Anticoagulant agents
Agent
Mechanism of action
Emergency reversal
Apixaban
Factor Xa inhibitor
*No antidote*
Administer activated charcoal if last ingested within 3 h
Consider PCC4 50 units/kg (max dose 5000 units) if bleed is life threatening
Dabigatran
Thrombin (factor II) inhibitor
Idarucizumab 5 g (2.5 mg doses 15 min apart). May administer a second 5 g dose if relevant bleeding continues
Renal replacement therapy can be utilized to reduce the duration of bleeding but should not be used for emergent reversal due to time constraints
Edoxaban
Factor Xa inhibitor
*No antidote*
Consider PCC4 50 units/kg (max dose 5000 units) if bleed is life threatening
Enoxaparin
Factor II and Xa inhibitor
Administer protamine to partially reverse (~60 %) the anticoagulant effects
≤8 h since last dose: 1 mg protamine for each 1 mg of enoxaparin
>8 h since last dose: 0.5 mg protamine for each 1 mg of enoxaparin
>12 h since last dose: protamine will not be effective; do not administer
Fondaparinux
Factor Xa inhibitor
*No antidote*
Consider rFVIIa 40 μg/kg if bleed is life threatening
Rivaroxaban
Factor Xa inhibitor
*No antidote*
Administer activated charcoal if last ingested within 8 h
Consider PCC4 50 units/kg (max dose 5000 units) if bleed is life threatening
Warfarin
VKORC antagonist (inhibits clotting factors II, VII, IX, and X)
Administer vitamin K 10 mg IV in all patients where reversal is needed
Administer FFP in addition to vitamin K for all life-threatening bleeds besides ICH
Administer PCC4 in addition to vitamin K as first line for ICH and if above measures are ineffective for all other bleeds that are life threatening
INR 2.0–3.9: 25 units/kg (max dose 2500 units)
INR 4.0–6.0: 35 units/kg (max dose 3500 units)
INR >6.0: 50 units/kg (max dose 5000 units)
Antiplatelet agents
Agent
Mechanism of action
Emergency reversal
Aspirin
Thromboxane A2 inhibitor
*No antidote*
Administer platelet transfusion
Consider DDAVP 0.3 μg/kg IV
Clopidogrel
Prasugrel
Ticagrelor
P2Y12 inhibitors
*No antidote*
Administer platelet transfusion
Consider DDAVP 0.3 μg/kg IV
The first approved DOAC, dabigatran, is an oral selective direct thrombin inhibitor. Dabigatran has a mean elimination half-life of 12–17 h with peak effects occurring 2 h after a dose is given [11]. Like all direct thrombin inhibitors, dabigatran will have noticeable effects on common coagulation tests like prolonging the PT and aPTT [12]. However, these tests are unreliable in providing quantitative assessments of anticoagulation [11]. In clinical trials, dabigatran was associated with similar rates of clinically relevant bleeding as warfarin, but rates of major gastrointestinal bleeding may be higher with dabigatran [13, 14]. Dabigatran’s pharmacodynamics effects depend primarily on age and renal function. It is removed primarily through renal elimination as an unchanged drug and, as a result, requires a dose adjustment in renal insufficiency [15]. A study in elderly subjects aged 65–87 years receiving dabigatran showed that the area under the concentration curve doubles in this population as compared to younger adults with more pronounced effects seen in elderly women [11].
Rivaroxaban is a direct oral factor Xa inhibitor. It is metabolized via the cytochrome P450 system with approximately 66 % of the drug then excreted through the kidneys [16]. However, renal function appears to have only a moderate effect on elimination half-life most likely due to alternate clearance pathways [17]. The elimination half-life is also shorter than dabigatran at 6–7 h with peak effects occurring 2 h after administration [18]. Phase III clinical trials with rivaroxaban found no difference in major bleeding events or clinical relevant bleeding as compared to existing anticoagulants [19, 20]. There appears to be less significant pharmacokinetic differences in the elderly associated with rivaroxaban and with no reported gender differences [21, 22]. Rivaroxaban has dose-dependent effects on the PT and aPTT, but the tests are not validated for quantitative use because of variability in testing materials [22, 23].
Apixaban is another direct oral factor Xa inhibitor that was recently approved for use in the United States. It is the only DOAC agent that has proven superior to warfarin in the incidence of spontaneous major bleeding and clinically relevant bleeding [24]. Apixaban has an effective half-life of about 12 h, and due to slower absorption, it takes longer for a peak effect to occur at 3–4 h after the dose [25]. As is the case with all DOAC agents, the anticoagulant effect of apixaban cannot be quantified using traditional laboratory measures like the aPTT or INR. Chromogenic anti-Xa assays do exist to measure the concentration of Xa inhibitors, but they are not readily commercially available [26]. Apixaban is primarily cleared through renal elimination and requires a dosage adjustment in renal insufficiency. Age- and sex-related differences also exist with the pharmacokinetics of apixaban with the elderly and female population having a moderately increased exposure [27].
Edoxaban, a direct factor Xa inhibitor, is the most recently approved DOAC agent in the United States. This agent is unique in that it has a rapid time to peak effect of 1.5 h while also displaying a long elimination half-life of 10–14 h [28]. Edoxaban displays major bleeding rates similar to that of warfarin and other DOAC agents despite being more effective at reducing some coagulation markers [29–31]. A small phase I trial was able to show that traditional laboratory measures like the PT, INR, and anti-FXa activity correlate closely with edoxaban plasma concentrations, but further validation of this result is needed before it should be relied upon in clinical practice [32]. The elimination half-life of edoxaban is prolonged in patients with renal failure, but no other altered pharmacokinetics have been reported in the elderly population. Pharmacokinetic studies have shown that hemodialysis is not effective at removing edoxaban from the blood and it should not be used as a means of reversal in a trauma scenario [33].
Antiplatelet Agents
Aspirin, clopidogrel, prasugrel, and ticagrelor are commonly used agents which decrease platelet activation and aggregation via glycoprotein IIb/IIIa. The effect of pre-injury antiplatelet agents on mortality in TBI patients has been studied with varying results. A recent retrospective analysis of 1552 patients found that pre-injury use of aspirin alone, clopidogrel alone, and a combination of the two did not result in increased mortality as compared to patients on no antithrombotic agent [7]. This result has been reinforced with a large meta-analysis showing only a minor effect on survival with pre-injury antiplatelet agents that did not meet statistical significance [34]. The effect of these agents on other outcomes such as the severity of bleeding and need for neurosurgical intervention is not as well defined. Thus, reversal is still warranted in many cases as it is unlikely that antiplatelet therapy is completely benign in a traumatic bleed [35–40]. Combination therapy with antiplatelet agents is common, and this has been shown to have an additive effect on the degree of platelet inhibition [41].
There are numerous assays currently marketed that test response to antiplatelet agents. However, current cardiology literature does not support antiplatelet testing to individualize therapy given the variability between assays and lack of established reference ranges. One study in trauma patients examined point-of-care testing for patients with a reported history of taking clopidogrel. The results confirmed previous literature outside of trauma populations, in that large variability between patients exists [42]. Viscoelastic testing is becoming commonplace in the setting of traumatic hemorrhage management, but this also has a lack of sensitivity to detect platelet dysfunction due to antiplatelet agents [43]. To date, antiplatelet testing cannot be used to reliably guide therapy in the management of traumatic hemorrhage.
Reversal of Pre-injury Antiplatelet and Anticoagulant Agents (Table 39.1)
Platelet transfusion is commonly employed in the management of traumatic intracranial hemorrhage in patients on pre-injury antiplatelet therapy, despite a lack of data to support its efficacy. Both aspirin and clopidogrel bind irreversibly to platelets causing dysfunction for the life of the platelet. If transfusion is to be beneficial, it would only do so by providing additional functional platelets. Two retrospective studies have shown no benefit on either morbidity or mortality [44, 45]. A meta-analysis of intracerebral hemorrhage (ICH) patients was also unable to show that platelet transfusion was beneficial [46]. One explanation for the lack of efficacy with platelet transfusion is that recent ingestion of an antiplatelet agent may quickly inactivate the infused platelets. Ultimately, early platelet transfusion is still recommended in patients with substantial bleeding who are on pre-injury antiplatelet therapy despite the lack of evidence supporting its efficacy.
Extrapolating data from other patient populations, several guidelines and reviews have suggested using desmopressin (DDAVP) to reverse platelet dysfunction although it has not been studied in trauma patients [47]. DDAVP is known to increase concentrations of von Willebrand factor and factor VIII thus enhancing platelet adherence. A meta-analysis of clinical trials found a small reduction in blood transfusion requirements in the postoperative period, though these were not urgent procedures that would be seen in the setting of trauma. It is suggested that DDAVP be used at doses of 0.3–0.4 μg/kg intravenously [48].
Vitamin K is the antidote for warfarin toxicity, and when administered intravenously, reversal effects can be seen within 12–24 h [49]. However, rapid reversal of the anticoagulant effects of warfarin has been shown to decrease progression of ICH and mortality [50]. Administration of fresh frozen plasma (FFP) can begin to reverse coagulopathy by supplying clotting factors II, VII, IX, and X while awaiting the full effects of reversal with vitamin K. While a targeted therapy, FFP use is fraught with limitations: optimal dosing is unknown, clotting factor concentrations are variable, the required volumes of FFP to be infused may be problematic for fluid-sensitive patients, it requires thawing prior to administration, and time to correction of INR is highly variable.
Prothrombin complex concentrates (PCCs) and recombinant activated factor VIIa (rFVIIa) may also be beneficial for the emergent reversal of warfarin. Three-factor PCCs contain factors II, IX, and X with little to no factor VII. Four-factor PCCs contain high concentrations of factors II, VII, IX, and X. PCCs provide replacement of carboxylated clotting factors and appear to be the only available option for immediate reversal of warfarin despite a lack of robust data for their use. Recent guidelines for anticoagulant reversal from the American College of Chest Physicians suggest four-factor PCC for patients with life-threatening warfarin-associated bleeding over plasma infusion [51, 52]. PCC agents and the clotting factors they replace have a significantly shorter half-life than that of warfarin, so combination therapy with vitamin K should still be utilized.
The introduction of the DOAC agents presents the trauma clinician with distinct new challenges in the setting of an emergency. Data from prospective phase III trials and a combined meta-analysis have suggested that there may be a lower risk of bleeding with these newer agents compared to warfarin [53]. However, there are limited options available for reversal of these agents, making a traumatic bleed associated with one of them problematic. Traditional reversal agents such as vitamin K, FFP, protamine, and cryoprecipitate have been proven ineffective. Idarucizumab was recently approved and is the first specific reversal agent for a DOAC agent. It was found to completely reverse the anticoagulant effects of dabigatran in patients with life-threatening bleeding or those requiring urgent surgery [54]. When dabigatran reversal is needed, clinicians also have the option of utilizing hemodialysis as it has been shown to remove up to 62 % of the drug in a 2-h session [55]. Though this is likely not useful in the case of an emergency bleed because of time constraints, renal replacement therapy has been shown to reduce the duration and severity of bleeding [56]. Unfortunately, the more commonly used DOAC agents, rivaroxaban and apixaban, lack a specific reversal agent, so nonspecific agents must be utilized.
It has been suggested that PCCs and rFVIIa may have a role in reversing the anticoagulant effects of DOAC agents in cases of severe bleeding by increasing the plasma concentration of the clotting factors they inhibit. However, studies supporting their use have been limited to animal studies or human studies that were severely limited by sample size and were with healthy volunteers [57–62]. These in vivo and in vitro studies have presented mixed results with no convincing evidence of benefit. Despite this, numerous guidelines still suggest reversal with PCCs in cases of emergent bleeding [63, 64]. Specific reversal agents for factor Xa inhibitors are being investigated and have shown promise in their early stages [65, 66].
Beta-Blocker Therapy
Beta-blockers (BB) are commonly prescribed in elderly trauma patients for blood pressure, heart rate, or arrhythmia control [67–71]. Studies have shown survival benefits for patients receiving BB therapy for cardiac and high-risk vascular procedures [68, 69]. For nonvascular operations, the data for BB use is mixed, where the cardiac events are less, but the mortality rates are not improved compared to those treated perioperatively for cardiac or vascular procedures [67–71].
Nonrandomized, cohort controlled studies in both adult burn and trauma patients have suggested potential benefits [72–77]. Effects of BB include decreased cardiac oxygen consumption and hypermetabolism [74]. BB also can decrease systemic and cerebral perfusion pressure [78]. Theorized mechanisms for BB effects may be secondary to suppression of IL-6 which has been associated with increased mortality for trauma and sepsis patients. A small, randomized trial of patients treated with BB found lower IL-6 levels [76]. In a study comparing older patients who were case matched for age, Injury Severity Score, Glasgow Coma Scale score and mechanism of injury, BB use decreased mortality [73]. A similar study in adult burn patients showed faster rates of healing and time to discharge for those on BB [72]. Patients in these studies who arrived to the hospital on BB were older and more severely injured [72, 73].
A subsequent retrospective study, which did not include cohorts or the use of case matching in the study design, found that patients admitted on BB without head injury had higher mortality than those admitted without BB therapy [79]. While for the overall patient population admitted on BB there was no difference in mortality, those admitted on BB appeared to have more warfarin use and vascular disease [79], suggesting that BB therapy may have been beneficial for those with head injury since mortality was not higher. Another group studied the use of BB in trauma patients with severe traumatic brain injury (TBI) and found a survival benefit. While not all patients in the severe TBI study were elderly, the mean age of the BB group was 50 compared to 36 years in those without BB use. Despite the significantly older age of those receiving BB therapy during hospitalization, the BB-treated TBI patients had improved survival compared to patients not treated with BB [80].
The former studies posed the question that BB may have caused injured patients to appear less ill due to less tachycardia at presentation and that this may have affected their subsequent resuscitation. Alternatively, BB use in the elderly may have been a marker for patients with more systemic illness at baseline, which would make the findings of lower mortality even more compelling [79, 80]. In a subsequent study, which looked at patients with isolated TBI, researchers found that patients admitted on BB were older, had more severe TBI, had more skull fractures, and required operative intervention more frequently, but had lower mortality than those who did not receive BB therapy [81]. Beta-blockade was independently associated with improved survival [81].
Despite the positive findings in retrospective, nonrandomized studies, there is still concern over the potential side effects of BB therapy from vasoconstriction and bradycardia due to antagonism of beta-mediated vasodilation and increased risk of vasovagal reaction. Early in hemorrhagic shock, the effects of BB may mask early recognition of shock, and the provider should seek other signs of poor perfusion such as acidosis, decreased urine output, and altered mentation since hypotension is a later manifestation of shock. One retrospective study which looked at the use of pre-injury BB, angiotensin-converting enzyme inhibitors (ACE-I) or angiotensin receptor blockers (ARB), calcium channel blockers, or amiodarone found no demonstrable effect on systolic or diastolic blood pressures in trauma patients presenting on these individual medications. However, patients who received the combination triple therapy of a BB, ACE-I/ARB, and calcium channel blocker had higher mortality and inhospital complications despite only mild changes in the initial hemodynamic profile at admission [82]. The need for triple therapy may be a marker for more severe underlying comorbidities in the patients requiring these medications, given that 22 % of complications in these patients were cardiac in nature [82].
Delirium and Management in the Elderly
Unlike dementia which is a chronic confusional state, delirium is an acute confusional state which occurs most commonly in older, hospitalized patients [83]. Acute brain dysfunction, or delirium, occurs in up to 70 % of mechanically ventilated patients in the surgical ICU [85–87] and recently has been reported in a similar proportion of trauma ICU patients [83–85]. Delirium has been identified in 15–53% of older patients undergoing operation [88, 89] and is associated with mortality rates of 22–76% [90], rivaling mortality rates of sepsis and acute myocardial infarction [84, 91]. The clinical manifestations of delirium vary from hypoactive with withdrawal and decreased motor activity to hyperactive with agitation, increased arousal, and aggression. Delirium is reportedly underdiagnosed in more than half of cases largely due to the under-recognition of the hypoactive form and attributing symptoms to dementia [92].
Evidence suggests that delirium may be secondary to altered neurotransmission, inflammation, and even chronic stress [93]. Administration of anticholinergic drugs has been shown to cause delirium in both animals and humans. Excess dopaminergic activity may also contribute to delirium as a regulator of acetylcholine, which supports the use of antipsychotic agents for treatment of delirium symptoms [83, 93].
With the potential for inflammation and alterations in neurotransmitters contributing to delirium, it is not unexpected that surgical procedures would potentially increase the incidence of delirium. In a Canadian study looking at over 19,000 patients aged 65 years or older undergoing elective surgery, 16 % of the elderly patients developed delirium. The biggest predictor for development of delirium was the duration of surgery, which accounted for about 44 % of the risk, but unique to this study was the finding that preoperative statin use accounted for a 28 % increase in delirium [94]. Other contributing factors to delirium are common in the postoperative setting. Multiple studies have concluded that exposure to sedatives and analgesics is associated with delirium [85–87, 95–97]. Not only are continuous sedative infusions contributors to higher rates of delirium, but they are also associated with increased mechanical ventilator days and intensive care unit lengths of stay [96]. In a study of patients with a hip fracture and an average age of 81 years, patients treated with fracture fixation under spinal anesthesia and a light level of sedation had 50 % less postoperative delirium than those managed at a deeper level of sedation during the procedure [98].
To confront the issues of continuous sedation increasing the risk of adverse outcomes, some recent studies have looked at changing how ventilated patients are managed, addressing pain first [85, 87]. If after treating with pain medications, a patient is still agitated, then delirium is addressed. Sedative medications should only be added if a patient is unable to be maintained safely on the ventilator or is at risk of removing other vital tubes or drains without sedation. This approach has gained significant traction in critical care organizations [99] and requires objective evaluation of patients with standardized tools which assess for pain and delirium screens such as the Confusion Assessment Method for the Intensive Care Unit (CAM-ICU) score [100]. If patients screen positive for delirium, non-pharmacologic treatment should be attempted. Review of medications which may have sedative properties or cognitive side effects should be eliminated or the dose minimized, if possible. Metabolic causes for delirium should be corrected such as hypoxia, electrolyte disturbances, and hypothyroidism. Underlying infection should be considered and appropriate testing done to exclude this possibility. If the patient has hearing or visual aids, these should be in place to improve the ability to communicate and orient the patient. Non-pharmacologic methods such as relaxation, music, restoration of the normal sleep-wake cycle with avoidance of loud noise, and bright lights during sleep should be employed as much as possible [84, 87, 97, 101].
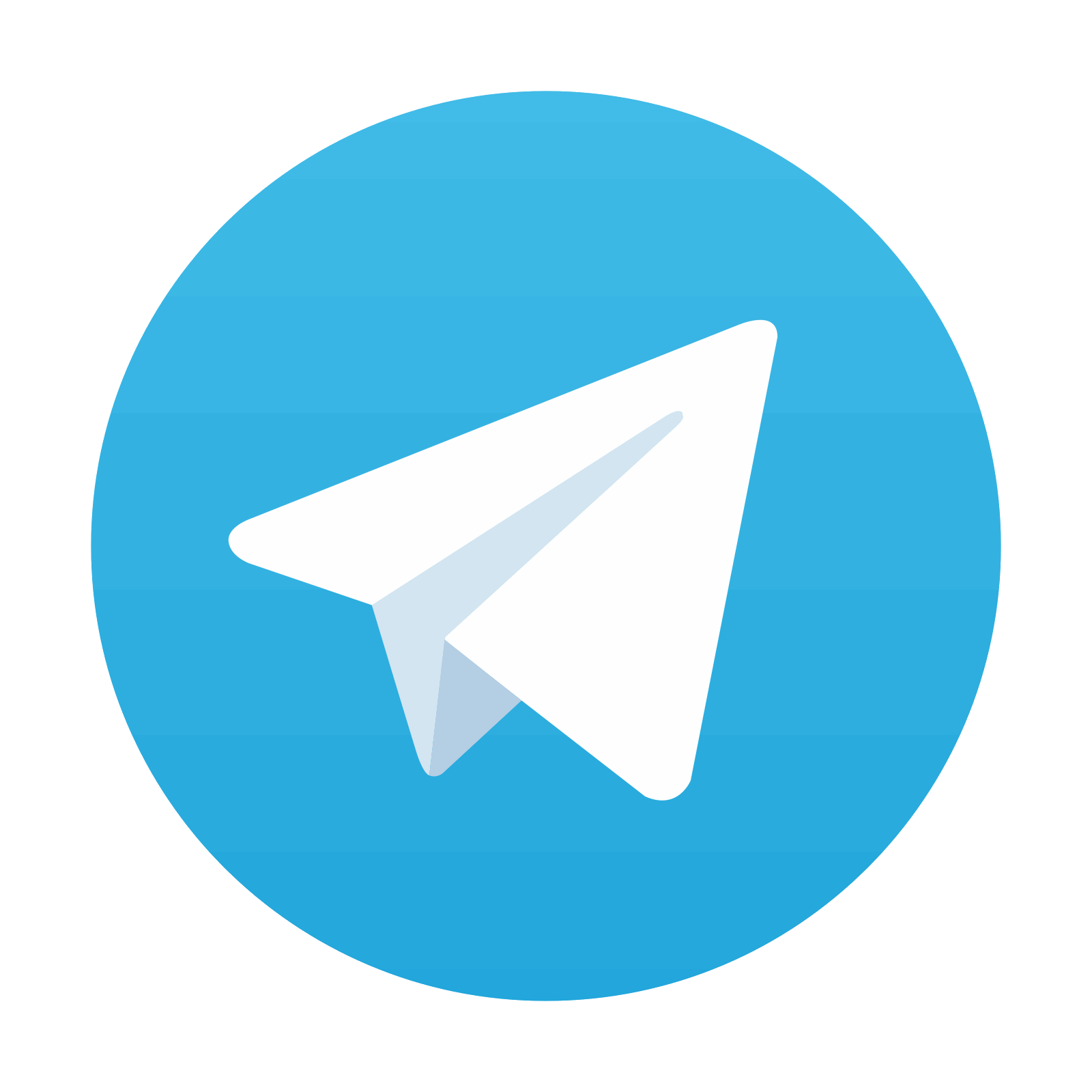
Stay updated, free articles. Join our Telegram channel

Full access? Get Clinical Tree
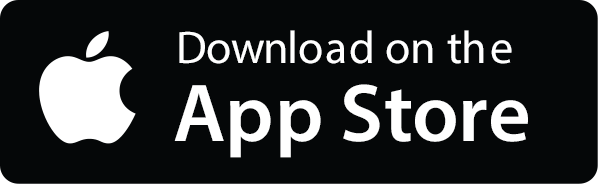
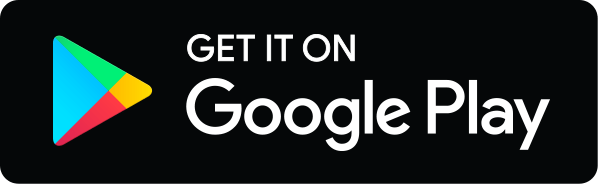