentum flavum. In this case, the needle tip may still be in the interspinous ligament, or it may be off the midline in the paraspinous muscles. To differentiate between these possibilities, one can carefully advance the needle and syringe a few millimeters in an effort to engage the ligamentum flavum. If it is still not possible to compress the air bubble, withdraw the needle into the subcutaneous tissue and reinsert it.
Once the ligamentum flavum is identified, the needle is slowly advanced with the nondominant hand while the dominant hand maintains constant pressure on the syringe plunger (Fig. 34-8). Do not advance the needle with the hand compressing the plunger because this does not allow for adequate control of needle movement. As the needle tip enters the epidural space, there will be a sudden and dramatic loss of resistance as the saline is rapidly injected. Saline injection into the epidural space can be moderately painful and patients should be forewarned. If the needle is advancing obliquely through the ligamentum flavum, it is possible to enter into the paraspinous muscles instead of the epidural space. In this case the loss of resistance will be less dramatic. To
help verify that the needle has entered the epidural space, 0.5 mL of air can be drawn into the syringe and injected. There will be virtually no resistance to air injection in the epidural space, while in the paraspinous muscles air injection will encounter demonstrable resistance.
help verify that the needle has entered the epidural space, 0.5 mL of air can be drawn into the syringe and injected. There will be virtually no resistance to air injection in the epidural space, while in the paraspinous muscles air injection will encounter demonstrable resistance.
After entering the epidural space, stop advancing the needle. Because the dura mater abuts the ligamentum flavum in many places, the dura may now be tented over the needle tip and advancing the needle any farther than necessary heightens the risk of accidental meningeal puncture, that is, “wet tap.” When the syringe is disconnected from the needle, it is common to have a small amount of fluid flow from the needle hub. This is usually the saline flowing back out of the epidural space but it could be CSF if the needle accidentally entered the subarachnoid space. CSF can often be distinguished by the fact that CSF will usually flow out in a volume greatly exceeding that used for the loss of resistance, CSF will be warm compared with saline, and CSF will test positive for glucose.
If a single-shot technique is to be used, then a local anesthetic test dose should be administered to help rule out undetected subarachnoid or IV needle placement. After a negative test dose, the desired volume of local anesthetic should be administered in small increments (e.g., 5 mL) at a rate of 0.5 to 1 mL/sec. Slow, incremental injection decreases the risk of pain during injection and allows detection of adverse reactions to accidental IV or subarachnoid placement before the entire dose is administered.
Continuous Epidural Anesthesia
Use of a catheter for epidural anesthesia affords much greater flexibility than the single-shot technique because the catheter can be used to prolong a block that is too short, to extend a block that is too low, or to provide postoperative analgesia. On the downside, catheters may migrate into an epidural vein, into the subarachnoid space, or out an intervertebral foramen. Catheter use is also more likely to result in unilateral epidural block, a clinical fact shown to result from catheter tips that end up in the anterior epidural space or migrate out an intervertebral foramina.18,34 An ever-changing selection of epidural catheters is commercially available. They differ in diameter, stiffness, location of injection holes, presence or absence of a stylet, construction material, and the like. Whichever catheter is chosen, it is important to verify that it passes easily through the epidural needle before the needle is placed in the epidural space. Epidural catheters are usually inserted through either Tuohy or Hustead needles because their curved tips help direct the catheter away from the dura mater. The needle bevel should be directed either cephalad or caudad, although the direction of the bevel does not guarantee that the catheter will travel in that direction. The catheter will typically encounter resistance as it reaches the curve at the tip of the needle, but steady pressure will usually result in passage into the epidural space. If the catheter will not pass beyond the needle tip, it is possible that the needle opening is not completely in the epidural space or that some structure in the epidural space is preventing catheter insertion (e.g., epidural fat). In this instance, the needle can be carefully advanced 1 to 2 mm more or rotated 180 degrees and the catheter reinserted. Although either of these maneuvers may result in successful catheter placement, they also increase the risk of accidental meningeal puncture. Alternatively, the procedure can be repeated at another interspace or with a different needle approach, for example, paramedian. Occasionally a catheter will advance only a short distance past the needle tip. This raises the possibility that the needle tip is not in the epidural space and needs to be repositioned. In this case, the catheter should not be withdrawn back into the epidural needle because of the risk that the catheter tip will be sheared off by the bevel’s sharp edge. Rather, the needle and catheter should be pulled out in tandem and the procedure repeated. An alternative explanation for the inability to thread an epidural catheter is that the tip of the epidural needle was bent during bony contact and now partially occludes the needle lumen.
The catheter should be advanced only 3 to 5 cm into the epidural space. Placing a longer length of catheter in the epidural space increases the risk that it will form a knot,35,36 enter an epidural vein, puncture the spinal meninges, exit an intervertebral foramen, wrap around a nerve root, or wind up in some other disadvantageous location. Once the catheter is appropriately positioned in the epidural space, the needle is slowly withdrawn with one hand as the catheter is stabilized with the other. After the needle is removed, the length of the catheter in the epidural space is confirmed by subtracting the distance between the skin and the epidural space from the length of the catheter below the skin. Documenting this distance is important when trying to determine if catheters used in the postoperative period have been dislodged.
An epidural test dose must be administered through the catheter to test for IV or subarachnoid placement before incrementally delivering the entire epidural drug dose. In addition, because of the risk of undetected IV or subarachnoid migration of the catheter over time, additional test doses must be administered before each top-up dose is given through the catheter. As with continuous spinal anesthesia, a reasonable guideline for top-up doses is to administer half the initial local anesthetic dose at an interval equal to two thirds the expected duration of the block.
Epidural Test Dose

The most common test dose is 3 mL of local anesthetic containing 5 μg/mL of epinephrine (1:200,000). The dose of local anesthetic should be sufficient that subarachnoid injection will result in clear evidence of spinal anesthesia. Intravenous injection of this dose of epinephrine typically produces an average 30 beats per minute heart rate increase between 20 and 40 seconds after injection.38,39 Heart rate increases may not be as evident in some patients taking beta-blocking drugs; reflex bradycardia often occurs in these patients.38,40 In beta-blocked patients, a systolic blood pressure increase of ≥20 mm Hg may be a more reliable indicator of IV injection.38,40
Importantly, the sensitivity of the standard 15-μg epinephrine test dose has been shown to be markedly diminished by pre-existing high thoracic epidural anesthesia and/or concurrent general anesthesia.41 Larger epinephrine doses may be effective at detecting IV injection in these settings, but that has not been shown experimentally.
Isoproterenol has also been used to detect intravascular injection.42 In addition, air injection combined with a precordial Doppler to detect the characteristic murmur has been used successfully to test for IV placement of epidural catheters.37 These techniques have been developed for the use in laboring women in whom the sensitivity of epinephrine as a test dose is disturbingly low because maternal heart rate increases during contractions are
often as large as those produced by epinephrine.43 The clinical indications for these alternative tests of IV injection await additional larger studies.
often as large as those produced by epinephrine.43 The clinical indications for these alternative tests of IV injection await additional larger studies.
Combined Spinal–Epidural Anesthesia
Combined spinal–epidural anesthesia (CSEA) is a useful technique by which a spinal block and an epidural catheter are placed simultaneously. This technique is popular because it combines the rapid onset, dense block of spinal anesthesia with the flexibility afforded by an epidural catheter. There are special epidural needles with a separate lumen to accommodate a spinal needle available for CSEA (Fig. 34-6). However, the technique is easily performed by first placing a standard epidural needle in the epidural space and then inserting an appropriately sized spinal needle through the shaft of the epidural needle and into the subarachnoid space. The desired local anesthetic is injected into the subarachnoid space, the spinal needle is removed, and a catheter placed in the epidural space via the epidural needle. The catheter can then be used to extend the height or duration of intraoperative block or can be used to provide postoperative epidural analgesia.
An interesting pharmacologic aspect of CSEA is the observation that after the peak spinal block height is established, both saline and local anesthetic injected into the epidural space are effective at pushing the block level higher.44,45,46 This observation has been interpreted to indicate that the mechanism by which the epidural “top-up” increases block height is by a volume effect (i.e., compression of the spinal meninges forcing CSF cephalad) as well as a local anesthetic effect.
A potential risk of this technique is that the meningeal hole made by the spinal needle may allow dangerously high concentrations of subsequently administered epidural drugs to reach the subarachnoid space. Anecdotal case reports and in vitro animal studies suggest that this may be a legitimate concern.43,47,48,49 Although CSEA is advantageous in some circumstances, additional prospective studies are necessary to identify the relative risks and limitations of the technique.
Pharmacology
Successful spinal or epidural anesthesia requires a block that is high enough to block sensation at the surgical site and last for the duration of the planned procedure. However, variability between patients is considerable (Figs. 34-9 and 34-10), reliably predicting the height and duration of central neuraxial block that will result from a particular local anesthetic dose is difficult. Thus, recommendations regarding local anesthetic choice and dose must be viewed as approximate guidelines. The clinician must understand the factors governing spinal and epidural block height and duration to individualize local anesthetic choice and dose for each patient and procedure.
Spinal Anesthesia
Block Height
Table 34-1 lists some common surgical procedures that are readily performed under spinal anesthesia and the block height that is usually sufficient to ensure patient comfort. Also listed are techniques that are appropriate to achieve the desired block height. The rationale for these recommendations is explained in the following section.
Table 34-1. Representative Surgical Procedures Appropriate for Spinal Anesthesia | ||||||||||||||||||||||||||||||||
---|---|---|---|---|---|---|---|---|---|---|---|---|---|---|---|---|---|---|---|---|---|---|---|---|---|---|---|---|---|---|---|---|
|
Baricity and Patient Position
The height of spinal block is thought to be determined by the cephalad spread of local anesthetic within the CSF. Table 34-2 lists some of the many variables that have been proposed to influence the spread of local anesthetics within the subarachnoid space. Many of these variables have been shown to be of negligible clinical importance. Of those factors that do exert significant influence on local anesthetic spread, the baricity of the local anesthetic solution relative to patient position is probably the most important. Baricity is defined as the ratio of the density (mass/volume) of the local anesthetic solution divided by the density of CSF, which averages 1.0003 ± 0.0003 g/mL at 37°C. Solutions that have the same density as CSF have a baricity of 1 and are termed isobaric. Solutions that are denser than CSF are termed hyperbaric, whereas solutions that are less dense than CSF are termed hypobaric.
Table 34-3 lists the baricity of local anesthetic solutions commonly used for spinal anesthesia. For practical purposes, solutions with a baricity <0.9990 can be expected to reliably behave hypobarically in all patients. Hypobaric solutions are typically prepared by mixing the local anesthetic solution with distilled water. Solutions with a baricity of ≥1.0015 can be expected to reliably behave hyperbarically. Hyperbaric solutions are typically prepared by mixing the local anesthetic in 5% to 8% dextrose. The baricity of the resultant solution depends on the amount of dextrose added; however, dextrose concentrations between 1.25% and 8% result in equivalent block heights.50,51 Lower dextrose concentrations have been shown to have a concentration-dependent effect on block height, with 0.33% producing a block to T9.5 on average, 0.83% producing a block to T7.2, and 8% producing a block to T3.6.52
Table 34-2. Factors that Have Been Suggested as Possible Determinants of Spread of Local Anesthetic Solutions within the Subarachnoid Space | ||
---|---|---|
|
Table 34-3. Baricity of Solutions Commonly Used for Spinal Anesthesia | ||||||||||||||||||||||||||||||||
---|---|---|---|---|---|---|---|---|---|---|---|---|---|---|---|---|---|---|---|---|---|---|---|---|---|---|---|---|---|---|---|---|
|
Baricity is important in determining local anesthetic spread and thus block height because gravity causes hyperbaric solutions to flow downward in CSF to the most dependent regions of the spinal column, whereas hypobaric solutions tend to rise in CSF. In contrast, gravity has no effect on the distribution of truly isobaric solutions. Thus, the anesthesiologist can exert considerable influence on block height by choice of anesthetic solution and proper patient positioning. Spinal block can be restricted to the sacral and low lumbar dermatomes (“saddle block”) by administering a hyperbaric local anesthetic solution with the patient in the sitting position53 or by administering a hypobaric solution with the patient in the prone jackknife position. Similarly, high thoracic to midcervical levels of anesthesia can be reached by administering hyperbaric solutions with the patient in the horizontal and Trendelenburg positions54,55 or by administering hypobaric solutions with the patient in a semisitting position. However, this use of hypobaric solutions is not recommended because the high block achieved and the diminished venous return associated with the upright posture can lead to significant cardiovascular compromise.
The sitting, Trendelenburg, and jackknife positions have marked influences on the distribution of hypobaric and hyperbaric solutions because these positions accentuate the effect of gravity. However, most spinal anesthetics are administered as hyperbaric solutions injected while patients are in the horizontal lateral position, after which they are turned to the horizontal supine position. In this situation the influence of gravity is more subtle because the dependent areas of the spinal column do not deviate as much from the horizontal. While the patient is turned laterally, gravity has a small but measurable effect on local anesthetic distribution in that hyperbaric solutions will produce a denser, longer-lasting block on the dependent side, while hypobaric solutions will have the opposite effect.56 This makes hypobaric solutions ideal for unilateral procedures performed in the lateral position when the operative side is nondependent (e.g., hip surgery). Hyperbaric solutions can be used to advantage for unilateral procedures performed in the supine position if the operative side is dependent during drug injection and the patient is left in the lateral position for at least 6 minutes.56 However, despite differences in block density and duration, peak block height will be comparable between the dependent and nondependent sides.
When the patient is turned supine following hyperbaric drug injection in the lateral position, the normal spinal curvature will influence subsequent movement of the injected solution. Hyperbaric solutions injected at the height of the lumbar lordosis will tend to flow cephalad to pool in the thoracic kyphosis and caudad to pool in the sacrum (Fig. 34-11). Pooling of hyperbaric local anesthetic solutions in the thoracic kyphosis has been evoked to explain the clinical observation that hyperbaric solutions tend to produce blocks with an average height in the midthoracic region (Fig. 34-9). In addition, hyperbaric solutions have also been observed to produce blocks with a bimodal distribution; that is, one group of patients with blocks centered in the low thoracic region and a second group of patients with blocks centered in the high thoracic region.57,58 The presumed explanation for this observation is that the lumbar lordosis produces “splitting” of the local anesthetic solution with some portion flowing caudad toward the sacrum and the remainder flowing cephalad into the thoracic kyphosis. The cephalad extent of the block then depends on what fraction of the injected drug flows cephalad. Consistent with this hypothesis is the fact that eliminating the lumbar lordosis by maintaining the hips flexed has been shown to significantly reduce58 or eliminate57 the bimodal distribution of blocks without affecting maximal block height.
Obviously, gravity influences the distribution of hyperbaric and hypobaric solutions only until they are sufficiently diluted in CSF so that they become isobaric. At this point, the local anesthetic solution no longer moves in response to changes in patient position and the block is said to be “fixed.” Interestingly, the time required for a local anesthetic solution to become fixed may be considerable. Povey et al.53,54 showed that hyperbaric bupivacaine
injected in the sitting position produces a saddle block that is restricted to the lumbar segments for as long as the subjects remained sitting. However, even 60 minutes after bupivacaine injection the block spread to midthoracic levels after turning the patients supine. Similarly, Bodily et al.59 found that hypobaric lidocaine administered in the jackknife position rose as many as six dermatomes when patients were allowed to sit upright in the recovery room as long as 60 minutes after lidocaine injection. Whether it is also possible to affect spread so long after injecting hyperbaric or hypobaric solutions in the horizontal position is unclear. Nonetheless, these findings demonstrate that in some situations it may be possible to exert influence on block height by adjusting patient position for at least 60 minutes after local anesthetic injection.
injected in the sitting position produces a saddle block that is restricted to the lumbar segments for as long as the subjects remained sitting. However, even 60 minutes after bupivacaine injection the block spread to midthoracic levels after turning the patients supine. Similarly, Bodily et al.59 found that hypobaric lidocaine administered in the jackknife position rose as many as six dermatomes when patients were allowed to sit upright in the recovery room as long as 60 minutes after lidocaine injection. Whether it is also possible to affect spread so long after injecting hyperbaric or hypobaric solutions in the horizontal position is unclear. Nonetheless, these findings demonstrate that in some situations it may be possible to exert influence on block height by adjusting patient position for at least 60 minutes after local anesthetic injection.
In contrast to the situation with hyperbaric solutions, patient position has no effect on the distribution of isobaric solutions because these solutions are not influenced by gravity. Consequently, isobaric solutions tend not to spread as far from the site of injection and produce blocks with an average height in the low thoracic region (Fig. 34-9).51,60 The obvious caveat is that the local anesthetic solution must be truly isobaric in the patient in whom it is used. Because of the variability in CSF density among patients, it is difficult to produce reliably isobaric local anesthetic solutions. Nonetheless, as indicated in Table 34-3, several local anesthetic solutions are used as if they were isobaric. It is noteworthy that while isobaric solutions produce an average block height that is lower than comparable hyperbaric solutions,51,60,61,62 the “isobaric” solutions produce blocks with a much greater variability in height.63,64,65 Logan et al.63 have termed plain bupivacaine “an unpredictable spinal anesthetic agent.” The greater variability in spread may stem in part from the fact that these solutions are actually slightly hypobaric and their spread has been shown to be affected by patient position.66,67 Temperature-related changes in baricity may also play a role in the variability in distribution of these nearly isobaric solutions. For example, Stienstra and van Poorten68 have shown that the distribution of plain bupivacaine is significantly altered by changes in temperature of the injected solution, with greater spread found with warmer injectate. In addition, McClure et al.69 have shown that increasing the volume and decreasing the concentration of isobaric tetracaine also increases the variability in block height. These and other unknown factors may play a role in the unpredictability of these nearly isobaric solutions. Although unpredictability is cause for concern, it should be pointed out that the lower average block height achieved offers potential advantages for surgical procedures below the umbilicus because of the decreased incidence of cardiovascular side effects associated with lower blocks. The isobaric solution that has been shown to most reliably produce a low thoracic block is 10 mg of tetracaine crystals diluted in 1- or 2-mL room temperature saline and injected in the horizontal position.69
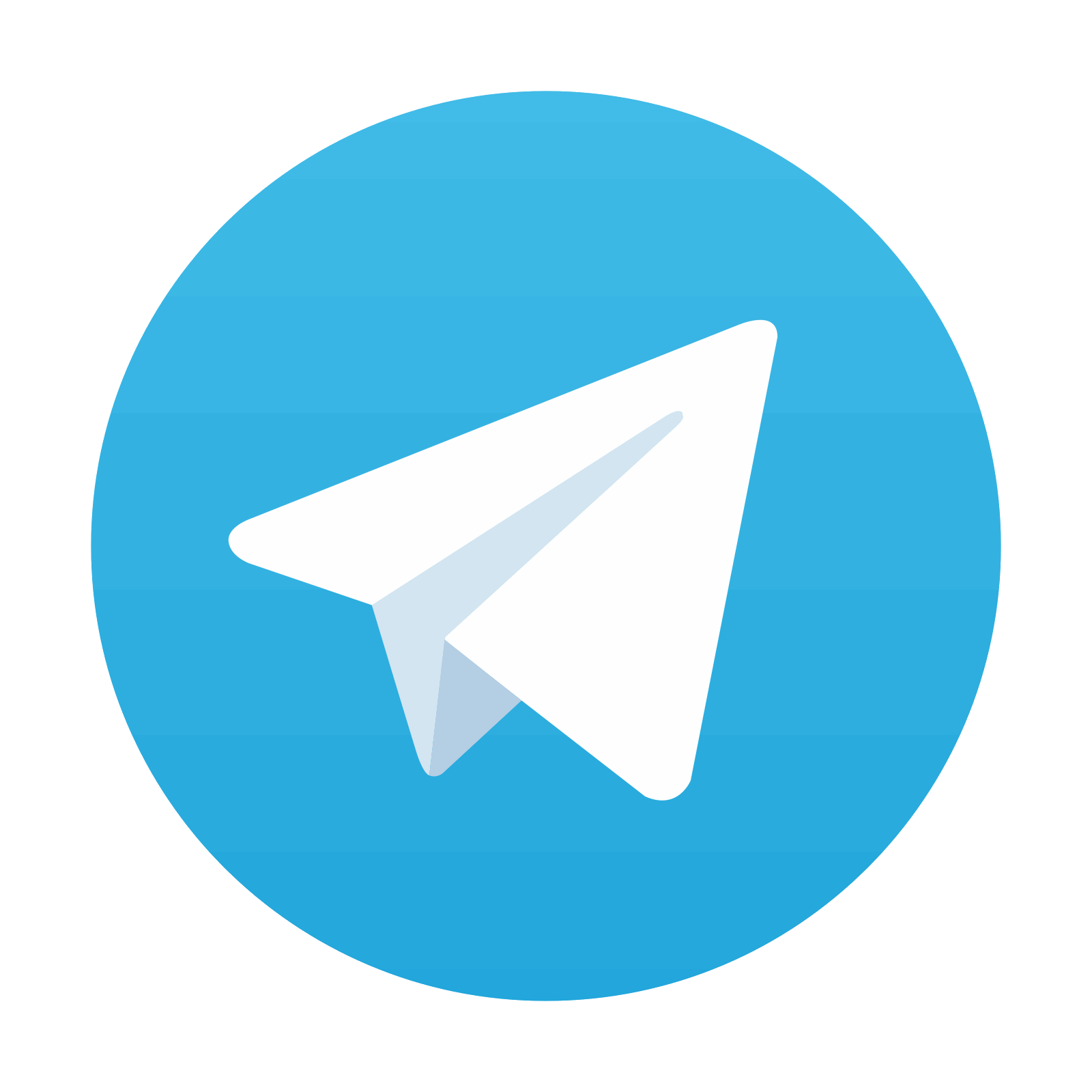
Stay updated, free articles. Join our Telegram channel

Full access? Get Clinical Tree
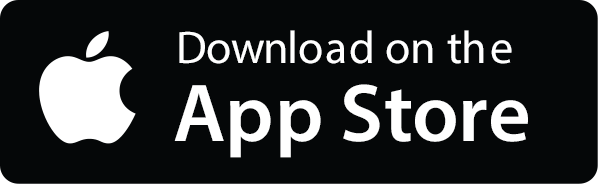
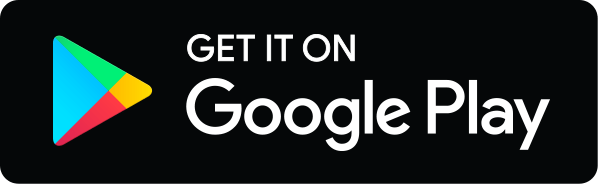
