66 Community-Acquired Pneumonia
Pneumonia is an infection of the gas-exchanging units of the lung that is most commonly caused by bacteria but occasionally due to viruses, fungi, parasites, and other infectious agents. It is the eighth leading cause of death in the United States and the number one cause of death from infectious diseases.1 When this infection arises in patients who are residing out of the hospital, it is termed community-acquired pneumonia (CAP), although the population included in this definition is expanding. Currently the “community” includes complex patients such as those who have recently been hospitalized, those in nursing homes, and those with chronic diseases who are commonly managed in such facilities as dialysis centers or nursing homes. These patients are now referred to as having “healthcare-associated pneumonia” (HCAP), and it remains controversial whether their treatment should be similar to that for CAP or nosocomial pneumonia.2
Incidence
In 1994, over 5.6 million people were diagnosed with CAP in the United States. The majority, 4.5 million, were treated out of the hospital, and only a minority of hospitalized patients were cared for in the intensive care unit (ICU).1,3 Although the majority of patients with CAP are managed in the outpatient setting, morbidity, mortality, and the major portion of the cost of treatment is focused on hospitalized patients, particularly those admitted to critical care units. In addition, those patients with comorbid illness and those of advanced age make up a large proportion of the hospitalized critically ill population. In particular, the elderly have a higher mortality from CAP than younger patients, generally as a reflection of the fact that they more commonly have comorbid illness.3
Although CAP can vary from being a mild to a severe illness, very few hospitalized patients are severely ill enough to require ICU admission.4–5 Torres, et al. specifically examined all ICU admissions over a 4-year period and found that 10% were related to CAP.4 In that study, CAP patients who required ICU care were admitted directly to the ICU 42% of the time, after admission to another ward 37% of the time, and after transfer from another hospital in 21% of patients.4 In another study of 395 patients admitted to the hospital with CAP, only a total of 64 (approximately 15%) were admitted to the ICU.6 Recently, Woodhead et al. found that CAP accounted for 5.9% of all ICU admissions, but that early admission (within 2 days of hospitalization) was associated with a lower mortality (46.3%) than late admission (>7 days in the hospital, 50.4% mortality).7
Kaplan and colleagues evaluated the cost of care for elderly patients with CAP in the United States.5 Using Medicare data, they evaluated all individuals aged 65 or older admitted to nonfederal hospitals in 1997. A total of 623,718 patients were evaluated, with 86% being aged 70 or older, and the mean age was 77 years. Underlying illness was present in two-thirds, with congestive heart failure, the most common comorbidity, present in 32%. In this population, the use of ICU, mechanical ventilation, or both was common, with 140,226 patients having complex courses of illness. The overall mortality rate was 10.6% but rose higher with advancing age, nursing home residence, and comorbid illness. The mean length of stay was 7.6 days, with a mean cost of $6949, but costs were greater for patients with complex illness and mechanical ventilation and less for those with simple pneumonia. Costs generally paralleled length of stay but were disproportionately high for those needing mechanical ventilation, where the mean length of stay was 15.7 days and the cost $23,961. Interestingly, there was little extra cost for nonsurvivors compared with survivors, except in the group with complex pneumonia as a whole but not in those requiring mechanical ventilation. The findings not only emphasize the high impact of CAP on costs and outcomes in the United States but also demonstrate the disproportionate increase in costs when patients are treated with mechanical ventilation, thereby raising for discussion the ethics and appropriateness of such care in the very elderly. Other studies of CAP have reported that costs are higher for patients with comorbid illness than those without, but in those without comorbid illness, the cost for those who died was less than for those who survived, while the opposite was true when the entire CAP population was considered.8
Risk Factors for Developing Severe CAP
In all studies of CAP, patients who are admitted to the hospital or ICU commonly have a number of coexisting illnesses, suggesting that individuals who are chronically ill have an increased risk of developing severe illness (Box 66-1). In one study, the mean age of all CAP patients was 59 years, coexisting illness was present in 46%, whereas 74% had a history of prior cigarette smoking.6 Patients often have a history of coexisting illness, and the most common chronic illnesses in these patients are respiratory disease, cardiovascular disease, and diabetes mellitus, findings that have been echoed in a number of studies.1,4,9 In studies of severe CAP, serious coexisting illness is present in 46% to 66% of all patients.4,5,9 The most common respiratory illness in CAP patients is chronic obstructive pulmonary disease (COPD), a finding that applies to those with either mild or severe forms of CAP.4 Among those with severe CAP, cigarette smoking and alcohol abuse are also quite common, and cigarette smoking has been identified as a risk factor for bacteremic pneumococcal infection.4,10 Other common illnesses associated with CAP include malignancy, neurologic illness (including seizures), as well as AIDS.1,9,11 One study identified alcohol abuse as a risk factor, along with the failure to receive antibiotic therapy before hospital admission, a finding suggesting that a delay in therapy may convert milder forms of pneumonia into a more severe illness.9,11 In addition, genetic differences in the immune response may predispose certain individuals to more severe forms of infection and adverse outcomes, and may be reflected by a family history of severe pneumonia or adverse outcomes from infection.
Prognostic Factors
In a meta-analysis of 33,148 patients with CAP, the overall mortality rate (OR) was 13.7%, but those admitted to the ICU had a mortality rate of 36.5%.12 Eleven prognostic factors were significantly associated with mortality:
In other studies, the clinical features that predict a poor outcome (Box 66-2)1 include advanced age (>65 years), preexisting chronic illness of any type, absence of fever on admission, respiratory rate greater than 30 breaths/min, diastolic or systolic hypotension, elevated blood urea nitrogen (>19.6 mg/dL), profound leukopenia or leukocytosis, inadequate antibiotic therapy, need for mechanical ventilation, hypoalbuminemia, and the presence of certain “high-risk” organisms (type III pneumococcus, Staphylococcus aureus, gram-negative bacilli, aspiration organisms, or postobstructive pneumonia). Other studies have found that when CAP patients have a delay in the initiation of appropriate antibiotic therapy, mortality is increased.1,4,11,13
Box 66-2
Risk Factors for a Poor Outcome from Community-Acquired Pneumonia
One study of 3233 patients in Spain found that risk factors for all-cause mortality were a higher severity of illness on admission, need for ICU care, and the presence of multilobar infiltrates. However, late mortality (after at least 3 days) was reduced if blood cultures were negative, antibiotic therapy was consistent with guidelines, and if an etiologic agent was identified.14 Thus severity of illness on admission most affects early mortality, while therapy-related, modifiable risk factors impact late mortality.
One approach to evaluating CAP patients is to use a scoring system to define prognosis and predict the risk of death. The investigators in the Pneumonia Outcomes Research Team (PORT) study have developed a mortality prediction rule that classifies all patients into one of five groups (Pneumonia Severity Index [PSI] classes I to V), each with a different risk for death.15 Patients in classes IV and V have a predicted mortality risk of 8.2% to 9.3% and 27% to 31.1%, respectively, whereas those in classes I and II have a mortality risk of 0.1% to 0.4% and 0.6% to 0.7%, respectively, and those in class III have a risk of death of 0.9% to 2.8%. To use this scoring system, patients have points calculated based on such factors as age, sex, presence of comorbid medical disease, certain physical findings, and certain laboratory data.15
Although the PORT scoring system has been shown to be accurate for predicting mortality and prognosis, it is important to realize that it does not directly measure severity of illness, since many points in the scoring system are for comorbid conditions rather than features of illness. The investigators from the PORT study evaluated the use of ICU by patients with CAP and the ability of the scoring system to predict need for ICU care. From their original database, 170 patients were admitted to the ICU and compared to 1169 who were managed out of the ICU. While the PORT rule was useful for predicting mortality, there was a poor correlation between the need for ICU admission and the risk of death. In fact, 27% of the ICU patients were in PSI risk classes I to III, and this group, although needing intensive care, had a significantly lower mortality than patients in risk classes IV and V.16 In another study, patients in PORT class V were evaluated, and only about 20% needed ICU admission; they had a 37% mortality compared to the 20% mortality of the PSI V patients who did not need the ICU.17 In general, the PSI V patients who needed the ICU tended to get more of their points from acute illness, while those not needing the ICU tended to score points because of chronic disease factors. The findings are quite important for demonstrating that the need for ICU care does not always equate with a high risk of death. In the Infectious Diseases Society (IDSA)/American Thoracic Society (ATS) CAP guidelines, these limitations were discussed, including the fact that age and comorbidity are heavily weighted variables for defining mortality risk, tending to move all older patients into high PORT score classes.1,18 On the other hand, in a young patient without comorbid illness, the pneumonia must be particularly severe to place the patient in a high-mortality risk group, and certain vital sign thresholds must be exceeded to accumulate points toward a poor prognosis. These thresholds are heart rate greater than 125 beats/min, respiratory rate greater than 30 breaths/min, and systolic blood pressure less than 90 mm Hg.
Although prognostic scoring systems can be complex and difficult to apply in clinical practice, the PORT prediction rule has been promoted as a way to avoid overestimating severity of illness, and calculation of the score has been advocated as a way of keeping some patients out of the hospital who have a low risk of death. For the critical care physician, the opposite problem—underestimating severity of illness—is a more serious concern, and the use of the CURB-65 approach, modified from the British Thoracic Society (BTS) rule, is a simple and accurate way to address this issue. CURB-65, an acronym for the clinical features used to assess pneumonia severity and prognosis,18 assigns 1 point, on a 5-point scale, to confusion, blood urea >7 mmol/L (19.6 mg/dL), respiratory rate ≥30 breaths/min, blood pressure <90 mm Hg systolic or ≤60 mm Hg diastolic, and age ≥ 65 years. In one study, when the score was 0 to 1, the mortality rate was 0%, whereas mortality was more than 20% for a score of 3 or higher, and those with a score of 2 had a mortality of 8.3%.
Use of the CURB-65 rules may be a problem in the elderly, reflecting the altered clinical presentations of pneumonia in this population. In one study, a rule similar to CURB-65 had a 66% sensitivity and a 73% specificity for predicting mortality in a population that included 48% of patients who were at least 75 years of age.19,20 Interestingly, although the rule was not optimal in an elderly population and did not work as well as it did in other populations, it had a higher sensitivity for predicting mortality than the Prognostic Scoring Index (PSI) derived from the PORT study.15,20 Some studies have compared the PSI and CURB-65 and found them to be similar for identifying low-risk populations, but the CURB-65 may be more discriminating for identifying poor prognosis in those with severe illness, compared to the PSI.21
Other prognostic scoring systems have been developed to define the presence of severe pneumonia. One called the CUR-XO is based on defining the need for ICU admission by the presence of one of two major criteria: arterial pH < 7.30 or systolic BP < 90 mm Hg.22 In the absence of these criteria, severe CAP can also be identified by the presence of two of six minor criteria including: confusion, BUN > 30 mg/dL, respiratory rate > 30/minute, PaO2/FIO2 ratio < 250, multilobar infiltrates, and age of at least 80. When these criteria were met, the tool was 92% sensitive for identifying those with severe CAP and was more accurate than the PSI or CURB-65 criteria, although not quite as specific as the CURB-65 rule.22 Using this approach, some criteria (acidosis and systolic hypotension) are weighted more heavily than others, which contrasts with the approach of some of the other approaches to define severe CAP.
A different approach than assessing risk for death is to use scoring systems to define the need for ICU interventions such as intensive respiratory and vasopressor support (IRVS). The SMART-COP tool was developed to predict the need for IRVS.23 Using a multi-variate model, there were eight clinical features associated with the need for IRVS: systolic blood pressure <90 mm Hg, multilobar infiltrates, albumin < 3.5 g/dL, respiratory rate elevation (≥25 for those ≤age 50, and ≥30 for those >age 50), tachycardia (>125/min), confusion, low oxygen (<70 mm Hg if ≤age 50 or <60 mm Hg if >age 50), and arterial pH <7.35. The abnormalities in systolic blood pressure, oxygenation, and arterial pH each received 2 points, while the 5 other criteria received 1 point each, and with this system, the need for IRVS was predicted by a SMART-COP score of at least 3 points. Using this cutoff, the sensitivity for need for IRVS was 92.3% and the specificity 62.3%, with a positive and negative predictive value of 22% and 98.6%, respectively. The PSI and CURB-65 did not perform as well overall for predicting the need for IRVS.
Pathogenesis
Pneumonia results when host defenses are overwhelmed by an infectious pathogen. This may occur because the patient has an inadequate immune response, often as the result of underlying comorbid illness (congestive heart failure, diabetes, renal failure, COPD, malnutrition), because of anatomic abnormalities (endobronchial obstruction, bronchiectasis), as a result of acute illness-associated immune dysfunction (as can occur with certain viral infections), or because of therapy-induced dysfunction of the immune system (corticosteroids). Pneumonia can also occur in patients who have an adequate immune system if the host defense system is overwhelmed by a large inoculum of microorganisms (massive aspiration) or if the patient encounters a particularly virulent organism to which he or she has no preexisting immunity or to which the patient has an inability to form an adequate acute immune response.24,25
Recent studies have evaluated the normal lung immune response to infection and have shown that in most patients with unilateral CAP, the inflammatory response is limited to the site of infection, not spilling over to the uninvolved lung or the systemic circulation.26,27 In patients with localized pneumonia, tumor necrosis factor alpha (TNF-α), interleukin (IL)-6, and IL-8 levels were increased in the pneumonic lung and generally not increased in the uninvolved lung or in the serum.26,27 In patients with severe pneumonia, the immune response is characterized by a “spillover” of the immune response into the systemic circulation, reflected by increases in serum levels of TNF-α and IL-6.28 It remains uncertain why localization does not occur in all individuals and why some patients develop diffuse lung injury (e.g., acute respiratory distress syndrome [ARDS]) or systemic sepsis as a consequence of pneumonia. These complications may result from an inability to develop a brisk lung immune response, as a consequence of either specific bacterial virulence factors, inadequate or delayed therapy, or genetic polymorphisms that affect the immune response. In fact, one study suggested that if bacteria persisted in the lung in spite of therapy, then inflammation in the form of IL-1β was persistent and at a high level, presumably being driven by the ongoing presence of the organisms.29 Although there are a large number of genes that have been identified as being able to affect the severity and outcome of CAP by affecting the inflammatory response, the ability to use this information to impact patient management has not emerged.
Pneumonia-associated inflammation may also impact the long-term mortality of CAP. While the in-hospital mortality implications of CAP are well-known, there is also a high incidence of late mortality among hospitalized CAP patients. In one study of elderly patients hospitalized with CAP, the 1-year mortality rate exceeded 40%.30 The explanation for this finding is unclear, but other studies have shown that patients with high levels of systemic inflammation (defined by serum levels of IL-6 and IL-10) on admission and on discharge have an increased mortality at 6 months to 1 year.31 In addition, patients with evidence of cardiac dysfunction complicating CAP, as reflected by high serum levels of B-natriuretic peptide (BNP) are also likely to have increased disease-related mortality.32
Clinical Features
Symptoms and Physical Findings
Patients with CAP and an intact immune system have a normal pulmonary response to infection and generally have respiratory symptoms such as cough, sputum production, and dyspnea, along with fever and other complaints. Cough is the most common finding and present in up to 80% of all patients but is less common in the elderly, those with serious comorbidity, or patients coming from nursing homes.33 The elderly generally have fewer respiratory symptoms than younger individuals; as mentioned, the absence of clear-cut respiratory symptoms and an afebrile status have themselves been predictors of an increased risk of death.1,18 Pleuritic chest pain is also common in patients with CAP, and in one study its absence was also identified as a poor prognostic finding.34
In the elderly patient, pneumonia can have a nonrespiratory presentation with symptoms of confusion, falling, failure to thrive, altered functional capacity, or deterioration in a preexisting medical illness such as congestive heart failure.33,35 In one study, delirium or acute confusion were significantly more frequent in the elderly patients with pneumonia than in age-matched controls who did not have pneumonia.35 In that study, there was no association between the type of isolated microorganisms and the clinical presentation of CAP, except for pleuritic chest pain, which was more common in pneumonia caused by bacterial pathogens such as S. pneumoniae. Approximately 16% of elderly patients with pneumonia were considered well nourished, compared with 47% of controls, with kwashiorkor-like malnutrition being the predominant type of nutritional defect and the one associated with delirium on initial presentation. Several other studies have examined the clinical presentation of pneumonia in the elderly and found that a nursing-home elderly population had a substantially higher mortality rate than other individuals with CAP. These findings may be a reflection of the fact that patients residing in a nursing home had a higher frequency of comorbid illness and dementia. Metlay and coworkers studied 1812 patients of all ages and found that with advancing age, patients tended to have a longer duration of symptoms such as cough, sputum production, dyspnea, fatigue, anorexia, myalgia, and abdominal pain.33 In general, overall symptoms were less prominent in patients older than age 65 than in those who were younger.
Another study evaluated 1474 patients with CAP, of whom 305 were older than 80 years of age.36 The population excluded individuals in nursing homes and severe immune suppression (neutropenia, AIDS, and transplant). Clinically, the very elderly had less pleuritic chest pain, headache, and myalgias and were more likely to be afebrile and to have altered mental status on admission. Overall mortality was higher in the older patients (15% versus 6%), as were in-hospital complications and early mortality (within 48 hours). The PSI values, as expected, were higher in the older population, in part because comorbid illness and age itself add to the PSI score; but still, the mortality rate for patients in PSI class V was 24% in the younger population versus 32% in the elderly.
Physical findings of pneumonia include tachypnea, crackles, rhonchi, and signs of consolidation (egophony, bronchial breath sounds, dullness to percussion). Patients should also be evaluated for signs of pleural effusion. In addition, extrapulmonary findings should be sought to rule out metastatic infection (arthritis, endocarditis, meningitis) or to add to the suspicion of an “atypical” pathogen such as M. pneumoniae or C. pneumoniae, which can lead to complications as bullous myringitis, rash, pericarditis, hepatitis, hemolytic anemia, or meningoencephalitis. One of the most important ways to recognize severe CAP early in the course of illness is to carefully count the respiratory rate. In the elderly, an elevation of respiratory rate can be the initial presenting sign of pneumonia, preceding other clinical findings by as much as 1 to 2 days.37 In fact, in one study, tachypnea was the most common finding in elderly patients with pneumonia, being present in over 60% of all patients and occurring more often in the elderly than in younger patients with pneumonia.33
Radiographic Features
For most patients, CAP is defined by a combination of clinical symptoms and the presence of a new radiographic infiltrate, but not all patients with this illness will have this finding when first evaluated. Even when the radiograph is negative, if the patient has appropriate symptoms and focal physical findings, pneumonia may still be present. In one study, 47 patients with clinical signs and symptoms of CAP were evaluated with both chest radiography and high-resolution computed tomography (CT) of the chest.38 Eight patients with a negative chest radiograph were identified by CT to have pneumonia and, in general, more extensive disease was found on CT than on chest radiography.38 The findings of this study confirm the need to repeat the chest film after 24 to 48 hours in certain symptomatic patients with an initially negative chest film. Although some studies have suggested that febrile and dehydrated patients can have a normal chest radiograph when first admitted with pneumonia, the idea of hydrating pneumonia is in the realm of “conventional wisdom” and anecdotal reports.
The presence of alveolar densities (lobar or bronchopneumonic) has been associated with a high likelihood of a bacterial etiology, but it is extremely difficult to distinguish among specific pathogens by using patterns of radiographic abnormalities.39 The chest radiograph may have prognostic value in patients with severe pneumonia, with multilobar infiltrates or rapid progression of infiltrates serving as poor prognostic signs, helping to identify patients who require intensive care.1,4 Chest CT can also have value in the critically ill patient in situations when a noninfectious process is being considered, or when complications such as pneumothorax, empyema, or abscess are suspected. CT can suggest certain alternative noninfectious diagnoses such as granulomatous vasculitis, acute eosinophilic pneumonia, and bronchiolitis obliterans with organizing pneumonia.
Typical Versus Atypical Pneumonia Syndromes
In the past, the clinical and radiographic features of CAP have been organized into patterns of either “typical” or “atypical” pneumonia syndromes, with the idea being that specific patterns could suggest certain etiologic agents. The typical pneumonia syndrome is characterized by sudden onset of high fever, shaking chills, pleuritic chest pain, lobar consolidation, a toxic-appearing patient, and the production of purulent sputum. Although this pattern has been attributed to pneumococcus and other bacterial pathogens, these organisms do not always lead to such classic symptoms, particularly in the elderly. The atypical pneumonia syndrome, which is characterized by a subacute illness, nonproductive cough, headache, diarrhea, or other systemic complaints, is usually due to infection with M. pneumoniae, C. pneumoniae, Legionella species, or viruses. However, patients with impaired immune responses may present in this fashion, even with bacterial pneumonia. Thus, the ability to use the features on clinical presentation to predict the likely etiologic agents is limited and often misleading.1,39–41
In one study examining the microbial etiology and clinical presentation of CAP, clinical features were no more than 42% accurate in differentiating pneumococcus, M. pneumoniae, and other pathogens from one another.40 In another study of 359 patients with CAP, a comparison of patients with S. pneumoniae, H. influenzae, L. pneumophila, and C. pneumoniae revealed no significant differences in their clinical presentations.41 The limitations of clinical features in defining microbial etiology also apply to evaluations of radiographic patterns.39
Using Clinical Features to Define Severe Community-Acquired Pneumonia
Although there is no uniformly accepted definition for severe CAP, this term generally refers to any patient who is admitted to the ICU because of CAP. Most of these patients have “respiratory failure” which is defined by the presence of hypoxemia or hypercarbia, and not all such patients require mechanical ventilation. Some patients with CAP are treated in the ICU because the pneumonia has led to clinical instability of an underlying disease, but the pneumonia itself may not be severe. Bacteremia does not always correlate with more severe illness, and its presence alone is not always a predictor of a poor outcome, with most episodes of bacteremia being due to pneumococcus. However, in the elderly with pneumococcal pneumonia, bacteremia is present in one fourth of patients with CAP and is often associated with azotemia and multilobe involvement.42 When an infection such as pneumonia is complicated by severe sepsis or septic shock (not just bacteremia), outcome is adversely affected, with increases in mortality, length of stay, and costs for survivors.
For nearly 20 years, guidelines have attempted to define when patients should be admitted to the ICU, but the decision is still best made by careful clinical assessment. The 1993 ATS guidelines used the presence of any one of 10 criteria to define the patients who needed ICU admission.1,6 However, subsequent studies showed that 65% of all admitted CAP patients (not needing ICU care) had one of these criteria present, and thus a more specific definition of the need for ICU admission was required.6 Ewig and colleagues evaluated all 10 criteria in a patient cohort and suggested that ICU admission be considered if patients had two of three “minor criteria” present on admission or one of two “major criteria” present on admission or later in the hospital course.6 The minor criteria were systolic blood pressure less than 90 mm Hg, PaO2/FIO2 ratio less than 250, or multilobar infiltrates, while the major criteria were need for mechanical ventilation or septic shock. This definition of need for ICU care had a sensitivity of 78%, a specificity of 94%, a positive predictive value of 75%, and a negative predictive value of 95%. As discussed earlier, another way to identify patients with more severe illness is to apply the BTS rule in its original or modified version. One study found that the use of the revised ATS criteria had a sensitivity of 70.7% and a specificity of 72.4% for predicting need for ICU admission.16 The BTS criteria were much less sensitive with similar specificity, whereas the PORT rule (class IV or V) had similar sensitivity but lower specificity (although this latter rule was very effective at predicting risk of death).
The most recent IDSA/ATS guidelines for CAP suggested that ICU care be considered if the patient had one of two major criteria (need for mechanical ventilation or septic shock with the need for vasopressors), or 3 of 9 minor criteria.1 The minor criteria include: respiratory rate ≥30 breaths/min, PaO2/FIO2 ratio ≤250, multilobar infiltrates, confusion/disorientation, uremia (BUN level >20 mg/dL), leukopenia (WBC count <4000 cells/mm3), thrombocytopenia (platelet count <100,000 cells/mm3), hypothermia (core temperature <36°C), and hypotension requiring aggressive fluid resuscitation. Other factors to consider in the decision making process are hypoglycemia (in a nondiabetic patient), hyponatremia, acute alcohol intoxication, cirrhosis, asplenia, and unexplained metabolic acidosis. The use of these minor criteria to define need for ICU admission requires validation. However, in one study, patients who met only minor criteria for ICU admission did not have an increase in mortality, whereas in another study, presence of four minor criteria was very accurate for defining the need for ICU care.43,44
There is some debate about the benefit of ICU care for patients with CAP, but the benefit seems most certain if patients are admitted early in the course of severe illness, thus emphasizing the need for sensitive criteria to define severe illness. In one recent study, patients with an obvious need for ICU care who were directly admitted to the ICU had a mortality rate of 10.9%, which was significantly lower than the 19.6% mortality rate of those without obvious need for ICU care who had delayed admission.45 The measurement of admission respiratory rate is a simple and reliable assessment, and investigators have observed a linear relationship between admission respiratory rate (once it rose > 30 breaths/min) and mortality.46 If patients are put in the ICU when they meet several “minor” criteria or when they have an elevated respiratory rate, this type of expectant management may have benefits and may keep mortality rates in the 25% to 50% range. This is in marked contrast to the experience in older studies that reported mortality rates above 70% for pneumococcal bacteremia patients admitted to an ICU late in the course of illness, when nearly all admitted patients were mechanically ventilated on arrival to the ICU. In studies of severe CAP with good outcomes, approximately 60% of ICU admitted patients were intubated.4,6
Etiologic Pathogens
Likely Pathogens
Even with extensive diagnostic testing, an etiologic agent is defined in only about half of all patients with CAP, pointing out the limited value of diagnostic testing and the possibility that we do not know all the organisms that can cause CAP.1,41 In the past 4 decades, a variety of new pathogens for this illness have been identified, including L. pneumophila, C. pneumoniae, severe acute respiratory syndrome coronavirus, novel H1N1 influenza, and hantavirus. In addition, antibiotic-resistant variants of common pathogens such as S. pneumoniae have become increasingly common. One of the ways CAP leads to respiratory failure is when it is complicated by ARDS. All of the bacteria and viruses listed here, as well as pneumonia due to aspiration, have been reported to cause ARDS.
The likely pathogens for infection vary depending on patient risk factors for specific microorganisms and the presence of certain comorbid illnesses, but for all patient groups, including those with severe CAP, pneumococcus is the most common pathogen.1 In fact, in one study of lung puncture cultures, this organism was even identified as being common in patients who had no diagnosis established by routine diagnostic testing.47 The incidence of antibiotic-resistant pneumococci has increased in recent years, and up to 40% of these organisms can have reduced sensitivity to penicillin or other antibiotics, although the clinical relevance of in vitro resistance is still uncertain.1,48,49 Identified risk factors for drug-resistant S. pneumoniae (DRSP) include β-lactam therapy in the past 3 months, alcoholism, age older than 65 years, immune suppression, multiple medical comorbidities, and contact with a child in day care.1,50,51 Other common infecting organisms in those with severe CAP include viruses (e.g., influenza, respiratory syncytial virus, and the coronavirus illness of severe acute respiratory syndrome [SARS]), L. pneumophila, M. pneumoniae, M. tuberculosis, and H. influenzae (especially in smokers). In the setting of severe pneumonia, patients can be infected with S. aureus (including methicillin-resistant forms, or MRSA) or enteric gram-negatives and (rarely) anaerobes. In the elderly, including those with aspiration pneumonia, healthcare-associated pneumonia, and in those with underlying cardiopulmonary disease, enteric gram-negative organisms are often seen.
The frequency of gram-negative CAP is difficult to define, but in one study of 559 hospitalized patients with CAP, 60 patients had gram-negative enteric infections, including 39 with P. aeruginosa.1,52 Risk factors for gram-negative organisms were probable aspiration (OR=2.3), previous hospital admission within 30 days of admission (OR=3.5), previous antibiotics within 30 days of admission (OR=1.9), and presence of pulmonary comorbidity (OR=2.8). Risk factors for P. aeruginosa were pulmonary comorbidity (OR=5.8) and previous hospitalization (OR=3.8). Infection with a gram-negative pathogen led to ICU admission and mechanical ventilation more often than infection with other organisms. The mortality rate of CAP due to P. aeruginosa was 28%. In a more recent study from Korea, 10% of 912 CAP patients had gram-negatives, with Klebsiella spp. being twice as common as P. aeruginosa.53 Patients with gram-negatives had a higher mortality than those without, and risk factors for gram-negative infection included septic shock, cardiac disease, smoking, hyponatremia, and dyspnea. Nursing home patients (HCAP) were included in the population of patients studied, again emphasizing the overlap between CAP and HCAP. Another recent study of 3272 episodes of CAP found that 2% were caused by enteric gram-negatives (most commonly P. aeruginosa), and the risk factors for these organisms were COPD, current use of corticosteroids, prior antibiotic therapy, tachypnea ≥ 30/minute, and septic shock on admission.54 Patients with these organisms needed ICU care more often and had a higher mortality and length of stay than those without these pathogens present.
Although aspiration has often been considered a risk factor for anaerobic infection, studies of severe CAP in elderly patients with aspiration risk factors suggested that this population is very likely to have gram-negative infection.55,56 One study evaluated 95 residents of long-term care facilities who had pneumonia requiring ICU admission and risk factors for oropharyngeal aspiration such as swallowing disorders due to neurologic illness, disruption of the gastroesophageal junction, dysphagia, or anatomic abnormalities. Using protected bronchoalveolar lavage (BAL) sampling within 4 hours of admission, a total of 67 pathogens were identified, with enteric gram-negatives in 49%, anaerobes in 16%, and S. aureus in 12%.55 Fifty-five percent of the anaerobes were recovered along with aerobic gram-negative co-infection. The presence of anaerobes did not correlate with oral hygiene but did correlate with functional status, being more common in patients who were totally dependent. Of the seven patients who received inadequate therapy for anaerobes, six recovered, raising a question about whether these organisms really need to be treated. These findings suggest that anaerobes may not really be pathogens but could simply be colonizers in the institutionalized elderly, including those with aspiration risks.55
Primary pulmonary infection with atypical pathogens has been reported for patients with severe CAP for many years. In fact, in one ICU in Spain, atypical pathogens were present in almost 25% of all patients, but the responsible organism varied over time. Legionella was the most common atypical pathogen leading to severe CAP in 14% of patients during one time period, but in the same hospital a decade later, it was seen in only 2%, having been replaced by Mycoplasma and Chlamydophila infection, which were found in 17% of patients compared with only 6% a decade earlier.9,11 Several studies have shown that even if bacterial pathogens lead to CAP, they can be accompanied by atypical pathogens in the form of mixed infection.57,58 Atypical pathogens can include C. pneumoniae, M. pneumoniae, and L. pneumophila, and some recent studies have shown that these infections are common in patients of all ages, not just young and healthy individuals; these organisms have even been reported among the elderly in nursing homes.1,57,59 When mixed infection is present, it may lead to a more complex course and a longer length of stay than if a single pathogen is present, which may explain the increasing number of studies that show a reduction in CAP mortality, including those in the ICU, when initial therapy provides coverage for these organisms, compared with regimens that do not provide coverage.60,61 Interestingly, multiple retrospective studies of pneumococcal bacteremia have shown a reduced mortality when dual therapy (usually involving a macrolide) rather than monotherapy is used, raising the possibility that even these patients have mixed infection with atypical pathogens.62,63 The frequency of atypical pathogens can be as high as 60%, with as many as 40% of all CAP patients having mixed infection.58 These high incidence numbers have been derived with serologic testing, which is of uncertain accuracy.
Atypical organism pneumonia may not be a constant phenomenon, and the frequency of infection may vary over the course of time and with geography. In fact, one study showed that the benefit of providing empirical therapy directed at atypical pathogens was variable, being more important in some calendar years than in others.61 The incidence of Legionella infection among admitted patients has varied from 1% to 15% or more and is also a reflection of geographic and seasonal variability in infection rates, as well as a reflection of the extent of diagnostic testing.
In the past, S. aureus was an uncommon cause of CAP, but it was capable of leading to severe pneumonia. In the past several years, a community-acquired strain of MRSA (CA-MRSA) has emerged as a cause of severe CAP, particularly in patients without a history of previous hospitalization or chronic illness, often as a complication of influenza infection.1,64,65 The organism can lead to a severe bilateral necrotizing pneumonia, often related to toxin production by the organism. This organism is distinct from the nosocomial strain of MRSA and is clonal in origin, usually due to the USA-300 strain.
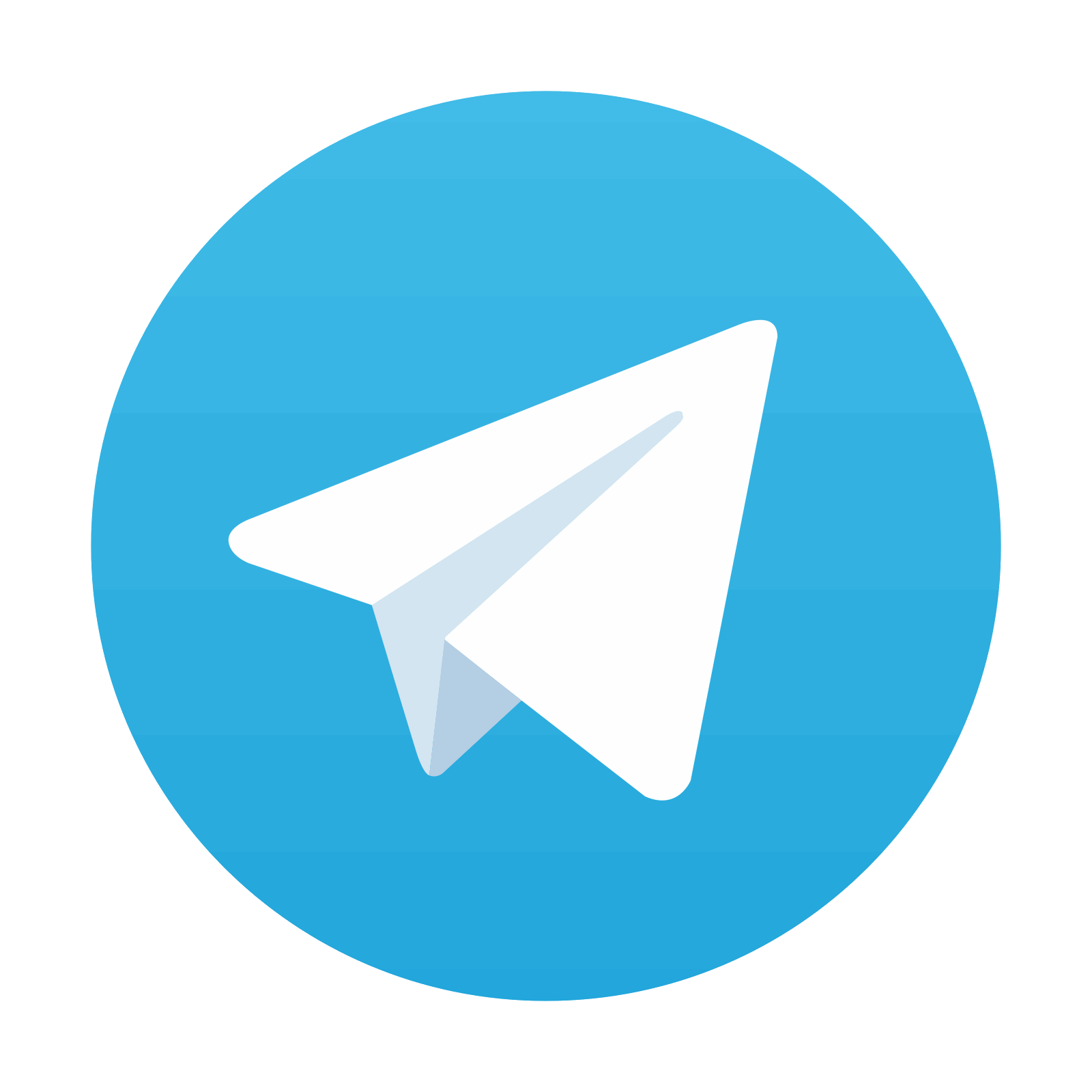
Stay updated, free articles. Join our Telegram channel

Full access? Get Clinical Tree
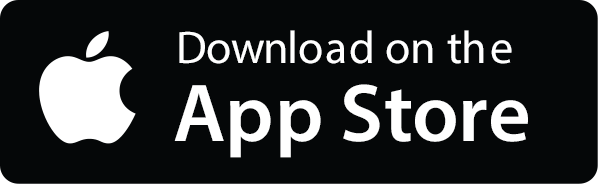
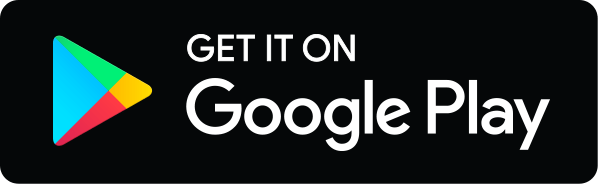