Reports
Reports of AAGA had to be of procedures in which the patient expected to be unconscious
First reports (irrespective of when the AAGA occurred) collected over 12 months
Reports gathered by local coordinators across the whole of the UK (NHS only) and Republic of Ireland (all hospitals)
Type of report
300 reports
• 141 certain/probable
• 17 awake paralysis due to drug error
• 7 ICU
• 32 after sedation
The 141 certain/probable and possible reports were the basis of in-depth analysis
(the following summary applies to these)
Incidence
Estimated incidence of reports of AAGA was ~1:19,000
Incidence varied considerably in different settings
• 1:8000 with NMB
• 1:136,000 without
Psychological experiences
Wide range, most reports lasting <5 min
Distress particularly likely when patients experienced paralysis
Longer-term psychological effects identified in approximately 50 %
Risk factors: four categories were identified
1/ Drug factors: neuromuscular blockade, thiopental, TIVA
2/ Patient factors: female gender, age (younger adults but not children); obesity, previous AAGA and possibly difficult airway management
3/ Subspecialties: obstetric, cardiac, thoracic, neurosurgical
4/ Organisational factors: emergencies, out of hours operating, junior anaesthetists
TIVA
AAGA approximately twice as likely during TIVA as during volatile anaesthesia
However, many AAGA cases during TIVA involved use of non-TCI techniques: inadequate dosing using non-TCI regimens was common
High-risk situations were conversion of a volatile anaesthetic to TIVA and transfer of paralysed patients outside theatres
NMB
93 % of reports to NAP5 concerned patients who had received an NMB
Depth of anaesthesia monitoring
Specific monitors are rarely used during general anaesthesia in UK practice—processed EEG in 2.8 % of general anaesthetics
The overall findings are supportive of the use of depth monitors during TIVA with NMB
End-tidal anaesthetic gas monitoring is an alternative to depth monitoring, but in ~75 % of reports to NAP5, it would likely have been impractical or ineffective at preventing AAGA
A survey of anaesthesia activity in the UK in 2013 estimated that there were approximately 2.8 million GAs that year and that only 2.8 % had depth of anaesthesia (depth) monitoring [5]. There are several likely reasons to explain this low percentage. First, there are practical difficulties. During surgery, the scalp is often inaccessible and the sensors readily detect electrical interference especially from diathermy. The EEG itself is a random waveform which changes rapidly. Its interpretation is complicated and depends on the circumstances of the patient. In an attempt to simplify the situation, monitors have been developed that process the EEG (pEEG) and present to the anaesthetist a score related to central nervous system (CNS) suppression (usually a score from 100 to 0). The score however is derived from a complex algorithm, and the ordinary anaesthetist will not understand the process within the monitor and may treat the score with suspicion. The process calculating the score requires a length of EEG and therefore takes time. A delay, perhaps of 15 or 30 s, may be considered to be unacceptable in some circumstances. Finally there is an easier alternative to pEEG monitoring. In contrast to pEEG, the inspired anaesthetic vapour concentration monitoring is reliable and practical in all patients having an inhalational anaesthetic via a tracheal tube or supraglottic airway device.
Immobility
There has also been a misunderstanding of what the EEG monitor can achieve. It was a common assumption that a patient who moved was awake, and therefore a monitor was wanted that would warn of movement. We now appreciate that all pEEG monitors will fail to reliably predict movement because movement during anaesthesia is controlled primarily through the spinal cord. In rodents the dose required to immobilise decorticate animals is same as that for intact animals [6]. In goats, the cerebral and spinal cord circulations can be isolated, and by adjusting anaesthesia levels separately in the brain and spinal cord, it has been demonstrated that almost always immobility is related to spinal cord effect [7]. In humans halothane suppresses movement at lower BIS values than sevoflurane which could mean that the EEG effects of the agents are different or that halothane suppresses the spinal cord more than sevoflurane [8]. These studies support the idea that in respect of the relationship of dose of anaesthetic to movement, the spinal cord is more important than the cortex (which is where consciousness is assumed to lie). EEG monitors, therefore, cannot measure the effect of anaesthesia in respect of the likelihood of movement. The anaesthetist, on the other hand, may wish to deliver anaesthesia to the endpoint of immobility, and for this task, EEG monitors are not useful.
The dose that causes immobility however may be so high that it is close to the dose that could cause cardiovascular depression in vulnerable patients. Consequently, neuromuscular blocking drugs (NMBs) may be indicated but such a step makes the patient vulnerable to AAGA . Inhalational anaesthesia can be monitored by gas analysis, but total intravenous anaesthesia (TIVA ) cannot as yet be monitored continuously. It is the scenario of TIVA therefore, in the presence of NMBs, that a depth monitor may be most useful. In the UK in 2013, 23 % of patients managed with TIVA and NMBs had depth monitoring [5].
AAGA : Unmet Need
EEG depth monitors have been available widely for two decades. Electrode placement and cabling have been simplified and are less impractical than before. In a survey of opinions Myles et al. [9] found that anaesthetists were prepared to use depth monitoring if there was evidence that it prevented AAGA . To date the evidence is not clear enough to be certain, but as the technology improves, the potential for utility may increase.
In the UK NAP5 study [2], the likely causes of AAGA were examined in a large group of patients reporting AAGA in 2013 (see Table 19.1). Of the 300 patients with reliable reports, 28 were managed with TIVA (and NMB). This was a surprisingly large group, but the main problem with TIVA was that the propofol doses were clearly too low. Four groups could be identified:
Sick patients in whom the dose has been reduced to prevent cardiovascular depression
Transfer of patients to from resuscitation or surgery to intensive care
Poor dosing—failure of technique
Infusion errors (disconnection, extravasation, syringe driver failure)
In 7 % of reports (all techniques including TIVA and inhalational techniques), the reason for AAGA was not determined inferring that the doses used were within accepted practice and yet were inadequate.
The clinician needs a monitor to warn of both inadequate and excessive depths of anaesthesia. The following discusses the uncertainty of propofol anaesthesia and prepares the way for understanding the need for more routine use of depth monitoring.
Uncertainty About Propofol Dose and Effect
Pharmacodynamics
It is generally accepted that anaesthetic effect is related, at least in part, to the concentration of drug at ligand-gated receptors at the target site. The main target receptors are GABA-A which are rapidly acting membrane ion channels that inhibit action potentials. However, other receptor types may also be involved, and there are intracellular mechanisms that regulate and modify the number and effect of the activated receptors. The true relationship between receptor site drug concentration and drug effect therefore is variable both between and within individuals.
The target receptors are in the CNS not the blood. The relationship between blood and target propofol concentration is unknown but is inferred by the EEG . The assumption is that the EEG effect is related to blood level because it is assumed that target concentration will match the blood level, albeit after a short delay. This is the principle of effect-site concentration.
Pharmacokinetics
Blood levels of propofol cannot be measured continuously. Rapid near patient blood testing is possible but not widely available yet [10, 11]. Expired gas monitoring can detect propofol but is not yet accurate enough for clinical use [12, 13]. Tracking blood levels frequently over time, therefore, has been achieved only from a limited number of patients. The majority of pharmacokinetic data have been derived from much larger samples of patients in whom only a few blood levels have been obtained [14], and in these studies the relationship of dose to blood level, even with target controlled infusions, varies widely [15].
Cardiovascular Depression
Propofol reduces both cardiac contractility and peripheral vascular resistance [16]. Consequently, the dose has to be reduced in patients with cardiovascular disease or instability, but by how much it should be reduced is uncertain. Neuromuscular blocker drugs (NMBs) and potent opioids remove the possibility of movement and modify the autonomic reaction to pain so that patients who cannot move may not change their heart rate and blood pressure appreciably to alert the anaesthetist of AAGA [2].
Mortality
Sick and elderly patients are vulnerable to cause cardiovascular depression. One hopes that serious problems are rare. In the common situation however, the certainty of propofol dose and effect can also be challenged. A worrying association has been found between mortality and three coincident features of anaesthesia: EEG suppression, low blood pressure and low doses of anaesthesia (so-called triple low) [17, 18]. In sedated patients in intensive care, burst suppression is associated with high mortality. Such concepts are concerning and make EEG monitoring desirable.
In summary, the dose of propofol that achieves anaesthesia in sick patients managed with NMBs is uncertain. Measuring blood levels would help but there would still be a degree of uncertainty. Depth monitors may help these patients, and their use is increasing.
The remainder of this chapter explores the potential of how EEG monitoring can assess depth in TIVA patients. It has four sections:
Concepts
Essential EEG knowledge for the clinician
Evidence for and against EEG DoA monitoring
Future directions and the potential for clinical utility
Concepts
What Is Depth of Anaesthesia ?
The concept of depth has not always been accepted. It can be said that a patient is either anaesthetised or not and that depth is therefore a false construct [19]. Whereas this statement is reasonable from the patient’s perspective in terms of their consciousness , there are additional neurological effects that take place beyond the point of loss of consciousness , and these effects constitute a progressive suppression of the CNS. The stages of ether anaesthesia originally described by Guedel in 1937 show that increasing the dose of anaesthesia progressively suppresses important neurological reflexes and functions [20]. For example, the dose causing suppression of the response to pain is less than that which causes apnoea. A more recent example is the demonstration that after consciousness has been lost, increasing the blood propofol concentration, tracked by processed electroencephalography (pEEG), can prevent a response to defined painful stimuli of increasing severity [21] (Table 19.2). In a recent editorial Pandit [23] described anaesthesia as being “a spectrum of brain states” because not all parts of the brain, and its associated functions, are suppressed similarly and this will lead to variation in the characteristics of CNS suppression at various doses.
Table 19.2
Clinical markers of depth of anaesthesia
Score | Description | Level of sedation or anaesthesia |
---|---|---|
5 | Responds readily to name spoken | Minimal |
4 | Lethargic response to name spoken | Moderate |
3 | Responds after name called loudly/repeatedly | Moderate |
2 | Purposeful response to mild-to-moderate shaking | Moderate |
1 | Responds to trapezius squeeze | Deep |
0T | No response to trapezius squeezea | Light general anaesthesia |
0E | No response to electrical stimulationb | Deeper general anaesthesia |
In principle, increasing the dose of an anaesthetic beyond that needed to cause unconsciousness has two related effects.
- 1.
It increases brain suppression. There are, however, a few minor exceptions: ether anaesthetics have an excitement phase (notorious with diethyl ether, but only at induction and at low doses), benzodiazepines can cause clinical paradoxical excitement during sedation, and nitrous oxide and ketamine cause paradoxical EEG excitement.
- 2.
It reduces the likelihood of AAGA . There are no known analeptic effects of anaesthesia, and it may be assumed that increasing the dose will reduce the chance of any reaction to an arousing stimulus.
If these two statements are accepted, anaesthesia depth is related to dose. Yet how certain is this principle?
Is Depth Related to Anaesthesia Blood Level?
Before an operation begins , the patient is given an effective blood level and appears adequately anaesthetised. When the operation begins, the patient often reacts by moving or having a tachycardia (or other autonomic responses). The blood level of anaesthetic has to be increased to stop the patient reacting. The certainty of effect is therefore only temporary, and anaesthesia depth varies even when the blood level of anaesthetic remains constant. Blood levels will need to be increased, or decreased, appropriately to match the stimuli. Whereas it is true that increasing the blood level increases the depth, we cannot be certain about the depth at a specified blood level. It is helpful to know the blood level, but information about the effect on the target organ may be useful.
How Can Consciousness and Depth Be Described?
Consciousness and anaesthesia , two commonly used terms, are both difficult to describe, and their measurement is elusive. However descriptions or definitions of various levels of consciousness are generally accepted [24]. The levels of sedation (minimal, moderate and deep) leading to anaesthesia have been defined within a so-called continuum of level of consciousness [24]. The continuum infers that the boundaries between the levels are blurred. In principle the levels are useful but in practice the patient can move from one level to the next quickly and subtle changes are difficult to chart accurately. The levels of sedation have been used to create a widely used scale of sedation known as the Observer’s Assessment of Alertness and Sedation (OAA/S) scale: a scale ranging from 5 (full consciousness ) down to 0 (unconscious, or anaesthesia, as defined by no response to painful stimulus) [22].
There are few descriptors of depth of unconsciousness. The Extended Observer’s Assessment of Alertness and Sedation (EOAA/S) score has recently been proposed (see Table 19.2) by Kim et al. [21]. It uses two painful stimuli, one more intense than the other and depth of unconsciousness is described by the lack of response to these.
Diagrammatic Representation of Consciousness and Depth
Increasing CNS suppression can be represented on a line (Fig. 19.1). The line represents the consciousness -anaesthesia depth continuum: the state of full wakefulness is on the extreme left and total cortical suppression on the extreme right. In this model a drug such as propofol is given to achieve increasing blood levels. The distance between the far left point and any other point on the line is proportional to the blood level of propofol. The points on the line represent the blood level of propofol but these are arbitrary and have no units or scale. The patient begins at the extreme left, and the brain will be progressively suppressed so that consciousness is lost at some point X on the line. X separates consciousness from anaesthesia. Further travel along the line to the right beyond X represents increasing depth.
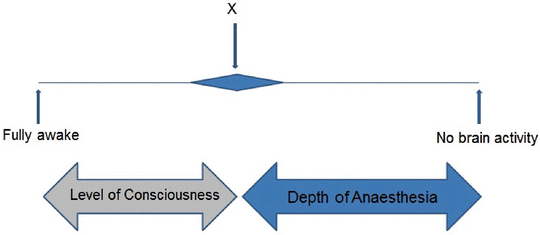
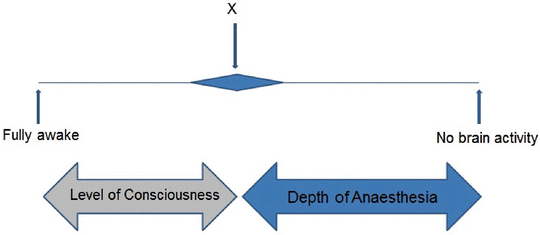
Fig. 19.1
Consciousness and depth. A horizontal line representing the continuum of cortical depression related to the blood level of propofol. The midpoint (X) marks the point of loss of consciousness and separates consciousness from of anaesthesia. The terms “level of consciousness ” and “depth of anaesthesia ” are therefore distinct. The line length is proportional to the dose of an anaesthetic drug such as propofol. The blue horizontal diamond illustrates that X has variability: X may represent the median dose of propofol causing unconsciousness in a population, or it could represent the variability within an individual over time.
On the consciousness part of the line lie the levels of sedation. The line (see Fig. 19.2) shows the distance between moderate sedation (known as or conscious sedation in the UK) and loss of consciousness , and this represents the difference between the blood levels required to cause sedation and loss of consciousness ; it represents the width of the margin of safety of sedation.
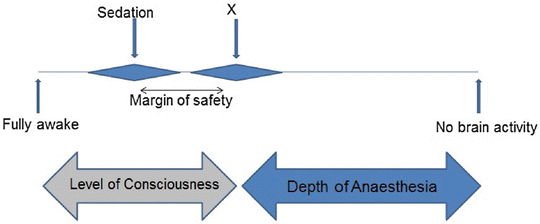
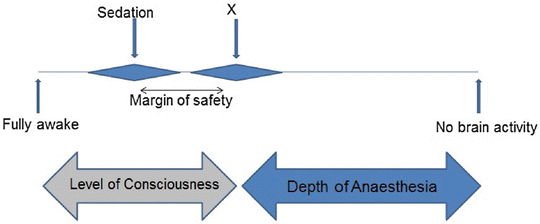
Fig. 19.2
Sedation and margin of safety. A horizontal line representing the continuum of cortical depression related to the blood level of propofol. The line length is proportional to the dose of an anaesthetic drug such as propofol. The midpoint (X) marks the point of loss of consciousness and separates consciousness from of anaesthesia. The distance between the points marked by sedation and X is proportional to the difference between the doses required to cause sedation and unconsciousness. This describes margin of safety of a moderate sedation technique. The blue horizontal diamonds illustrate potential variation of the points marked by “sedation” and X.
What happens to the right of X is less clear. As CNS suppression progresses, I propose that there are three zones (Fig. 19.3). In the zone centred on X, the patient appears anaesthetised, yet they can, in theory, react to stimuli and move back into sedation and consciousness . In this zone therefore the patient is only probably anaesthetised; their unconsciousness is uncertain. Y marks the point of definite anaesthesia in which the patient can be stimulated but does not react and therefore is safe from a return to unwanted consciousness . In the zone centred on Y is a state that could be termed definitely anaesthesia. The final zone has danger. Z is the point at which the patient is unnecessarily deep and they are in danger of cardiovascular depression or other unspecified damaging effects. The distances between these points or zones are unknown and could be investigated. The distance between Y and Z could represent the margin of safety for depth of anaesthesia. The distances between X and Y could be termed a margin of uncertainty of anaesthesia.
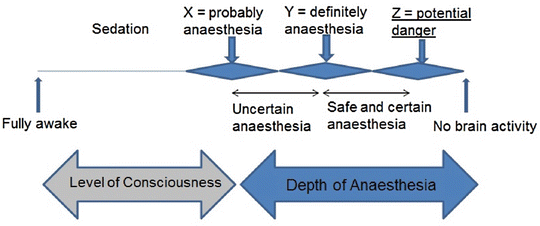
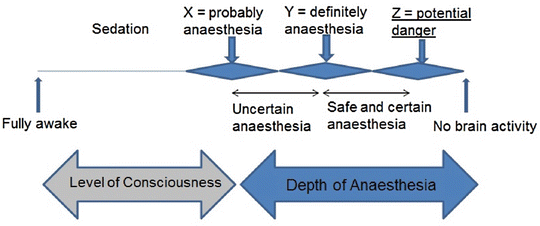
Fig. 19.3
Depth of anaesthesia and margin of safety. A horizontal line representing the continuum of cortical depression related to the blood level of propofol. The line length is proportional to the dose of an anaesthetic drug such as propofol. X (loss of consciousness ) could be considered to be “probably anaesthesia”. Higher doses are needed to ensure the patient is definitely anaesthetised (Y). Excess doses beyond this point could expose the patient to harm (Z). The distance between X and Y could be termed a margin of uncertainty (within anaesthesia). The distance between Y and Z could represent the margin of safety of anaesthesia. The blue horizontal diamonds indicate potential variation of X, Y and Z.
Is EEG Related to Consciousness?
The EEG changes according to the position on this line. The rapidity of change of consciousness is a major problem because usually an EEG monitor reacts too slowly to be of use. Often the EEG can do no more than confirm the observation that a patient has changed their conscious level. For example, if target controlled infusion (TCI) of propofol is set at a low target blood level (e.g. 1 mcg/ml), a patient may become deeply sedated before the EEG can detect it. Likewise, if a procedure is undertaken on deeply sedated patient, they could awaken before the EEG changes. The EEG is not useful in either situation. In practical terms, therefore, the EEG is not useful for rapid changes in consciousness : propofol sedation is likely to be in this category. Some studies have shown a relationship between EEG and conscious level [25], but there is considerable variation and the association is loose and unreliable.
In the sedated patient observation alone is likely to be better than relying on an EEG . If an EEG could warn of impending loss of consciousness or unanticipated awakening, then it would have utility. The evidence (below) shows that the EEG is poor at this task for sedation. Other monitors, such as capnography, have a higher priority in this situation, and the observer would need to intervene to maintain a patent airway rather than wait for the EEG to change.
Is EEG Related to Depth?
The anaesthesia zone concept is theoretical and, as yet, has no accepted descriptors or markers. Can the EEG help? The EEG is a measure of cortical suppression, and this is a strong reason to consider the EEG as a useful surrogate marker of both consciousness and depth. The validity of this statement is difficult to prove beyond doubt but it has a degree of truth. Conscious level too often changes too quickly during propofol sedation, but if depth changes less quickly, perhaps the EEG could be useful.
Is EEG Related to Dose?
This depends on several factors, the most important being the type of EEG , the drug or combination of drugs and the presence of painful stimulation. Testing the relationship of EEG to dose would require steady-state conditions and these are often impractical. Possibly, there is a strict relationship between propofol blood level and EEG effect, but this might only apply to unstimulated patients. Once the patient is stimulated, there could be a rapid change in EEG . Possibly, the relationship between propofol blood level and EEG effect is non-linear (see Fig. 19.4) so that within a certain range of blood level, the EEG remains relatively unchanged with stimulation, but if the blood level is lower than this range, stimulation could cause awakening. Nevertheless, the effective blood level range would depend upon pain, analgesia and other sedative drugs.
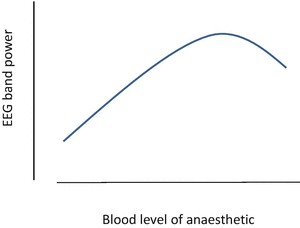
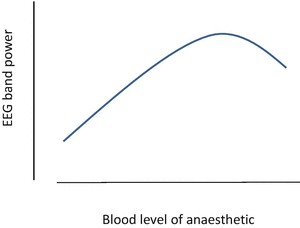
Fig. 19.4
Common relationship of EEG band power to concentration of anaesthetic
Essential EEG Knowledge for the Clinician
General EEG Knowledge
The EEG detects electrical activity of the outer layer of cerebral cortex from scalp electrodes. The signals are considered to be postsynaptic potentials originating from groups of axons. The waveform is random, complex and of variable and low amplitude (usually less than 100 μV). Signals from muscle are much larger (up to 500 μV) and easily hide the EEG in a moving patient. Visual inspection of an EEG shows oscillations between 1 and 20 Hz. The EEG is unequal over the scalp: parietal, central and frontal positions are most accessible and give highest amplitude signals. An EEG specialist can describe these and interpret them. There are three features: a continuous waveform, transients and periods of electrical silence. Transients are seen in natural sleep. The continuous waveform changes with consciousness and depth [26]. Electrical silence occurs with deep anaesthesia (and coma).
Unprocessed EEG in Natural Sleep
The EEG in awake and natural sleep is irregular. Conventional classification of EEG frequency bands are gamma (>30 Hz), beta (14–30 Hz), alpha (8–13 Hz), theta (4–7) and delta (<4 Hz). In the relaxed awake state, intermittent alpha oscillations are common, but these disappear in the alert state—the EEG becomes low amplitude with frequencies between 30 and 50 Hz. Sleepiness usually begins with large slow waves, and as sleep progresses five EEG stages can be seen. In stage 1 alpha oscillations become less regular and eventually disappear; background amplitude decreases. Sleep spindles characterise stage 2 (these are prominent transients of 12–14 Hz lasting more than 0.5 s, beta and theta activity become obvious and other transients appear (vertex sharp waves and K-complexes)). Stages 3 and 4 are marked by increasing prominent theta waves. Stages 1–4 are termed slow wave sleep (SWS). Stage 4 SWS progresses to a fifth stage known as rapid eye movement (REM) sleep in which eye movements cause large voltage shifts in frontal channels. REM has background low amplitude gamma activity and looks similar to that seen in the alert state.
Unprocessed EEG in Anaesthesia
The EEG under anaesthesia is relatively regular. There are methodological problems when comparing different drugs (and doses) because efficacy is usually assessed by suppression of movement to a painful stimulus, which is known to be mainly influenced by the spinal cord. High doses of propofol are needed to suppress movement unless an opioid is used, and the different inhalational agents have different spinal cord suppression effects. Therefore it is difficult to be sure that the EEG effects of various drugs have been compared under equal depth doses. Nevertheless the differences between the vapour anaesthetics and propofol are probably not important. EEG changes during progressively increasing doses of propofol [27], sevoflurane [28] and isoflurane [29] anaesthesia are broadly similar.
At induction the waveform becomes low amplitude and prominent oscillations disappear. Oscillations of 8–20 Hz appear and these become slower and more regular and increase in amplitude as doses increase. At deeper levels there are periods of EEG suppression alternating with bursts of high amplitude slow waves: this is burst suppression [30]. Further increasing the dose causes electrical silence . All these changes are reversed as anaesthesia dose reduces, and pain also causes some reversal [31].
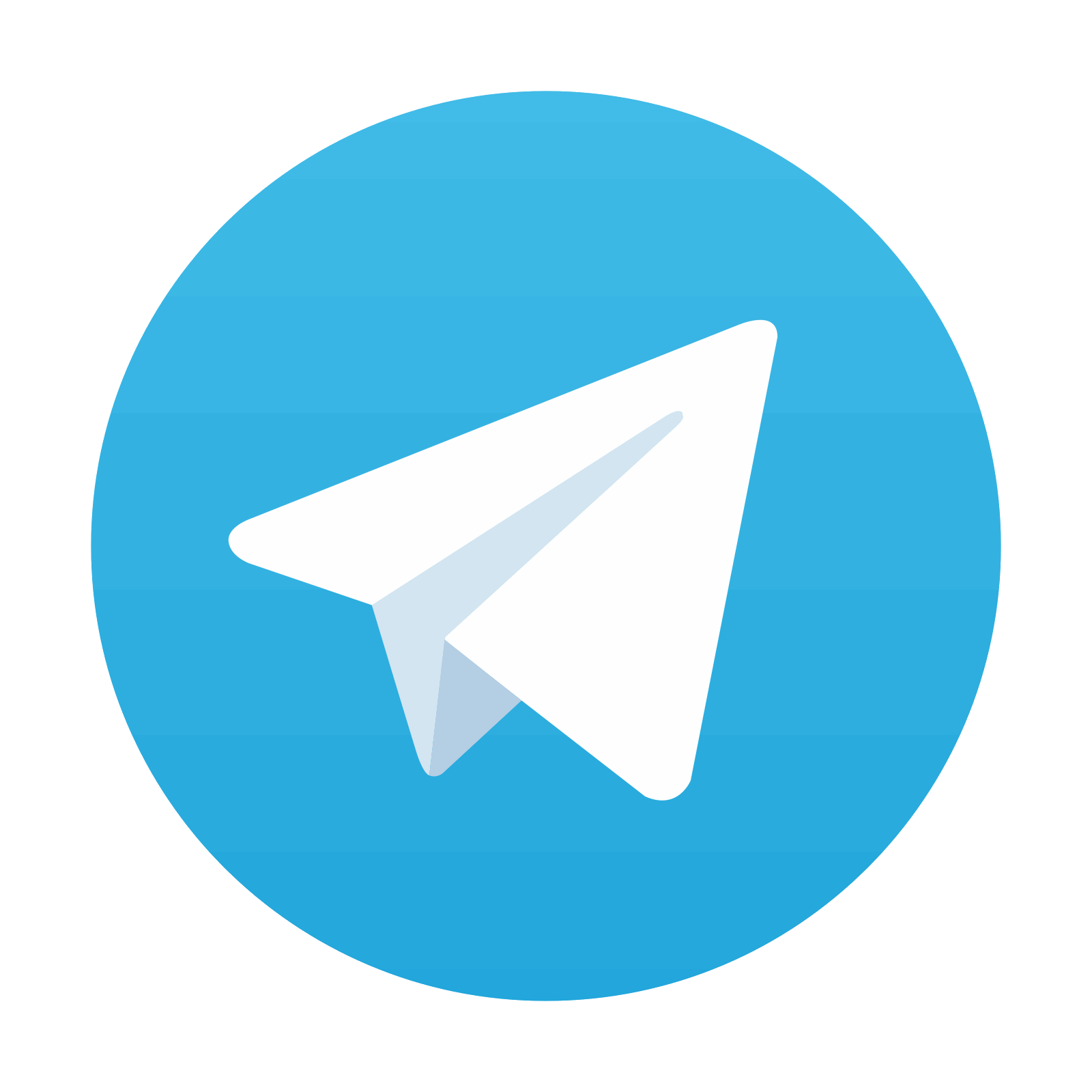
Stay updated, free articles. Join our Telegram channel

Full access? Get Clinical Tree
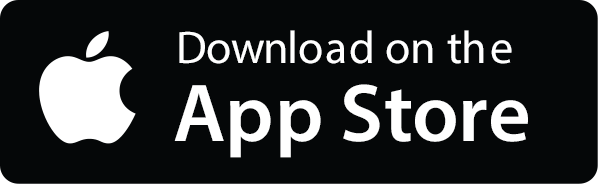
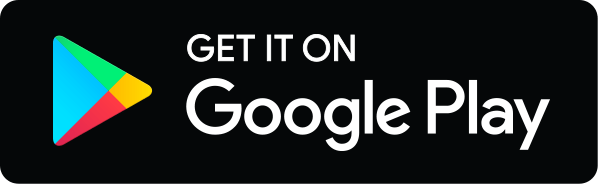