- •
Traditionally, coagulation has been presented as a set of discrete “intrinsic,” “extrinsic,” and “common” pathways, but this view fails to include the many interactions between pathways, any of the natural inhibitors of clot formation, involvement of inflammatory mediators, platelets, endothelial cells, adhesive glycoproteins, or thrombolytic factors in the process of hemostasis.
- •
Generalized bleeding in critically ill children and infants is often caused by trauma or sepsis-related disseminated intravascular coagulation (DIC). The combination of a prolonged prothrombin time, hypofibrinogenemia, thrombocytopenia, and evidence of microangiopathic hemolysis on peripheral blood smear in the appropriate clinical setting is sufficient to suspect the diagnosis of DIC.
- •
Children with severe trauma frequently present with massive bleeding and acidosis secondary to hypoperfusion.
- •
Platelets not only produce a physical barrier at the site of vascular injury, they also serve to focus the clotting process at the point of bleeding by delivering vasoconstrictors and clotting factors to the bleeding site and by providing a surface on which clot development occurs. Additionally, in concert with endothelial cells, they moderate both coagulation and host immune response.
Hemostasis dysfunction is a frequent component of critical illnesses and may comprise an intrinsic component of the illness (e.g., sepsis, trauma) or represent a byproduct or a comorbidity not directly related to the critical illness. While this may frequently lead to a bleeding diathesis, not all hemostatic alterations produce this end result. Indeed, there is a spectrum of potential consequences ranging from none to minor to severe hemorrhage or development of a thrombotic disorder ( Box 89.1 ). A brief review of the initiation and regulation of hemostasis as well as typical/usual diagnostic studies to assess hemostasis provides information to adequately assess the severity and need for intervention in critically ill children (and adults) who present with abnormal hemostasis laboratory results or clinical abnormalities suggestive of altered hemostasis ( Box 89.2 ).
Hemorrhage
Abnormalities in fibrin generation
- •
Consumption of clotting factors
- •
Decreased synthesis of clotting factors
- •
Abnormalities in primary hemostasis
- •
Thrombocytopenia
- •
Endothelial cell dysfunction
- •
Local inhibition of clot formation (e.g., generation of heparan sulfate cleaved from cell membranes)
- •
Increase in fibrinolysis (decreased PAI-1, increased tPA)
- •
Increase in fibrinolysis
- •
Decrease in fibrinolytic inhibitors (e.g., TAFI, PAI-1)
- •
Thrombosis
Endothelial cell dysfunction
- •
Decrease in generation of natural anticoagulant activated protein C with resultant continued activation of clot formation
- •
Decrease in fibrinolysis in conjunction with ongoing thrombin generation
- •
Decrease in ATIII protein C or protein S synthesis
- •
In vivo platelet activation
Decreased cleavage (degradation) of vWF (i.e., decreased ADAMTS13) with resultant increase in platelet adhesion to ECs and collagen matrix
Primary hemostasis → vascular phase
- •
Vasoconstriction in response to vascular injury
- •
Platelet activation and adhesion to endothelial cells and subendothelial matrix (e.g., collagen)
- •
Local platelet activation results in release of peptides that further stimulate vasoconstriction and activation of additional platelets (e.g., serotonin, ADP, thromboxane A2)
Secondary hemostasis → clot formation
- •
Sequential activation of soluble zymogen clotting factors resulting in production of a fibrin clot and platelet scaffold on cell surfaces (e.g., platelets, endothelial cells) and subendothelial matrix (e.g., collagen)
- •
Clot retraction mediated by platelets in the scaffold results in strengthening of local clot
Fibrinolysis → reestablishment of blood flow
- •
Activation of endogenous fibrinolytic enzymes (e.g., conversion of plasmin → plasminogen by thrombin or tPA)
Overview of hemostasis
While the terms hemostasis and coagulation are frequently used interchangeably, they are not synonymous. Coagulation is the process by which blood transforms from a liquid to a solid state and is defined by the process of clot formation. However, hemostasis is more comprehensive and dynamic. It includes all the processes involved in the arrest of bleeding and in maintaining liquid blood when clot formation is not desired. This latter feature requires the process of fibrinolysis to return blood to its flowing state following local clot formation. While coagulation does not occur in flowing blood but rather on cell surfaces or in structures of the extracellular matrix (e.g., collagen), the tests used to measure coagulation use cell-free plasma. However, hemostasis involves platelets and endothelial cells (ECs) along with various plasma proteins that promote and inhibit clot formation. Consequently, the traditional clotting tests most commonly used in clinical medicine (i.e., prothrombin time [PT] and/or International Normalized Ratio [INR], activated partial thromboplastin time [aPTT, also PTT], thrombin time [TT]) assess only one aspect of hemostasis. The process of hemostasis can be thought of as consisting of three separate though highly interdependent and interconnected steps: (1) primary hemostasis (i.e., the adhesion of platelets to ECs with secondary platelet activation and platelet plug formation; Fig. 89.1 ); (2) coagulation (the sequential activation of procoagulant clotting factors; Fig. 89.2 ); and (3) fibrinolysis (the dissolution of formed clots by activation of fibrinolytic proteases from their zymogen to active form; Figs. 89.3 and 89.4 ). In critical illness, the normal regulation (i.e., “balance”) of hemostasis is disrupted, resulting in either hemorrhage (e.g., disseminated intravascular coagulation [DIC]) or pathologic thrombosis (e.g., deep venous thrombosis [DVT], pulmonary embolism; Fig. 89.5 ).





Traditionally, coagulation has been presented as a set of discrete pathways—intrinsic, extrinsic, and common—that progress in an orderly nonoverlapping sequence. This depiction, while useful when explaining the various tests to assess clot formation (i.e., PT/INR, aPTT, TT), fails to represent the many interactions between pathways or include any of the natural inhibitors of clot formation or the involvement of inflammatory mediators, platelets, ECs, adhesive glycoproteins, or thrombolytic factors in the process of hemostasis.
Historically, the intrinsic pathway, beginning with the activation of factor XII (FXII) to activated FXII in contact with some biological or foreign surface, was believed to be physiologically the most important in the initiation of clot formation because deficiencies of FVIII (hemophilia A) or FIX (hemophilia B) produced a severe bleeding diathesis. However, we now understand that the activation of FX to FXa through the action of the FVIIa/tissue factor (TF) complex plays a more central role in this process (see Fig. 89.2 ). The elements of the clotting cascade act in concert, hence the use of the term tenase to describe the action of FVIIa/TF complex, along with the FIXa/FVIIIa complex on the activation of FX to FXa, and the use of the term prothrombinase to describe the FXa/FVa complex, which cleaves prothrombin (FII) to form thrombin (FIIa). Various positive feedback loops involving thrombin enhance clot formation, and several points of crosstalk exist between the two arms of the clotting cascade, among which is FVIIa being able to enhance the activation of FIX to FIXa and FXI to FXIa, further highlighting the central role of FVIIa and TF in vivo (see Fig. 89.2 ). Activation of coagulation initiated by FVIIa/TF results in clotting on biological surfaces (e.g., platelets, ECs, the subendothelial matrix) and biological polymers (catheters, grafts, stents, etc.).
Role of platelets and von willebrand factor in hemostasis
Platelets not only participate in clot formation through the formation of a platelet plug but also provide an important surface on which clot formation may occur, bring additional procoagulant proteins to areas of injury (e.g., FVIII and von Willebrand factor [vWF]), and provide specific proteins that regulate the clotting response (FVIII, inhibitors of fibrinolysis, etc.) required to limit clot formation to the area of tissue injury and bleeding ( Fig. 89.6 ). Under resting unstimulated conditions, platelets do not adhere to the vascular endothelium, but upon stimulation or when the endothelium is mechanically disrupted (e.g., cut) or activated by inflammation, platelets will become activated and express on their surface vWF mobilized from internal pools and bound from plasma-derived vWF. This binding of vWF to the platelet allows for the efficient binding of platelets to activated ECs and to the subendothelial matrix. Once adherent, activated platelets secrete various molecules that further enhance platelet adherence and aggregation, vascular contraction, clot formation, and wound healing. Platelet adhesion to ECs or to subendothelial matrix can be enhanced or diminished by the amount and structure of vWF. Deficiency or inhibition of the metalloproteinase ADAMTS13 will result an increase in circulating unusually large molecular weight multimers of vWF (UL-vWF) with consequent enhanced platelet adhesion, which is associated with an increased risk of unwanted thrombus formation. Conversely, a decrease in the total amount of vWF in circulation or bound to platelets—or in vivo degradation of vWF, as is seen with certain subtypes of von Willebrand disease (vWD)—is associated with decreased platelet adhesion to areas of injury, with consequent increased bleeding. This bleeding diathesis associated with vWD is further increased by a concomitant decrease in circulating FVIII that occurs in most individuals with vWD owing to an increased in vivo degradation of FVIII not complexed to vWF.

Role of endothelial cells in hemostasis
The endothelium plays a critical role in the regulation of blood coagulation and platelet activation. It also modulates vascular tone and permeability, as detailed later (see also Chapter 25 ).
ECs also synthesize and secrete components of the subendothelial extracellular matrix, including adhesive glycoproteins, collagen, fibronectin, and vWF. ECs ordinarily present a nonthrombogenic surface to flowing blood but enhance clot formation when disrupted by trauma or injured by infection or inflammation. This process involves multiple components of the protein C pathway ( eFig. 89.7 ). When this system is disrupted, the endothelium may become a prothrombotic rather than an antithrombotic organ, and localized thrombosis may occur. When thrombin is generated as a consequence of bleeding, thrombomodulin expressed on the surface of ECs bind thrombin, which then facilitates the conversion of protein C bound to the EC protein C receptor (EPCR) to activated protein C (APC), which, in turn, degrades FVIIIa and FVa, thereby damping down further thrombin formation. However, when ECs are stimulated (e.g., by inflammation), surface expressed TF facilitates the conversion of FVII to FVIIa with resultant generation of FXa, which can catalyze the cleavage of prothrombin (FII) to thrombin (FIIa) on cell (phospholipid) surfaces (e.g., platelets). The balance between the generation of thrombin and APC plays a central role in determining whether a clinical syndrome will be characterized by bleeding or by thrombosis.

An additional important property of APC is its modulation of fibrinolysis; APC is capable of neutralizing the fibrinolysis inhibitors plasminogen activator inhibitor type-1 (PAI-1) and thrombin activatable fibrinolysis inhibitor (TAFI). PAI-1 is a glycoprotein of the serine protease inhibitor family. Its primary role in vivo is the inhibition of both tissue- and urokinase-type plasminogen activators. PAI-1 is an acute-phase protein that increases during acute inflammation. In patients with sepsis, increased levels of PAI-1 are associated with increased levels of various cytokines and acute-phase proteins, abnormal coagulation parameters, increased severity of disease, and poorer outcomes. The regulation of the production of PAI-1 is multifactorial. The 4G/5G insertion/deletion promoter polymorphism of the PAI-1 gene has been shown to affect PAI-1 plasma levels; individuals with the 4G/4G genotype display the highest PAI-1 levels, whereas those with the 5G/5G genotype display the lowest (the 4G/5G genotype results in intermediate levels). Differences in PAI-1 levels have been demonstrated to affect the risk of developing severe complications and death from sepsis, higher PAI-1 levels generally being associated with increased mortality in animal models of sepsis and higher severity of illness and organ dysfunction scores in septic patients, including children with meningococcal sepsis. However, the regulation of PAI-1 levels is complex, involving more than promoters of synthesis. APC can stimulate fibrinolysis by forming a tight 1:1 complex with PAI-1, leading to inactivation of this fibrinolysis inhibitor. High levels of thrombin lead to increased levels of APC, which can complex to PAI-1. This complex is subsequently cleared from the circulation, resulting in PC depletion.
Thrombin generation also increases the levels of TAFI, also known as carboxypeptidase R. TAFI is an important negative regulator of the fibrinolytic system and has been shown to inactivate inflammatory peptides that play a role in the contact activation of coagulation, such as complement factors C3a and C5a. The full role of TAFI in the hemostatic and innate immune response to sepsis is still under active investigation. Studies suggest that its role as a regulator of fibrinolysis appears to be of prognostic significance similar to that of PAI-1 (i.e., elevated levels resulting in decreased fibrinolysis appear to be associated with a poorer outcome in sepsis).
Crosstalk between coagulation and inflammation
Evidence exists that the coagulation system developed during evolution as an intrinsic component of the human host defense to infection. Consequently, inflammation, whether induced by infection or noninfectious causes, has the potential to result in activation of coagulation leading to dysregulation of hemostasis. During sepsis, TF expression is upregulated in activated monocytes and ECs as a response to endotoxin and other pathogen-associated/pathogen-initiated events, with both increased secretion of proinflammatory cytokines and activation of coagulation leading to increased thrombin generation. This increase in thrombin generation results in upregulation of coagulation and inflammation through platelet activation and induction of both procoagulant and anticoagulant proteins, and the induction of proinflammatory and antiinflammatory cytokines and mitogenic responses ( eFig. 89.8 ). , Concurrent with coagulation activation, two other crucial mechanisms occur during sepsis. One is the depression of natural anticoagulant systems, involving antithrombin and protein C (PC, APC), and the second is the inhibition of fibrinolysis through the production of PAI-1 and TAFI (see Fig. 89.5 ). Components of the PC system have been shown to be major regulators of coagulation (i.e., natural anticoagulants) with decreased activity of this pathway resulting in pathologic thrombosis, and they have been shown to possess intrinsic immunomodulating properties. In vitro, APC inhibits tumor necrosis factor-α elaboration from monocytes, blocks leukocyte adhesion to selectins, and influences apoptosis. Additionally, coincident with activation of coagulation through the contact phase of activation (FXII conversion to FXIIa mediated by high-molecular-weight kininogen and prekallikrein), complement activation is also induced by the conversion of prekallikrein to kallikrein by FXIIa (see eFig. 89.8 ) The presence of systemic inflammatory syndrome and coagulation activation leading to DIC in the setting of sepsis has been shown to result in worse clinical outcomes whether measured by degree of organ dysfunction or mortality. ECs and the natural inhibitory pathways of coagulation are of particular interest in this intersection of coagulation and inflammation, as potential therapies have been based around these systems. ,

Hypercoagulability and thrombophilia
Hypercoagulable describes the biochemical state wherein one’s coagulation balance favors clot formation, while the term thrombophilia describes the clinical condition of increased risk (incidence) of pathogenic clotting. Both can result from inherited or acquired factors. , As sepsis or critical illness frequently results in an activated clotting balance (hypercoagulable state), the risk of unwanted clotting is high in these patients. Limited studies have also demonstrated that patients exhibiting inherited risk factors for thrombosis are at higher risk for thrombosis during critical illness. The risk of thrombosis is not solely the consequence of activation of hemostasis but is also enhanced by decreased levels of natural anticoagulant proteins (e.g., APC, protein S [PS], and antithrombin-III [ATIII]) and in fibrinolytic activity, which may be acquired or inherited. However, routine screening of critically ill patients for thrombophilic risk factors is not currently recommended. Reduced levels of ATIII and PC may also occur in critically ill children as a result of decreased production secondary to impaired liver function, loss from the vascular space in the case of capillary leakage, and consumption (e.g., the conversion of PC to APC). Additionally, ATIII and PC levels are normally decreased at birth and do not achieve near-adult levels until 3 to 6 months of age. This must be taken into consideration when interpreting levels of these proteins in young infants.
Approach to the patient with an identified or suspected coagulation disorder
Clinical history
Diagnostic assessment begins at the bedside. The medical history, both past and present, may provide insights into the risk for significant bleeding. A prior history of prolonged or excessive bleeding or of recurrent thrombosis is important. Specific questions regarding bleeding should investigate the occurrence of any of the following: spontaneous, easy, or disproportionately severe bruising; intramuscular hematoma formation (either spontaneous or related to trauma); spontaneous or trauma-induced hemarthrosis; spontaneous mucous membrane bleeding; prior problems with bleeding related to surgery (including dental extractions, tonsillectomy, and circumcision); the need for unanticipated transfusions in the past; menstrual history; and, finally, current medications.
In spite of the decreased general use of aspirin in children, there remain numerous over-the-counter aspirin-containing medications that can potentially interfere with platelet-mediated primary hemostasis. Patients (and parents) may not be aware of the aspirin contained in these products. High doses of over-the-counter and prescription nonsteroidal antiinflammatory drugs (NSAIDs)—for example, ibuprofen, and naproxen-sodium—may also impair platelet function. However, unlike aspirin, which produces a noncompetitive inhibition of platelets, the inhibition caused by these other NSAIDs is competitive and resolves once the drug is no longer present. Other drugs used in the intensive care unit (ICU) associated with bleeding abnormalities must also be considered. In trauma situations (either surgical or accidental), it is important to determine the severity of injury relative to the magnitude of bleeding that follows. A prior personal history (or strong family history) of significant thrombosis (e.g., DVT, pulmonary embolus, stroke) also suggests the possibility of the presence of a hypercoagulable condition. As thrombotic events are generally uncommon in children, an occurrence in young adult relatives, particularly early cardiovascular events such as myocardial infarction, should cause the clinician to consider the possibility of a congenital thrombophilic abnormality in the patient. These abnormalities include ATIII deficiency, PC or PS deficiency, the presence of the FV Leiden R506Q mutation, the prothrombin G20210A polymorphism/mutation, and hyperhomocysteinemia (discussed later). In addition, vasculitis associated with an autoimmune disorder such as systemic lupus erythematosus (SLE) must always be considered in the evaluation of a child with an unexplained pathologic clot. In all cases, the family history is important in attempting to separate congenital from acquired disorders.
Generally, defects in primary or secondary hemostasis can be separated according to the nature of the bleeding. Patients with primary hemostatic defects tend to manifest capillary-type bleeding characterized by oozing from cuts or incisions, mucous membrane bleeding, or excessive bruising. This type of bleeding is seen in patients with quantitative or qualitative platelet defects or vWD. In contrast, patients with dysfunction of secondary hemostasis (i.e., fibrin clot formation) tend to display large-vessel bleeding characterized by hemarthrosis, intramuscular hematomas, and the like. This type of bleeding is most often associated with specific coagulation factor deficiencies or inhibitors. However, patients with severe platelet-type defects may also manifest this type of bleeding. Consequently, the presence of only mucosal bleeding is more helpful, as this would point to a platelet-type defect affecting mainly primary hemostasis rather than an abnormality of fibrin clot formation (i.e., a coagulopathy).
Physical examination
Development of generalized bleeding in critically ill patients in the ICU presents a special problem. Such bleeding is often a marker of severe underlying multiorgan system dysfunction. Correction of the coagulopathy usually requires improvement in the patient’s overall clinical status. Supportive evidence or physical findings of other concurrent organ system dysfunction (e.g., renal failure, respiratory failure, hypotension) often are readily apparent. With the exception of massive transfusion syndrome (discussed later), generalized bleeding in critically ill children and infants is often caused by sepsis-related DIC. However, the clinician must also consider in the differential diagnosis the coagulopathy of severe liver dysfunction, undiagnosed congenital bleeding disorders (e.g., hemophilia, vWD), nonaccidental injury, or vitamin K deficiency in newborns or older children with malabsorption. In young infants (<3 months of age), the coagulation system is often not yet mature, and prolongation of the PT or aPTT may not reflect an abnormality in hemostasis. Consequently, the intensivist must also take this into consideration when interpreting abnormal results. In these cases, consultation with a pediatric hematologist may be indicated.
The physical examination of the patient with a bleeding disorder should address several basic questions. Is the process localized or diffuse? Is it related to an anatomic or surgical lesion? Is the bleeding primarily mucocutaneous? Finally, when appropriate, are there signs of thrombosis (either arterial or venous)? The answers to these questions may provide clues to the cause of the problem (primary vs. secondary hemostatic dysfunction).
During the general examination, particular attention should be paid to the presence of several specific physical findings that may be helpful in determining the etiology of a suspected hemostatic abnormality. For example, the presence of an enlarged spleen coupled with thrombocytopenia suggests that splenic sequestration may be a contributor to the observed thrombocytopenia. Splenomegaly accompanied by prolongation of the PT or aPTT may be a consequence of underlying liver disease, whereas splenomegaly in the presence of normal PT and aPTT may indicate that a marrow infiltrative process may be present. Further, evidence of liver disease (e.g., portal hypertension, ascites) points to decreased clotting factor synthesis as a possible etiology of a prolonged PT or aPTT. When lymphadenopathy, splenomegaly, or other findings suggestive of disseminated malignancy are detected, acute or chronic DIC should be suspected as the cause of prolonged coagulation times, hypofibrinogenemia, or thrombocytopenia. Purpura spots that are palpable suggest capillary leak from vasculitis. In contrast, purpura spots associated with thrombocytopenia or qualitative platelet defects are generally not elevated and cannot be distinguished by touch. Finally, venous and arterial telangiectasia may be seen in vWD and liver disease, respectively. When selective pressure is centrally applied to an arterial telangiectasia, the entire lesion fades, whereas a venous telangiectasia requires confluent pressure across the entire lesion (as with a glass slide) for blanching to occur.
Basic tests of hemostasis
A listing of commonly available and used tests of hemostasis is provided in eBox 89.3 . In the general ICU population, the most common tests obtained are PT, aPTT, and fibrinogen. The INR is reported out with the PT, as it is calculated from the PT taking into account the sensitivity of the thromboplastin reagent employed by the testing lab. The INR was developed to allow clinicians to assess and monitor the intensity of anticoagulation employing a vitamin K antagonist (e.g., warfarin) when the PT was obtained in laboratories using different thromboplastin reagents. As the INR is calculated from the PT, it is often used as a surrogate for the INR in which the clinician need not know the control PT from the testing lab. However, the INR has been standardized and validated for the unique derangements in plasma clot formation induced by vitamin K inhibitors; subsequent application to all conditions that may cause a prolongation in PT is conjectural. Indeed, studies investigating the ability of the INR to predict bleeding in numerous clinical settings other than in the presence of warfarin anticoagulation have not demonstrated a clear association, although there may be an association between preoperative INR and postoperative bleeding. That being said, an elevated INR can be assumed to indicate that the PT is also prolonged.
Coagulation (clot formation): functional assays with values either represented as time to fibrin clot formation or calculated from time to clot formation
Primary tests
PT: prothrombin time
- •
Measures clot formation initiated via the “extrinsic” pathway via conversion of X → Xa by the FVIIa/TF complex
- •
aPTT: activated partial thromboplastin time
- •
Measures clot formation initiated via the “intrinsic” pathway via XII → XIIa conversion
- •
Commonly referred to as the PTT (partial thromboplastin time), which is no longer performed
- •
INR: International Normalized Ratio
- •
Calculated from the PT, taking into account the International Sensitivity Index (ISI) of the thromboplastin reagent used by the testing lab
- •
Developed to standardize intensity of anticoagulation for patients on vitamin K antagonist therapy but frequently used clinically to assess state of coagulation and risk of bleeding without supporting data
- •
Fibrinogen: measures the amount of clottable fibrinogen
Secondary tests
TT: thrombin time
Mixing study (inhibitor screen)
- •
Screening test for a clotting factor deficiency versus presence of an inhibitory substance
- •
Performed using a 1:1 mixture of patient plasma/normal plasma; can be performed on either PT or aPTT
- •
Interpretation: correction of a prolonged time into the normal range suggests the presence of a clotting factor deficiency while failure to correct into the normal range suggests the presence of a circulating inhibitor (or inhibitory substance)
- •
Specific clotting factors assays
- •
Obtained if mixing study is “negative” (i.e., demonstrates correction of PT and/or aPTT)
ACT: activated clotting time
- •
Performed using proprietary equipment; results dependent on the machine used
- •
Correlation with other measures of clot formation not established
- •
ROTEM: rotational thromboelastometry; also TEM (thromboelastometry)
- •
Measures clot formation, participation in platelets in clot formation, clot strength, and fibrinolysis
- •
Differs from TEG in that the sensor pin is oscillated within the blood sample
- •
TEG: Thromboelastogram
- •
Measures clot formation, participation in platelets in clot formation, clot strength, and fibrinolysis
- •
Differs from ROTEM (TEM) in that the blood sample is rotated around the fixed sensor pin
- •
Fibrinolysis (clot breakdown)
FDPs/FSPs: fibrin degradation products/fibrin split products
- •
Measures degradation fragments of native fibrinogen and fibrin
- •
Elevated in disseminated intravascular coagulation (DIC) and in syndromes characterized by primary fibrinogenolysis
- •
D-Dimer
- •
Measures fragment produced by proteolysis of polymerized fibrin monomer
- •
Requires cleavage of Aα and Bβ peptides from fibrinogen by thrombin
- •
Found to be (more) specific for DIC than FDPs/FSPs
- •
Plasminogen
- •
Zymogen form of plasmin; consumed during active fibrinolysis
- •
Platelet count
- •
Level below which platelets must be transfused has not been determined
- •
In oncology patients on chemotherapy, a threshold of 10,000/μL platelets is generally used for prophylactic platelet transfusion
- •
Does not provide information regarding function of platelets
- •
Platelet aggregation studies
- •
Provides information on platelet function
- •
Time-consuming and generally not available on an emergency basis; requires a large amount of blood
Bleeding time
- •
Manual test can be performed at bedside; reproducibility questionable
- •
No longer offered by many labs/institutions
- •
Bleeding time linearly prolonged at platelet counts <100,000/μL
Platelet mapping
- •
Obtained from TEG/ROTEM results; relies on evaluation of clot strength to enable a quantitative analysis of platelet function
- •
Variable results; specificity and utility in clinical decision-making not established
- •
PFA-100
- •
Developed as an in vitro bleeding time; poor correlation with platelet function by aggregometry and no longer used as a test of platelet function
- •
The standard tests to assess the status of the coagulation system use the conversion of fibrinogen to fibrin as their end point in one way or another. Consequently, they can be considered to be functional assays. While prolongation of the PT/INR or aPTT are interpreted as indicating a hypocoagulable state (i.e., decreased rate of clot formation), neither test is sensitive to conditions that result in an increase in the rate of clot formation (i.e., a hypercoagulable state). Correct interpretation of the PT and aPTT in neonates, particularly premature infants, requires that the clinician recognize two factors that cause normal values for the PT and aPTT to be longer than those for older children. First is that, at birth, plasma levels of many of the clotting proteins are low. While some studies demonstrate a slow increase into early adolescence, these levels generally reach near normal adult levels by 3 months of age, at which time the results may be accurately interpreted using the normal age range. The second is that the normal hematocrit for neonates is greater than that for older children, resulting in a reduced plasma volume. When the hematocrit is 55% or greater, this reduced plasma volume may result in an “over-anticoagulation” of a specimen collected into a typical commercial tube. Consequently, if the result is high, a repeat specimen obtained in a tube with reduced citrate should be obtained for confirmation of the abnormal results. This adjustment may also be appropriate when obtaining specimens from older children with elevated hematocrit secondary to cyanotic heart disease.
The thrombin time (TT) tests the conversion of fibrinogen to fibrin. Prolongation of the TT may reflect low fibrinogen levels (hypofibrinogenemia); presence of an inhibitor to thrombin (commonly, heparin contamination of the specimen); or the presence of an abnormal, poorly clottable, fibrinogen (dysfibrinogenemia). Fibrinogen levels <75 to 100 mg/dL are generally considered to represent a risk of bleeding. Elevated fibrinogen (hyperfibrinogenemia) is frequently seen in critically ill patients, as fibrinogen is an acute phase reactant. Consequently, finding a low-normal fibrinogen should cause the clinician to consider a process in which fibrinogen is being consumed (i.e., a consumptive coagulopathy). In this setting, one may consider obtaining a measure of fibrinogen/fibrin degradation (i.e., marker for the presence of fibrinolysis). While there are assays for both fibrin(ogen) split products, the D-dimer assay is generally considered to be more specific for the detection of fibrin degradation products that form following the generation of polymerized fibrin ( eFig. 89.9 ). While traditional fibrin split products (FSPs)/fibrin degradation products (FDPs) identify all proteolytic fragments of both native fibrin monomer and polymerized fibrin, the D-D fragment (D-dimer) is only produced following cleavage of fibrinogen by thrombin to form fibrin monomer, which then has (spontaneously) polymerized to form fibrin polymer. The finding of an elevated D-dimer requires that thrombin generation had occurred. However, trauma and surgery will result in increased D-dimer levels resulting from bleeding and response to bleeding.

The mixing study, also referred to as an inhibitor screen , is a useful next step when evaluating a prolonged PT or aPTT. If the prolongation is the result of a critical deficiency of a specific clotting factor(s), mixing of the patient specimen 1:1 with normal plasma will result in correction of the PT or aPTT into the normal range. Failure to demonstrate a meaningful correction suggests the presence of an inhibitory substance (e.g., an antibody) that impairs fibrin formation.
Currently, the assessment of an increased risk of pathologic clot formation relies on identification of myriad clinical and laboratory risk factors; numerous factors with the strongest association with thromboembolic events (TEs) are listed in eTable 89.1 . The role that other factors—such as ABO type, platelet cytoadhesion receptors, fibrinolysis regulatory proteins, and blood lipids—may play in TEs is currently uncertain.
Inherited | Acquired |
---|---|
Protein C deficiency | Critical illness, trauma |
Protein S deficiency | Central venous catheters |
Antithrombin deficiency | Oral contraceptives (estrogen) |
Elevated factor VIII | Pregnancy |
Dysfibrinogenemia | Obesity |
Factor V Leiden mutation | Age (infancy, adolescence) |
Prothrombin gene mutation (G20210A) | Antiphospholipid antibodies (especially, anti-PS, anti-β 2 -glycoprotein-1 antibodies) |
Elevated homocysteine |
The presence of antibodies directed against phospholipids may result in an artifactual prolongation of the PT and/or aPTT. These are referred to as lupus anticoagulants (LACs), as they were first described in patients with SLE. Prolongation of PT or aPTT caused by LAC does not reflect an increased risk of bleeding but rather can indicate a risk for thrombosis. While LAs are not inherently a risk for TEs, the specific antiphospholipid antibodies (APAs) are. Specifically, antibodies directed against phosphatidylserine and β 2 -glycoprotein-1 have been shown to represent strong risk factors for TEs. ,
Platelet function testing
While platelets participate in clot formation and deficiencies in platelet number or function increase the risk of bleeding, platelet function is not measured in the traditional plasma-based clotting studies. Platelet function testing has traditionally been performed in a plasma-free system in which aggregation of platelets is measured following addition of a specific platelet agonist ( eFig. 89.10 ). The pattern of responsivity gives clues to the specific functional defect, which is diagnostic at times. , This test is time intensive, requires a generous blood sample, and does not reflect the interaction of platelets with other cells and its role on clot strength and stability. Consequently, in an acute setting, these tests are of minimal usefulness. The template bleeding time assesses the role of platelets in initiating platelet clot formation in vivo (i.e., primary hemostasis). However, it is a manual test and poorly standardized. Consequently, many hospital laboratories no longer offer the test. A commercial test developed as an in vitro bleeding time (PFA-100) was touted as a substitution for the template bleeding time. However, it has not demonstrated the specificity required to be clinically useful and has fallen out of favor by many hematologists. There are flow cytometry–based tests measuring platelet activation, but these have not yet been incorporated into general laboratory platelet testing. Platelet function assessment by platelet mapping obtained from global measures of hemostasis have been developed; however, these also lack specificity and sensitivity and are not generally available.

vWF is a multimeric adhesive glycoprotein found in plasma, the extracellular matrix, ECs, and platelets. It is required for efficient adhesion of platelets to ECs and extracellular matrix and circulates in complex with FVIII. In this state, it protects FVIII from proteolytic cleavage. vWD is the most common inherited bleeding disorder, affecting 1% of the population. Acquired vWD has been documented in various lymphoproliferative, myeloproliferative, and autoimmune disorders. It has also been documented in a variety of congenital and acquired cardiac conditions characterized by an increase in blood flow shear rates. Such shear-induced acquired vWD has also been demonstrated with intravascular ventricular assist devices in adults and with extracorporeal membrane oxygenation (ECMO). The etiology of shear-induced vWD is related to promotion of self-association of vWF and increased activity of the vWF cleaving protease ADAMTS13 with consequent decrease of high-molecular-weight vWF multimers in circulation causing decreased platelet adhesion. This phenomenon may be more pertinent to children with critical illness. The diagnosis of vWD relies on three types of measurements: quantitative antigenic measurement of plasma vWF, a functional platelet adhesion/aggregation assay (ristocetin cofactor assay), and determination of plasma vWF multimeric profile. FVIII activity is also obtained, as the absence of vWF results in low FVIII activity due to in vivo proteolytic degradation. The type of inherited vWD will determine the pattern of results noted. In conditions of acquired vWD due to increased shear stress, one would expect to see both decreased total vWF and a loss of the largest vWF multimers in circulation.
Thromboelastography and thromboelastometry
Tests that measure thromboelastic characteristics of clot formation and dissolution in real time have been used to obtain more precise information regarding abnormalities in hemostasis and to guide hemostatic support measures. These tests include thromboelastography (TEG) and rotational thromboelastometry (ROTEM). The primary difference in test technique is that TEG employs a system in which a probe placed into a cuvette containing whole blood is rotated around the pin while clot formation occurs while ROTEM involves rotating the pin in the blood contained within the cuvette. Additionally, the probe (pin) is a torsion wire in TEG and an optical probe in ROTEM. Both provide information regarding rate of clot formation, maximal strength of the clot, and the timing of fibrinolysis ( eTable 89.2 and eFig. 89.11 ). To date, studies evaluating the superiority of these tests compared with traditional plasma-based clotting studies are inconclusive.
Variable | TEG | ROTEM |
---|---|---|
Measurement period | – | Reaction time |
Time from start to when waveform reaches 2 mm above baseline | R | Clotting time |
Time from 2 mm above baseline to 20 mm above baseline | K | Clot formation time |
Alpha angle (degree) | < (slope between R and K) | < (angle of tangent at 2 mm amplitude) |
Maximum strength | Maximal amplitude | Maximal clot firmness |
Time to maximum strength | – | MCF-t |
Amplitude at a specific time | A30, A60 | A5, A10… |
Clot elasticity | G | MCE |
Maximum lysis | – | CLF |
Clot lysis (CL) at a specific time (minutes) | CL30, CL60 | LY30, LY45, LY60 |
Time to lysis | 2 mm from maximal amplitude | CLT; 10% difference from maximum clot firmness |

Abnormal hemostasis in critical illness
Hemorrhage is common in critically ill patients. While bleeding secondary to a consumptive process (e.g., DIC) is serious and often encountered, there are many other causes for bleeding in ICU patients. Before appropriate therapy can be instituted, the intensivist must identify the general cause of the bleeding. eTable 89.3 presents the usual laboratory results noted in the most common of these conditions.
Clinical Syndrome | Screening Tests | Supportive Tests |
---|---|---|
Disseminated intravascular coagulation (DIC) | Prolonged PT, aPTT, TT; decreased fibrinogen, platelets; microangiopathy | (+) FDPs, D-dimer; decreased factors V, VIII, and II (late) |
Massive transfusion | Prolonged PT, aPTT; decreased fibrinogen, platelets ± prolonged TT | All factors decreased; (–) FDPs, D-dimer (unless DIC develops); (+) transfusion history |
Anticoagulant Overdose | ||
Heparin | Prolonged aPTT, TT; ± prolonged PT | Toluidine blue/protamine corrects TT; reptilase time normal |
Warfarin (same as vitamin K deficiency) | Prolonged PT; ± prolonged aPTT (severe); normal TT, fibrinogen, platelets | Vitamin K–dependent factors decreased; factors V, VIII normal |
Liver Disease | ||
Early | Prolonged PT | Decreased FVII |
Late | Prolonged PT, aPTT; decreased fibrinogen (terminal liver failure); normal platelet count (if splenomegaly absent) | Decreased factors II, V, VII, IX, and X; decreased plasminogen; ± FDPs unless DIC develops |
Primary fibrinolysis | Prolonged PT, aPTT, TT; decreased fibrinogen ± platelets decreased | (+) FDPs, (–) D-dimer; short euglobulin clot lysis time |
Thrombotic thrombocytopenic purpura | Thrombocytopenia, microangiopathy with mild anemia; PT, aPTT, fibrinogen generally within normal limits/mildly abnormal | ADAMTS13 deficiency/inhibitor, unusually large von Willebrand factor multimers between episodes; mild increase in FDPs or D-dimer |
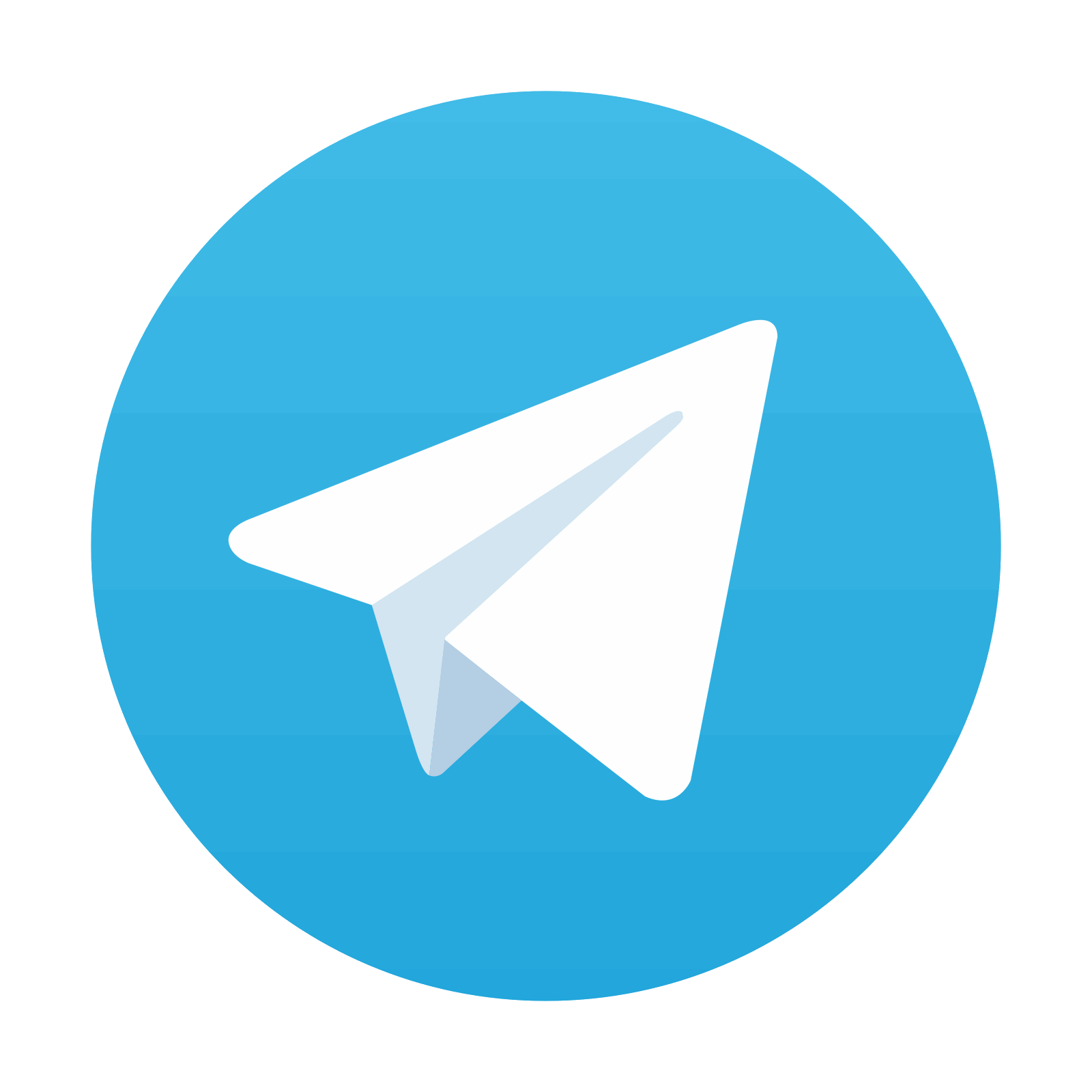
Stay updated, free articles. Join our Telegram channel

Full access? Get Clinical Tree
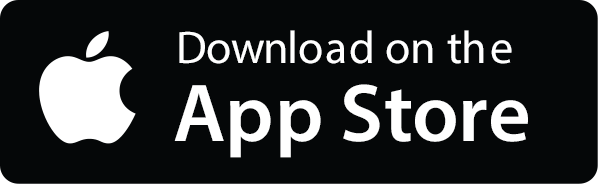
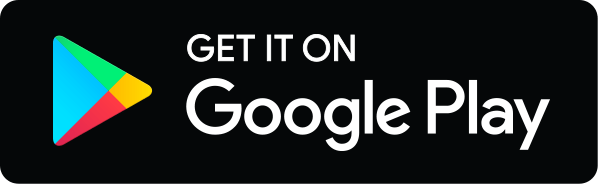
