Fig. 7.1
Physiopathology of trauma-induced coagulopathy
Transfusion of ≥10 units of RBCs, which approximates the total blood volume (TBV) of an average adult patient, within 24 h
Transfusion of at least 4 units of RBCs in 1 h with anticipation of continued need for blood product support
Replacement of .50 % of the TBV by blood products within 3 h
Patients who undergo massive transfusion have a high mortality rate. A mortality rate ranging between 25 % [11] and 50 % [12–15] has been reported. A recent meta-analysis focused on the association between RBCs transfusion and mortality or other significant outcomes such as multiorgan failure (MOF) and acute respiratory distress syndrome (ARDS) [16]. According to this study, the odds for mortality increases with each additional unit of RBCs transfused as well as the risks of MOF and ARDS. However, these results are based on observational studies only.
7.2 Clinical Management
In recent years, international guidelines [17–19] have been developed aimed at preventing and treating trauma-induced coagulopathy (TIC). The immediate identification of patients who may require transfusion of several units of blood is critical to ensure a timely intervention. Unfortunately clinical criteria as well as predictive scores have a lower than desirable predictive ability for massive transfusion. Predictive models of massive transfusion upon admission have been developed for this purpose. The Trauma-Associated Severe Hemorrhage (TASH) score has been reported to have a high predictive value [20] and is widely used in Germany and other European countries. The TASH score (Table 7.1) is based on a point system taking into consideration clinical and laboratory data as well as the severity of injuries (orthopedic fractures and intra-abdominal fluid). In a multicenter study based on the data of the German Trauma Registry, Borgman [21] divided the population of trauma victims according to their TASH score. A TASH score ≥ 15 was associated with a 40 % risk to receive a massive transfusion. Patients with a TASH score ≥ 15 had a high ISS (42 ± 15.4) and a mortality rate of 39.5 %. Maegele [22] and Brockamp [23] used the German Trauma Society database to evaluate the predictive values for MT of six different scoring algorithms. Although the weighted and more complex systems such as the TASH score had the highest overall accuracy, more simple score as the one proposed by Vandromme [24] performed nearly as well. The Vandromme score is based on five parameters four of which immediately available by clinical examination or by the generally available point-of-care devices: blood lactate ≥5 mMol/L, heart rate >105 bpm, INR >1.5, hemoglobin ≤11 g/dL, and systolic blood pressure <110 mmHg. Its predictive value for the need of massive transfusion increases with the number of positive parameters, and the model with ≥3 positive criteria showed a sensitivity of 53 % and a specificity of 98 %. A major limitation of all models is their retrospective nature and the lack of prospective validation. It has been therefore suggested that individual triggers should be considered in order to improve the accuracy of the models [46]. An unpublished analysis of about 1700 major trauma cases from the Italian Trauma Registry (RIT) showed that hypotension (systolic blood pressure, SBP ≤90 mmHg) on hospital admission is associated with a mortality rate as high as 50 % mainly due to uncontrolled hemorrhage. However many hypotensive patients do not meet the MT criteria as they do not survive enough time to receive 10 units of RBCs.
Table 7.1
The TASH score
TASH Score (Trauma-Associated Severe Hemorrhage) Predicts the need for massive transfusion based on clinical and laboratory data | |
---|---|
Sex | |
Male | +1 |
Female | 0 |
Hemoglobin | |
<7 g/dL | +8 |
<9 g/dL | +6 |
<10 g/dL | +4 |
<11 g/dL | +3 |
<12 g/dL | +2 |
≥12 g/dL | 0 |
Base excess | |
< −10 mmol/L | +4 |
< −6 mmol/L | +3 |
< −2 mmol/L | +1 |
≥ −2 mmol/L | 0 |
Systolic blood pressure | |
<100 mmHg | +4 |
<120 mmHg | +1 |
≥120 mmHg | 0 |
Heart rate | |
>120 bpm | +2 |
≤120 bpm | 0 |
Positive FAST for intra-abdominal fluid | |
YES | +3 |
Clinically unstable pelvic fracture | |
YES | +6 |
Open or dislocated femur fracture | |
YES | +3 |
Based on the available data from the literature and the trauma registries, the Italian Trauma Network (TUN) suggests to start an aggressive coagulation support in all the patients presenting with a significant uncontrolled bleeding associated with one or more of the following criteria (Fig. 7.2): SBP <100 mmHg, base excess (BE) < −6, lactate >5 mMol/L, hemoglobin <9 g/dL, or a international normalized ratio (INR) >1.5. The last criterion is thought to be relevant in the case of patients referred from other hospitals with laboratory data showing an impaired coagulation.
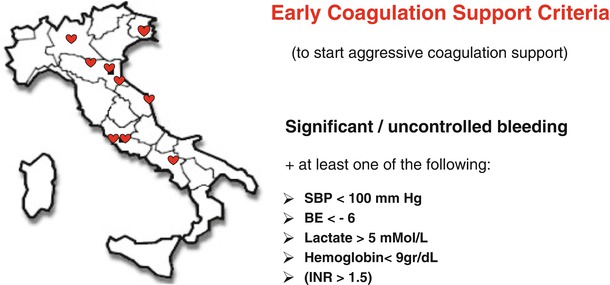
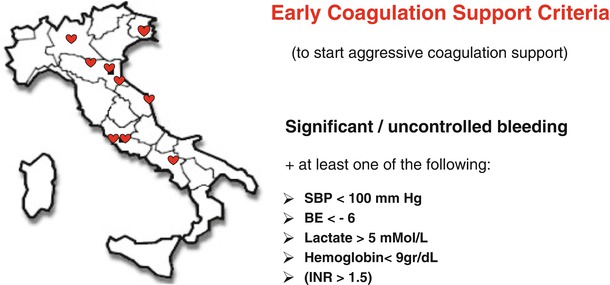
Fig. 7.2
Criteria to start coagulation support by “blind” administration of 2 g of Fibrinogen concentrate according to the ECS protocol
Any therapeutic strategy should rapidly tackle acute traumatic coagulopathy through the early replacement of clotting factors. However, due to the heterogeneous availability of hemocomponents and clotting factors in different countries and to the lack of sound data in the literature, there is not a widely agreed clinical strategy yet.
Moreover, because of the aging population in western countries, elderly people with cardiovascular comorbidities and on antiplatelet agents or oral anticoagulants are increasingly represented, thus introducing further complexity in identifying an adequate treatment approach for most of the bleeding patients.
An ideal strategy to prevent and treat the coagulopathy trauma should focus on four key points to be addressed in quick succession:
1.
Prevention and treatment of hyperfibrinolysis
2.
Identification and reverse of drug-induced coagulopathy
3.
Early support to the hemostatic process
4.
Goal-directed correction of specific coagulation abnormalities
7.2.1 Prevention and Treatment of Primary Hyperfibrinolysis
Hyperfibrinolysis has been identified in a significant percentage of severely injured patients. Acutely injured patients with severe hyperfibrinolysis (maximum clot lysis >15 % after 30 min) are reported to have mortality rates exceeding 70 % [25, 26]. The CRASH-2 [27] trial demonstrated the potential benefit of empiric antifibrinolytic therapy in injured patients. This study led to a Level 1A recommendation for early administration of antifibrinolytic agents. Based on the results of the CRASH-2 study, administration of tranexamic acid (TA) to all patients with ongoing bleeding or at risk of significant bleeding is now standard care. Although, hyperfibrinolysis may be identified only in a limited number of severely injured patients by viscoelastic tests Raza [28] demonstrated that as many as 57 % of the severely injured patients had fibrinolytic activation measured by plasmin-antiplasmin complex. These data may better explain the results of the CRASH-2 study. Although according to the current recommendations, all the bleeding patients should receive TA soon after injury, TA is probably to be avoided at a later stage. A new analysis of the CRASH-2 study shows that if treatment is not given until 3 h after injury, it is less effective and could even be harmful [29].
Recently, Moore and associates [30] observed three distinct phenotypes of fibrinolysis in response to trauma. Despite having similar demographics and injury patterns, 64 % of their patients presented with a fibrinolysis shutdown, while physiologic fibrinolysis was observed in 18 % of the patients and hyperfibrinolysis in another 18 %. Hyperfibrinolytic patients had a higher mortality rate due to exsanguination occurring early after injury, whereas shutdown patients had an increased delayed mortality more frequently due to organ failure. Modest levels of fibrinolysis seem to be protective. Therefore, according to this data, there might be a U-shaped curve in mortality with the highest peak for patients with acute hyperfibrinolysis and a second peak for patients with a fibrinolysis shutdown. This finding comes from a retrospective study and needs to be considered with caution. However, if they were confirmed, this could lead to reconsider the present indications for a mandatory initial administration of TA.
7.2.2 Identification and Reversal of Drug-Induced Coagulopathy
The number of patients on antiplatelet or anticoagulation therapies is increasing. A retrospective cohort study of trauma centers submitting data to the National Trauma Databank (NTDB) from 2002 to 2007 demonstrated an increase in vitamin K antagonists (VKA) use in the general trauma population from 2.3 % in 2002 to 4 % (p < 0.001) in 2006. In patients older than 65 years, the use of VKA increased from 7.3 to 12.8 % in the same period (p < 0.001). Pre-injury VKA use is age related with a sharp increase between the age of 45 and 70 years. Head trauma patients on VKA have an increased risk of intracranial hemorrhage. The younger patients on anticoagulants have a 50 % higher mortality [31]. Recent guidelines recommend emergency anticoagulation reversal with prothrombin complex concentrate (PCC) and vitamin K in trauma patients with major bleeding or cerebral hemorrhage who are on VKA treatment [32–34]. PCCs are classified into three-factor and four-factor products. Three-factor concentrates have therapeutically useful level of FII, FIX, and FX; four-factor PCCs contain FII, FVII, FIX, and FX together with protein C and S. PCC is associated with a non-negligible risk of thrombogenicity and disseminated intravascular coagulation (DIC) [35]. Eight retrospective studies have evaluated the outcomes associated with pre-injury anticoagulation therapy in patients admitted for traumatic head injury. In two of these studies, the mortality was similar between the control and warfarin-treated patients; in the other six studies, there was an increased mortality in the warfarin-treated group compared to the control group [36]. While mortality has been shown to increase in patients with traumatic brain injury on VKAs, data for trauma patients without brain injury are less clear. “Time to correction of the INR” or “extent of correction of the INR” has been used as a surrogate outcome of clinical effectiveness rather than the cessation of clinical bleeding or mortality. Only one retrospective analysis could demonstrate that in trauma patients receiving warfarin, the addition of PCC treatment to fresh frozen plasma (FFP) and vitamin K results in a quicker INR reversal. However, no outcome improvement was observed [37]. Notwithstanding the lack of strong evidence, all international guidelines agree in recommending the use of PCC for the emergency reversal of vitamin K-dependent oral anticoagulation. According to the ECS protocol, patients with significant active bleeding known to be on VKAs should immediately receive 25 UI/kg of PCC even before INR results. PCC treatment will be subsequently adjusted according to INR results [38, 39]. The italian F.C.S.A. recommendations [40] for the management of intracranial hemorrhage have been endorsed to guide treatment once INR is known (Fig. 7.3).
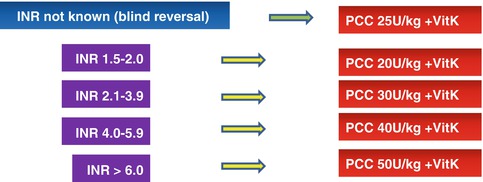
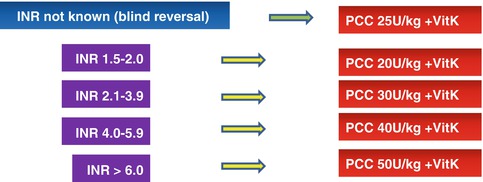
Fig. 7.3
Anticoagulation reversal for bleeding patients on vitamin K antagonists (From FCSA recommendations [40], modified)
Recently, several new oral anticoagulants (NOACs) have been introduced in the clinical practice, and a few more are under clinical development. These drugs are thought to have a more balanced benefit/risk ratio and several advantages over warfarin: a rapid onset of action, a more predictable anticoagulant effect so that routine laboratory monitoring is not required, and minor food and drugs interactions. Moreover they may carry less hemorrhagic risks, particularly, less intracranial bleeding [41]. NOACs are still relatively known to the practicing community, and there is little or no evidence to guide practical management when patients present with acute bleeding. The use of NOACs as well as the strategies to reverse their effects in case of bleeding is addressed in a separate section of the book (Chap. 12). We will therefore focus on a few simple concepts important to manage trauma patients with critical bleeding. Major and/or life-threatening bleeding should be treated with immediate anticoagulant withdrawal. However, neither FFP nor cryoprecipitate is effective in reversing the anticoagulant effect of the new drugs [42]. A few studies have investigated reversal agents; all of these, except one, have used animal model to evaluate the role of different reversal drugs. The only human study focused on the effect of four-factor PCC on coagulation tests [43]. The use of activated charcoal has been suggested if oral drug assumption is within a couple of hours. High doses of 3-factor or 4-factor PCC are probably effective for the reversal of factor Xa inhibitor such as rivaroxaban, but not for thrombin inhibitor as dabigatran [44]. The Italian Federation of Thrombosis Centers produced a consensus document suggesting the use of hemodialysis as a therapeutic option in case of assumption of dabigatran and the administration of 4-factor PCC for the reversal of rivaroxaban in the one-shot dose of 50 IU/kg [45]. The European Guidelines suggest to start with a similar dose (25–50 IU/kg).
Antiplatelet drugs – mostly aspirin and P2Y12 antagonists (clopidogrel, prasugrel, ticlopidine, and ticagrelor)are prescribed for prophylaxis and treatment in patients with cardiovascular or cerebrovascular diseases. These drugs are associated with an increased risk of intracranial hemorrhage. Drugs of both classes (with the exception of ticagrelor) irreversibly inhibit platelets function making their short half-lives clinically irrelevant. For each day after the interruption of these medications, the 10–14 % of normal platelet function is restored; so it takes 7–10 days for the entire platelet pool to be refurbished.
There is little evidence to guide platelet transfusion in major trauma patients on antiplatelet medications. A recent meta-analysis of six studies failed to demonstrate any clear benefit of platelet transfusions in patients with either spontaneous or traumatic intracranial hemorrhage [46]. In a retrospective analysis of a cohort of 113 patients with traumatic intracerebral hemorrhage (TICH) on antiplatelet medications, Washington and associates [47] could not find any statistically significant difference in outcome between patients treated with platelets and those who were not transfused. Similar data were reported by Downey in a retrospective study involving two level 1 trauma centers over a 4-year period [48]. Ivascu [49] and Fortuna [50] in two separate studies observed a nonsignificant trend toward higher mortality in patients with traumatic brain injury treated with platelets transfusion, but patients transfused were older and presented a lower Glasgow Coma Scale (GCS) and higher Injury Severity Score (ISS) [35]. All of these studies have some weakness: they are retrospective and relative small, and the indication and timing for platelet transfusion were not standardized. The timing of transfusion might play a key role as the hematoma may set up very early after trauma [51].
Despite the lack of evidence supporting the use of platelets, many institutions have implemented protocols for the reversal of platelet medications in the presence of TICH. According to a multidisciplinary institutional protocol published by Campbell et al. [52], 5 units of platelet concentrate (PLT) should be transfused right on admission to the patients on aspirin who present with a small TICH. Patients on P2Y12 antagonists or with large TICH should receive 10 units of platelets together with 0.3 mcg/kg of desmopressin. As patients with major trauma and critical bleeding often require surgical or intravascular procedures, many authors recommend the use of platelet concentrates even in the absence of brain injury [53].
7.2.3 Early Support to the Hemostatic Process
Hemostasis is critically dependent on fibrinogen as a substrate for clot formation. Fibrinogen is the single factor which is more and earlier affected in case of TIC. Many bleeding trauma patients with TIC present with a depletion of fibrinogen below levels currently recommended for therapeutic supplementation. Recently, Schlimp et al. [54] demonstrated that fibrinogen levels upon admission show strong correlation with rapidly obtainable routine laboratory parameters such as hemoglobin and BE. A level of fibrinogen lower than 150 mg/dL is detected in as many as 73 % of the patients with admission hemoglobin lower than 10 g/dL and in 63 % of those with a BE lower than −6. Moreover Rourke and Brohi [55] found low fibrinogen in 41 % of the patients who were hypotensive on admission. In their study, hypotension, increasing shock severity (as measured by the base deficit), and high degree of injury (ISS ≥25) were all associated with a reduction in fibrinogen levels. Fibrinogen depletion is associated with poor outcomes, and survival improves with the amounts of fibrinogen administered [56]. Fibrinogen is by far the most extensively represented coagulation protein in plasma. One liter of FFP contains on average 2 g of fibrinogen. However, until a few years ago, FFP transfusion was not recommended for the bleeding trauma patients in absence of a prolongation of the prothrombin time (PT) or INR or fibrinogen decrease to less than 1.5 g/L [57]. In the year 2007 retrospective evidences from both military [58] and civilian [59] practice were published suggesting improved outcomes in patients with massive bleeding, after the adoption of a massive transfusion protocol (MTP), including the early administration of high-dose FFP therapy. According to these first reports, a 1:1 FFP/RBCs ratio was associated with a sharp decrease in mortality. Because of these findings, significant changes in the treatment strategy of patients with critical bleeding were introduced, bringing to anticipate coagulation support by administering FFP with a high FFP/RBCs ratio. Several studies focused on this strategy trying to determine if standard doses of FFP and platelets in a fix ratio RBCs were able to improve survival. Notwithstanding a large number of studies, there are still conflicting evidences regarding the use of high ratios. Although many authors suggested that early and aggressive FFP transfusion may reduce mortality [60], the optimal FFP/RBC and PLT/RBCs ratio is controversial because of the possible survival bias flawing most studies [61, 62]. Survival bias is the bias resulting from the fact that surviving patients are more likely to receive more FFP and platelets compared with non-survivors, because they lived long enough to receive those blood products. This issue is still under debate: a multicenter prospective study on a large population of patients undergoing MT showed that high FFP/RBCs and PLT/RBCs ratios are associated with a survival benefit also when time dependency is accounted for [63], but more recently other authors came to opposite conclusion [64]. In their study, Khan and Brohi [65] observed that there was no consistent correction of any measure of clot function nor any large increases in the procoagulant factor level, when FFP was delivered during the acute phase of ongoing bleeding. Furthermore, FFP/RBCs ratios above 1:2 were not associated with any major hemostatic benefit.
Despite the widespread use of blood components to treat TIC, their effects on the coagulation profile when administered during damage control resuscitation remain therefore largely unknown. To define which is the best FFP/RBC and PTL/RBC ratio, a large multicenter randomized study has been designed: the Pragmatic Optimal Platelet and Plasma Ratio (PROPPR) trial [65]. The PROPPR was designed as a randomized trial to be conducted in subjects with the highest level of trauma activation and predicted to have a massive transfusion. Twelve North American level 1 trauma centers were involved in the trial. The PROPPR aimed to look for a different outcome in patients randomized into one of two standard transfusion ratio interventions: 1:1:1 or 1:1:2 (FFP, PLT, and RBCs). Despite being able to randomize over 680 patients, the PROPPR trial was unable to reach definitive conclusions [66]. Patients treated with a 1:1:1 ratio, who therefore received PLT in a very early treatment stage, had a 5 % lower 24-h mortality and a 3.7 % lower hospital mortality if compared with those who initially received FFP (but no PLT) in a 1:2 ratio and were subsequently treated with a 1:1:2 PLT/FFP/RBCs ratio. However, these differences were not statistically significant. The early use of PLT was not associated with a higher rate of ARDS.
Transfusing patients based on an empirical ratio rather than guided solely by laboratory data (goal-directed) is considered controversial by some researchers [67]. Moreover there are still conflicting opinions among the experts on the choice of using FFP as the initial strategy for supporting coagulation. Severe tissue damage and hemorrhagic shock are frequently associated with a decreased fibrinogen. Administration of FFP to bleeding patients may stabilize fibrinogen levels, avoiding a further decrease, but FFP transfusions cannot result in a significant increase of fibrinogen levels unless very high volumes are infused [68]. The ACIT study [69] confirmed these findings showing that fibrinogen did not correct with standard damage control resuscitation.
There are indeed several limitations on the use of FFP to prevent and treat TIC. Both FFP and pathogen-inactivated plasma (industrial purified plasma) need to be group matched, thawed, and warmed before administration. Therefore, unless pre-thawed plasma is available, plasma transfusion cannot be started at the same time as RBCs. A delay of 93 min has been reported [61] possibly explaining why the targeted FFP/RBCs ratio is reached only a few hours after starting treatment. During this interval, fibrinogen level is likely to be lower than desired.
FFP transfusion has also been associated with an increased risk of post injury multiple organ dysfunction syndrome (MODS), ARDS, and infections. These complications increase with the volume of transfused FFP [70]. The risk of transfusion-related acute lung injury (TRALI) has been greatly reduced by avoiding the use of the FFP of women with pregnancy history [71]. In Italy the recent introduction of pathogen-inactivated plasma enabled to further minimize the risks of TRALI and virtually eliminate the risks of transmission of infective diseases. Although these measures are effective in reducing some of the adverse effects of FFP transfusions, there are data [72] showing that in patients who require less than 6 units of RBCs, the complications related to plasma transfusion may overcome the benefits.
Moreover the early and aggressive administration of plasma may result in diluting the blood cells with a decrease in hemoglobin level [73] and may further contribute to reduce platelet marginalization with a potentially negative impact on platelet activation. Therefore, dilutional anemia might bring to transfusing a higher amount of RBCs in order to adhere to the transfusion trigger recommended by the European Guidelines (7–9 g/dL) [17].
The balance between a rapid intervention to treat or prevent TIC and the risk related to unnecessary transfusion of plasma is difficult to achieve. Therefore, several authors prefer to start treatment with coagulation factors and in particular with fibrinogen.
The use of coagulation factor concentrates has been proposed as the strategy to reduce and even avoid plasma transfusion in patients with significant bleeding. In the Austrian guidelines [74], fibrinogen concentrate is the first-line treatment. Two retrospective studies comparing data from Austrian Trauma Centers with the German Trauma (TR-DGU) registry reported a significant reduction in platelet transfusion and a limited decrease in blood transfusion for bleeding patients treated with fibrinogen concentrate and PCC who did not receive FFP during initial resuscitation [75, 76]. However, these results have not been confirmed by prospective controlled studies.
Although very appealing, the “plasma-free” strategy proposed by Schöchl [75] may not be easily adopted by many trauma centers as it requires thromboelastometry monitoring to be started straight on patient’s admission and not all the hospitals can adhere to this requirement at the moment. Moreover it may imply the use of a rather high amount of fibrinogen with considerable related costs.
7.2.4 Goal-Directed Correction of Specific Coagulation Abnormalities
The utility of the standard coagulation tests (PT, INR, activated partial thromboplastin time [aPTT], platelet count, and fibrinogen levels [Clauss method]) to guide the acute hemostatic treatment in trauma patients with significant bleeding is limited. First, their results are inevitably delayed. In addition, because they are not performed on whole blood but on plasma, they cannot analyze the entire process of coagulation as it occurs in vivo. Moreover, hyperfibrinolysis cannot be detected [77]. Although these conventional coagulation assays do not predict the future need for massive transfusion and have limited utility to direct ongoing blood component therapy in real time because of slow turnaround times, nevertheless all the guidelines still suggest they should be ordered to provide information that might be helpful in correcting abnormalities occurring during resuscitation. Recently, it has been suggested that point-of-care hemostasis assays, such as thromboelastography (TEG) and rotational thromboelastometry (ROTEM), might be better at assessing coagulopathy in patients with critical bleeding and TIC [78, 79]. These assays offer to clinicians a graphic representation of the coagulation process. In addition, the parameters obtained from TEG/ROTEM could provide a quantitative measure of individual components of the hemostatic process. Hence, the use of TEG/ROTEM can provide better information to guide blood component therapies in a more timely manner. There are several advantages of using TEG/ROTEM: first of all, the turnaround time for these assays is shorter compared with conventional assays; thus, they can be used in combination with clinical assessment for the decision-making process. Moreover the viscoelastic point-of-care (POC) tests can detect severe hyperfibrinolysis that plays a major role in TIC. TEG/ROTEM can be performed at the patient’s true temperature, which makes it more sensitive for detection of coagulopathy due to hypothermia. In a recent in vitro experimental study, Winsted et al. [80] observed that hypothermia affects coagulation more during hemodilution with starches than with crystalloids. Fibrinogen concentrate is effective in correcting dilutional coagulopathy induced by crystalloids irrespective of temperature. However, it is much less effective in case of hypothermia-induced coagulopathy associated with colloid-induced hemodilution. TEG/ROTEM has been shown to reduce the transfusion requirement and need for MT in patients undergoing cardiovascular surgery [81]. The early use of thromboelastometry to guide treatment is recommended by the Austrian Guidelines [19]. However, notwithstanding all these potential advantages, a Cochrane review suggested that there is no evidence that TEG/ROTEM reduced the morbidity and mortality in the massively transfused patients [82].
7.3 The Early Coagulation Support Protocol (ECS)
The updated European Guidelines [17] for management of bleeding and coagulopathy recommend that every trauma center implement an evidence-based treatment algorithm for the bleeding trauma patient and promote the use of treatment algorithms to guide clinical management. To adhere to these recommendations, the Italian Trauma Centers Network (TUN) has developed a treatment algorithm (early coagulation support or ECS) [83] aiming to improve and homogenize the early treatment of trauma patients with significant bleeding and at high risk of massive transfusion. The ECS has been formally adopted by several Italian Trauma Centers; to our knowledge, this is the first example in Europe of the adoption of the same step-by-step algorithm for the management of trauma-induced coagulopathy by a large number of trauma centers. The ECS protocol is summarized in Fig. 7.4. The ECS protocol is an integrated part of a comprehensive damage resuscitation strategy. It is also based on fluid restriction and the prohibition of colloids. As well as having the objective of ensuring an earlier coagulation support, the protocol also aims to limit the use of plasma in patients who are not likely to need it, in order to reduce plasma-related adverse effects.