32 Coma
Anatomy, Pathology, Pathophysiology
Consciousness depends upon an intact ARAS in the brainstem and adjacent thalamus, which acts as the alerting or awakening element of consciousness, together with a functioning cerebral cortex of both hemispheres, which determines the content of that consciousness.1,2 The ARAS lies within a more or less isodendritic core that extends from the medulla through the tegmentum of the pons to the midbrain and paramedian thalamus. The system is continuous caudally with the reticular intermediate gray matter of the spinal cord and rostrally with the subthalamus, hypothalamus, anterior thalamus, and basal forebrain.3 The ARAS itself arises within the rostral pontine tegmentum and extends across the mesencephalic tegmentum and its adjacent intrathalamic nuclei. ARAS functions and interconnections are considerable and likely contribute more than only a cortical arousal system. The specific role of the various links from the reticular formation to the thalamus has yet to be fully identified.4 Furthermore, the cortex feeds back on the thalamic nuclei to contribute an important loop that amplifies arousal mechanisms.5,6
The ascending arousal system contains cholinergic, monoaminergic, and γ-amino butyric acid (GABA) systems, none of which has been identified as the arousal neurotransmitter.2,7,8 Acute structural damage to, or metabolic/chemical disturbance of, either the ascending brainstem-thalamic activating system or the thalamocorticothalamic loop can alter the aroused attentive state. Consciousness depends on continuous interaction between the mechanisms that provide arousal and awareness. The brainstem and thalamus provide the activating mechanism, and the cerebrum provides full cognition and self-excitation. Content of consciousness is best regarded as the amalgam and integration of all cognitive function that resides in the thalamocortical circuits of both hemispheres. Altered awareness is due to disruption of this cortical activity by diffuse pathology. Focal lesions of the cerebrum can produce profound deficits such as aphasia, alexia, amnesia, and hemianopsia, but only diffuse bilateral damage sparing the ARAS and diencephalon can lead to wakeful unawareness. Thus there are two kinds of altered consciousness: (1) altered arousal due to dysfunction of the ARAS-diencephalon and (2) altered awareness due to bilateral diffuse cerebral hemisphere dysfunction.
Four major pathologic processes can cause such severe global, acute reductions of consciousness.1,9 (1) In the presence of diffuse or extensive multifocal bilateral dysfunction of the cerebral cortex, the cortical gray matter is diffusely and acutely depressed or destroyed. Concurrently, cortical-subcortical physiologic feedback excitatory loops are impaired, with the result that brainstem autonomic mechanisms become temporarily profoundly inhibited, producing the equivalent of acute “reticular shock” below the level of the lesion. (2) Direct damage to a paramedian upper brainstem and posterior-inferior diencephalic ascending arousal system blocks normal cortical activation. (3) Widespread disconnection between the cortex and subcortical activating mechanisms acts to produce effects similar to both conditions 1 and 2. (4) Diffuse disorders, usually metabolic in origin, concurrently affect both the cortical and subcortical arousal mechanisms, although to a different degree according to the cause.
Structural Lesions Causing Coma
Two herniation syndromes demonstrate the mechanism by which supratentorial lesions produce coma. The rate of evolution of a mass dictates whether the anatomic distortion precedes (in slowly evolving lesions) or parallels the patient’s deterioration of wakefulness. Transtentorial herniation can be central or predominantly unilateral. Central herniation results from caudal displacement by deep midline supratentorial masses, large space-occupying hemisphere lesions, or large uni- or bilateral compressive extraaxial lesions with compression of the ARAS. The progressive rostral-caudal pathologic and clinical stages of this herniation syndrome were outlined.1 Pathologically, bilateral symmetric displacement of the supratentorial contents occurs through the tentorial notch into the posterior fossa. Alertness is impaired early, pupils become small (to 3 mm) and reactive, and bilateral upper motor neuron signs develop. Cheyne-Stokes breathing, grasp reflexes, roving eye movements, or depressed escape of oculocephalic reflexes are the clinical manifestations. In the absence of effective therapy at this diencephalic stage, herniation progresses caudally to compress the midbrain, leading to a deep coma and fixed midposition (3-5 mm) pupils, signifying both sympathetic and parasympathetic interruption. Spontaneous eye movements cease, and oculovestibular and oculocephalic reflexes become difficult to elicit. Spontaneous extensor posturing may occur. Once this stage is reached, full recovery becomes unlikely. As the caudal compression-ischemia process advances, pontine and medullary function becomes destroyed, with variable breathing patterns and absent reflex eye movements. Finally, autonomic cardiovascular and respiratory functions cease as medullary centers fail.
Uncus herniation results from laterally placed hemisphere lesions, particularly of the temporal lobes, that cause side-to-side cerebral displacement as well as transtentorial herniation. Focal hemisphere dysfunction (hemiparesis, aphasia, seizures) precedes unilateral (usually ipsilateral) compression paralysis of the third cranial nerve. An early sign of uncus herniation is an ipsilateral (rarely contralateral) enlarged pupil that responds sluggishly to light, followed by a fixed, dilated pupil and an oculomotor palsy (eye turned downward and outward).1 The ipsilateral posterior cerebral artery can become compressed as it crosses the tentorium and causes ipsilateral occipital lobe ischemia. Progressively, the temporal lobe compresses the midbrain, with loss of arousal and bilateral or contralateral extensor posturing. Ipsilateral to the intracranial lesion, a hemiparesis may develop if the opposite cerebral peduncle becomes compressed against the contralateral tentorial edge (Kernohan notch). Abnormal brainstem signs become symmetric, and herniation proceeds in the same pattern seen with central herniation as rostrocaudal brainstem displacement progresses.
Extraaxial posterior fossa lesions cause coma by direct compression of the ARAS in the brainstem, and in the diencephalon by upward transtentorial herniation. Compression of the pons may be difficult to distinguish from intrinsic lesions but is often accompanied by headache, vomiting, and hypertension due to a Cushing reflex. Upward herniation at the midbrain level is initially characterized by coma, reactive miotic pupils, asymmetrical or absent caloric eye responses, and decerebrate posturing; caudal-rostral brainstem dysfunction then occurs, with midbrain failure and midposition fixed pupils.10 Causes of brainstem compression include cerebellar hemorrhage, infarction and abscess, rapidly expanding cerebellar or fourth-ventricle tumors, or less commonly, infratentorial epidural or subdural hematomas. Drainage of the lateral ventricles to relieve obstructive hydrocephalus due to posterior fossa masses can potentially precipitate acute upward transtentorial herniation.11,12
Downward herniation of the cerebellar tonsils through the foramen magnum causes acute medullary dysfunction and abrupt respiratory and circulatory collapse. Less severe impaction of the tonsils in the foramen magnum can lead to obstructive hydrocephalus and consequent bihemispheric dysfunction with altered arousal. Clinical manifestations include headache, nausea, vomiting, lower cranial nerve signs, vertical nystagmus, ataxia, and irregular breathing. Lumbar puncture in this setting carries a risk of catastrophic consequences.11
Nonstructural Causes of Coma
The pathophysiology of other metabolic encephalopathies is less well established and is extensively discussed elsewhere.1 Hepatic encephalopathy is caused not merely by ammonia intoxication but likely also involves accumulation of neurotoxins such as short-chain and medium-chain fatty acids, mercaptans, and phenols. Altered neurotransmission may play a role with accumulation of benzodiazepine-like substances, imbalance of serotonergic and glutaminergic neurotransmission, and accumulation of false neurotransmitters. The identity of the neurotoxin in uremic encephalopathy is uncertain and includes urea itself, guanidine and related compounds, phenols, aromatic hydroxyacids, amines, various peptide “middle molecules,” myoinositol, parathormone, and amino acid imbalance. The cause of the dysequilibrium syndrome may entail more than osmotic water shifts from plasma into brain cells, and reduction is reported in cortical potassium, with intracellular acidosis due to increased production of organic acids in the brain. The pathogenesis of pancreatic encephalopathy may involve patchy demyelination of brain white matter due to liberated enzymes from a damaged pancreas, disseminated intravascular coagulation, or fat embolism.
Differential Diagnosis
The vegetative state can be defined as wakefulness without awareness and is the consequence of various diffuse brain insults.1,13 It may be a transient phase through which patients in coma pass as the cerebral cortex recovers more slowly than the brainstem. Clinically, vegetative patients appear to be awake and to have cyclical sleep patterns; however, such individuals do not show evidence of cognitive function or learned behavioral responses to external stimuli. Vegetative patients may exhibit spontaneous eye opening, eye movements, and stereotypic facial and limb movements, but they are unable to demonstrate speech or comprehension, and they lack purposeful activity. Vegetative patients generate normal body temperature and usually have normally functioning cardiovascular, respiratory, and digestive systems, but they are doubly incontinent. The vegetative state should be termed persistent at 1 month after injury and permanent at 3 months after nontraumatic injury or 12 months after traumatic injury.14,15 Extended observation of the patient is required to assess behavioral responses to external stimulation and demonstrate cognitive unawareness. The EEG is never isoelectric but shows various patterns of rhythm and amplitude, inconsistent from one patient to the next. Normal EEG sleep/wake patterns are absent.
In the locked-in syndrome, patients retain or regain arousability and self-awareness, but because of extensive bilateral paralysis (i.e., de-efferentation) can no longer communicate except in severely limited ways. Such patients suffer bilateral ventral pontine lesions with quadriplegia, horizontal gaze palsies, and lower cranial nerve palsies. Voluntarily they are capable only of vertical eye movements and/or blinking.1 Sleep may be abnormal, with marked reduction in non-REM and REM sleep phases. The most common etiology is pontine infarction due to basilar artery thrombosis, but others are pontine hemorrhage, central pontine myelinolysis, and brainstem mass lesions. Neuromuscular causes of locked-in syndrome include severe acute inflammatory demyelinating polyradiculoneuropathies, myasthenia gravis, botulism, and neuromuscular blocking agents. In these peripheral disorders, upward gaze is not selectively spared.
Akinetic mutism describes a rare subacute or chronic state of altered behavior in which an alert-appearing patient is both silent and immobile but not paralyzed.16 External evidence of mental activity is unobtainable. The patient usually lies with eyes opened and retains cycles of self-sustained arousal, giving the appearance of vigilance. Skeletal muscle tone can be normal or hypertonic but usually not spastic. Movements are rudimentary even in response to unpleasant stimuli. Affected patients are usually doubly incontinent. Lesions that cause akinetic mutism may vary widely. One pattern consists of bilateral damage to the frontal lobe or limbic-cortical integration with relative sparing of motor pathways. Vulnerable areas involve both basal medial frontal areas. Somewhat similar behavior also can follow incomplete lesions of the deep gray matter (paramedian reticular formation of the posterior diencephalon and adjacent midbrain), but such patients usually suffer double hemiplegia and act slowly yet are not completely akinetic or noncommunicative.
Approach to Coma
Emergency Management
The key points of the rapid neurologic exam are: hand drop from over the head (to assess for malingering or hysterical loss of consciousness); pupillary size and response to light; abnormal eye movements (active disconjugate, unilaterally paralytic, passively induced, or absent); grimacing/withdrawal from noxious stimulation; and abnormal plantar response (unilateral or bilateral Babinski sign).17 Assisted ventilation should continue during the examination if necessary. Neuromuscular blockade required for patient management and care should be deferred if possible until the neurologic examination is completed (3-5 minutes). Signs of arousal or inadequate sedation include dilated reactive pupils, copious tears, diaphoresis, tachycardia, systemic hypertension, and increased pulmonary artery pressure. Thereafter, monitoring patients neurologically may require head computed tomography (CT) more frequently.
Maintain circulation to assure adequate cerebral perfusion. Appropriate resuscitation fluid is lactated Ringer’s solution; normal saline is also used when intracranial hypertension is suspected. A mean arterial pressure around 100 mm Hg is adequate and safe for most patients. While obtaining venous access, collect blood samples for anticipated tests (Box 32-1). Treat hypotension by replacing any blood volume loss, and use vasoactive agents. Judiciously manage systemic hypertension with hypotensive agents that do not substantially raise ICP by their vasodilating effect (labetalol, hydralazine, or a titrated nitroprusside infusion are the favored agents for managing uncontrollable hypertension). For most situations, systolic blood pressure should not be treated unless it is above 160 mm Hg. Maintain urine output at least 0.5 mL/kg/h; accurate measurement requires bladder catheterization.
Consider specific antidotes: Drug overdose is the largest single cause (30%) of coma in the emergency room. Most drug overdose can be treated by supportive measures alone. However, certain antagonists specifically reverse the effects of coma-producing drugs. Naloxone (0.4-2 mg, IV) is the antidote for opiate coma. The reversal of narcotic effect, however, may precipitate acute withdrawal in an opiate addict. In suspected opiate coma, the minimum amount of naloxone should be administered to establish the diagnosis by pupillary dilatation and to reverse respiratory depression and coma. Do not attempt to reverse completely all drug effects with the first dose. IV flumazenil reverses all benzodiazepine-induced coma. Coma unresponsive to 5 mg flumazenil in divided doses given over 5 minutes is not due to benzodiazepine overdose. Recurrent sedation can be prevented with flumazenil (1 mg IV) every 20 minutes.18 The sedative effects of drugs with anticholinergic properties, particularly tricyclic antidepressants, can be reversed with physostigmine (1-2 mg IV). Pretreatment with 0.5 mg atropine will prevent bradycardia. Only full awakening is characteristic of an anticholinergic drug overdose, as physostigmine has nonspecific arousal properties. Physostigmine has a short duration of action (45-60 minutes), and doses may have to be repeated.
Adjust body temperature: hyperthermia is dangerous because it increases brain metabolic demand and, at extreme levels, denatures brain proteins.19 Hyperthermia greater than 40°C requires nonspecific cooling measures even before the underlying etiology is determined and treated. Hyperthermia most often indicates infection but may be due to intracranial hemorrhage, anticholinergic drug intoxication, or heat exposure. A body temperature of less than 34°C should be slowly increased to above 35°C to prevent cardiac dysrhythmia. Hypothermia accompanies profound sepsis, sedative-hypnotic drug overdose, drowning, hypoglycemia, or Wernicke encephalopathy.
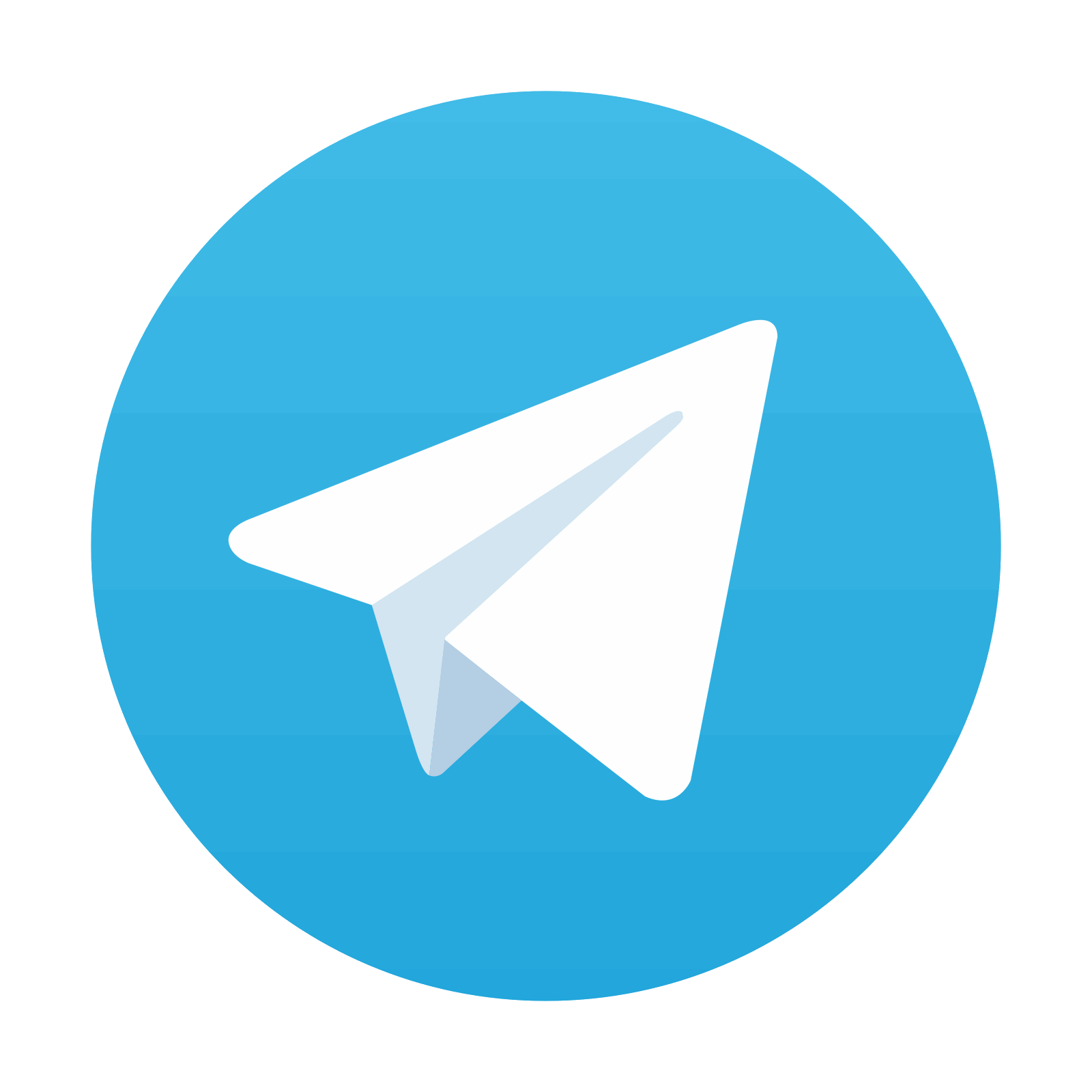
Stay updated, free articles. Join our Telegram channel

Full access? Get Clinical Tree
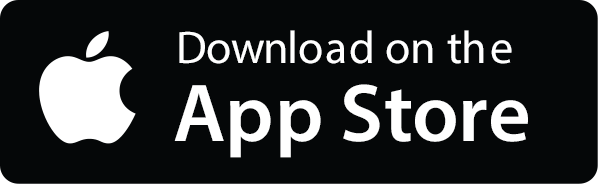
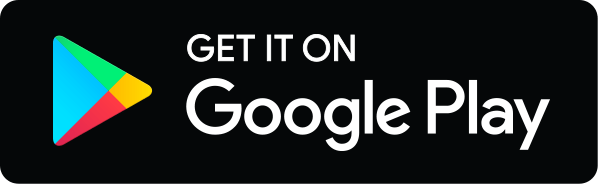