Fig. 12.1
Heparin rebound. Postoperative bleeding in aortic valve replacement. (a) Kaolin-TEG trace without heparinase. (b) Kaolin-TEG trace with heparinase. The presence of heparinase reduces R-time and K-time and normalizes alpha angle and MA
In the presence of heparin, TEG parameters return within reference ranges if the tests are performed in heparinase-coated cuvettes, suggesting that the enzyme is able to successfully neutralize the anticoagulant effect of UFH [40]. Heparinase-modified TEG is useful to monitor heparin reversal in special clinical settings such as cardiac surgery [43].
Heparinase I is an enzyme derived from the bacterium Flavobacterium heparinum that neutralizes heparin by enzymatic cleavage of alpha-glycosidic linkages at the antithrombin-binding site [44, 45]. If heparin activity is identified as the cause of bleeding, reversal with protamine can be performed avoiding unnecessary and potentially harmful blood transfusions.
In addition, heparinase is able to antagonize up to 6 IU/mL of heparin, so during cardiopulmonary bypass, the heparinase-modified thromboelastogram and the HEPTEM test of ROTEM are feasible despite anticoagulation with heparin in doses of 300 IU/kg, giving the chance to early understand the potential hemostatic deficiency regardless of heparin before CBP weaning [45, 46].
Therapeutic UFH affects TEG parameters at lower concentrations than those that affect the PT, aPTT, TT, and ACT and in a dose-dependent fashion. For example, the aPTT is only slightly prolonged at doses of heparin that will completely inhibit TEG clotting. The standard TEG is more sensitive to UFH and low-molecular-weight heparin (LMWH) than most conventional coagulation tests, except the anti-FXa activity. The difference between standard and heparinase-modified TEG parameters increases the sensitivity of the assay for the effects of heparins and is more sensitive to very low doses of UFH than anti-FXa activity [40].
The influence of unfractionated heparin on thromboelastometry parameters is markedly stronger than that of the LMWHs [47].
12.2.5 Low-Molecular-Weight Heparin Antithrombotic Effect Monitoring
LMWHs are derived from UFH by chemical or enzymatic depolymerization and have higher antifactor Xa and reduced antifactor IIa activity (in an antifactor Xa/antifactor IIa ratios between 2:1 and 4:1 depending on the type of LMWH). LMWHs present superior pharmacokinetic properties with a more predictable dose-response with respect to UFH due to their reduced binding to plasma proteins, endothelial cells, and macrophages [2]. As a rule, laboratory monitoring is needed when the variation in the individual response to a given drug is so variable that a correlation between dose and standard effect is difficult to predict [48]. The good bioavailability and long half-life of subcutaneous LMWHs provide a predictable anticoagulation effect and consequently routine monitoring of their action is not necessary. However, in special populations prone to overdosing such as pediatric, obese, and pregnant patients and in case of renal insufficiency (creatinine clearance <30 mL/min) LMWH anticoagulant effect is not foreseeable and laboratory measurements are recommended [2], LMWHs do not prolong the assays used to monitor UFH (aPTT and ACT) [49]. The antifactor Xa activity monitored by chromogenic assay is the test currently recommended by the College of American Pathologist [50]. However, this assay is neither standardized nor routinely available universally and around the clock. To overcome this drawback, a point-of-care qualitative test called enoxaparin clotting time has been developed, but currently it is withdrawn from the market [9].
In case of bleeding complications during LMWH therapy a quick bedside qualitative/semiquantitative monitoring able to discriminate the effect of the LMWHs as a major contributor to the hemostatic disturbance could be useful [51]. In this clinical setting, viscoelastic assays have been tested. Coppell and associates have investigated the correlation between the variables of the standard and heparinase-modified TEG with conventional clotting tests (aPTT, TT and antifactor Xa activity) obtained from in vitro citrated samples spiked with increasing amounts of UFH, LMWH, and danaparoid from 0.025 to 1.0 U/mL. The results of this study pointed out that UFH and LMWH (dalteparin) are able to modify the following TEG parameters: the R-time, the K-time, the α-angle and the MA. In Coppell’s study, LMWH prolongs R-time at blood concentrations ≥0.5 IU/mL and K-time at concentrations ≥0.0025 IU/mL, and reduces α-angle at concentrations ≥0.25 IU/mL and the MA at any concentration. Furthermore, the addition of heparinase neutralizes LMWH and normalizes TEG parameters [40] (see Fig. 12.2).
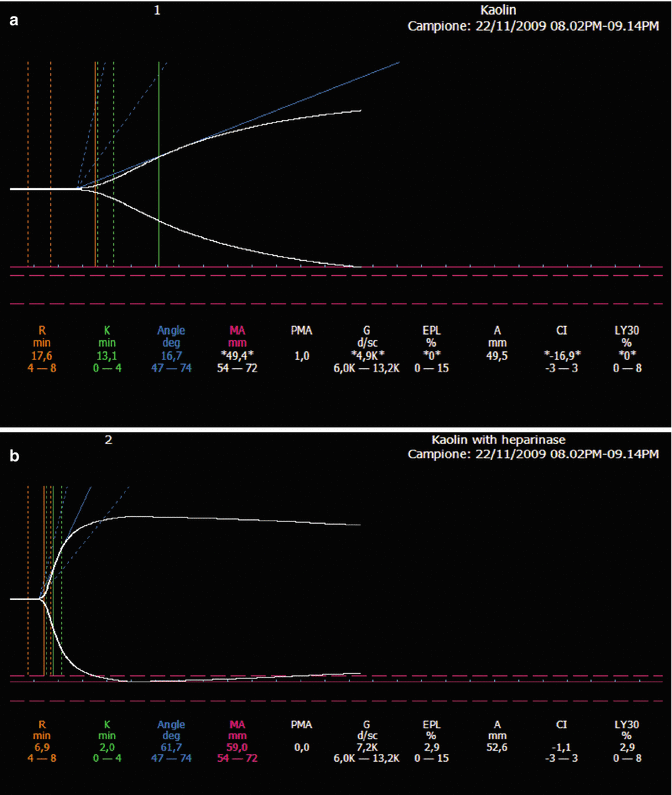
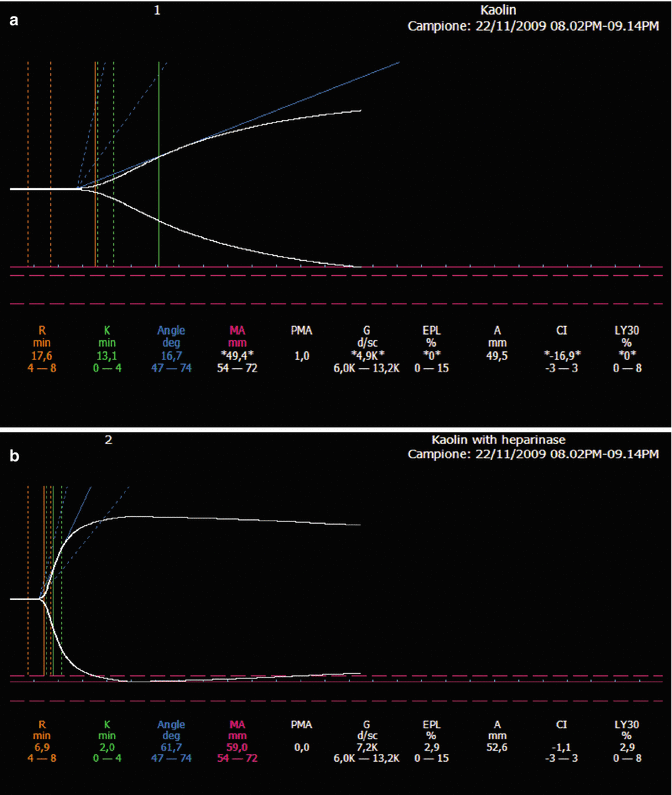
Fig. 12.2
Effects of therapeutic doses of enoxaparin (80 IU/kg twice a day) in a patient with thrombosis of right jugular subclavian axis. (a) Plain kaolin-TEG trace. (b) Kaolin-TEG trace with heparinase. In the presence of low-molecular-weight heparin, heparinase normalized the prolonged R- and K-times and the reduced alpha angle and maximum amplitude
In case of bleeding due to multiple and dynamic factors, VETs are able not only to establish the contribution of anticoagulants to the coagulation disorder but also to monitor the normalization of hemostasis after a reversal of the drug has been administered [1]. Regarding different LMWH agents, Zmuda and associates showed that enoxaparin caused a dose-dependent inhibition of TEG clotting of normal blood. Abnormal TEG clotting was observed in patients receiving enoxaparin whose plasma level of the drug was more than 0.1 anti-Xa U/mL. However, the degree of TEG abnormality did not always coincide with plasma levels of the agent. The inhibition of TEG clotting by enoxaparin was reversed by protamine sulfate and heparinase [39]. TEG is able to detect the presence of enoxaparin but does not seem an appropriate assay for monitoring adequate enoxaparin antithrombotic therapy. This statement is based on the inconsistent findings for the TEG parameters (reaction time, K-time and alpha angle) for plasma levels of anti-Xa activity [39]. Similar results have been pointed out by other authors. White and associates showed that there was no evidence of association between R and anti-Xa in patients treated with 1 mg/kg of enoxaparin. In addition, TEG was unable to predict anti-Xa activity. However, TEG R was prolonged in more than 90 % patients and showed a correlation with the dose of enoxaparin [52]. These conclusions are not share by other authors. Klien and associates underlined that the R-time from thromboelastogram correlates with serum anti-Xa in an orthopedic population treated with enoxaparin [53]. Therefore, the correlation between R-time and antifactor Xa in patients treated with LMWH remains still controversial. In order to optimized the thromboelastometric capability to monitor the anticoagulant effects of LMWH, new reagents like prothrombinase-induced clotting time reagent are currently under investigation [54].
Regarding the possibility of preventing bleeding associated to LMWH administration in surgical populations, the use of thromboelastography has been also proposed preoperatively to establish an adequate window of anticoagulant effect leading to a decreased risk of perioperative bleeding complications [55].
12.3 Fondaparinux
Fondaparinux is a synthetic factor Xa inhibitor analog of the active pentasaccharide present in UFH and LMWHs that involves the antithrombin-binding site [56–58]. It is approved for thromboprophylaxis in medical and orthopedic patients, for treatment of venous thromboembolism, and for NSTEMI-acute coronary syndromes in Europe after the results of the OASIS-5 trial [59, 60].
Due to its 100 % bioavailability after subcutaneous administration, fondaparinux elimination is completely dependent on renal clearance; therefore, it is contraindicated in patients with severe renal insufficiency (creatinine clearance <30 mL/min). In patients with moderate renal insufficiency (creatinine clearance 30–50 mL/min), the dose should be reduced by 50 %.
Routine anticoagulant monitoring is not recommended, but, considering that fondaparinux activity is not reversed by a specific antidote, in special situations such as renal insufficiency, extremes of body weight, and bleeding complications, it is useful to determine its real anticoagulant effect [48, 58, 61]. Fondaparinux slightly (but without clinical significance) prolongs the PT and the aPTT [62] and has no effect on the ACT. Therefore, these assays are not adequate to monitor the anticoagulant effect of fondaparinux [63]. A standard curve taking fondaparinux as a calibrator should be used for reporting fondaparinux levels using an antifactor Xa assay [62]. Patients with Non-ST segment elevation-Acute Coronary Syndrome (NSTE-ACS) often require urgent percutaneous coronary interventions (PCI) or aortocoronary bypass surgery, so a point-of-care test able to monitor quickly the anticoagulant effect of this agent should be useful. The main limitation of antifactor Xa assay calibrated for fondaparinux is the same that happens for LMWHs. It is not available in every laboratory at every time. In order to try to overcome this drawback, clinicians have analyzed the possibility of monitoring the fondaparinux anticoagulant activity using the point-of-care tests of hemostasis usually present in the intensive care units and operating theaters.
Gerotziafas and associates analyzed in vitro the influence of clinically relevant concentrations (prophylactic and therapeutic) of fondaparinux on thromboelastographic profiles of citrated whole blood of 12 healthy volunteers after triggering TF pathway with a minimal amount of thromboplastin. Diluted thromboplastin was added to samples supplemented with fondaparinux (0.25; 0.5; 1 μg/mL). At concentrations used in prophylaxis regimen (0.25 μg/mL equivalent to 0.27 anti-Xa IU/mL), fondaparinux significantly prolonged the R- and K-times but did not significantly modify the alpha angle as compared to the control. At concentrations observed after administration of therapeutic doses for the treatment of deep venous thrombosis (≥0.5 μg/mL for fondaparinux), fondaparinux induced a significant prolongation of R- and K-times and a significant decrease of the alpha angle (p < 0.05). Fondaparinux did not induce modifications of MA at any concentration. In this study, R- and K-times were the most sensitive parameters of the modified TF-TEG assay to reveal the anticoagulant effect of prophylactic concentrations of fondaparinux. A ceiling effect of the inhibitory effect of fondaparinux was observed at the concentration of 0.55 anti-Xa IU/mL. This study provides evidence that the whole-blood TF-triggered TEG assay is sensitive to the presence of clinically relevant concentrations of fondaparinux. Moreover, the alpha angle may be used in order to distinguish the effect of prophylactic and therapeutic concentrations [64].
However, these results have been not confirmed by other authors. Eller and associates spiked blood samples from healthy volunteers with therapeutic and supra-therapeutic concentrations of fondaparinux and other direct antifactor Xa and antifactor IIa drugs. Only supra-therapeutic concentrations of fondaparinux significantly influenced the clotting time (CT) of INTEM assay of ROTEM. No significant modifications were observed at therapeutic and prophylactic doses. Moreover, no modifications of EXTEM-CT were observed at any concentration.
Again, in another study, the authors prospectively measured the antifactor Xa levels and analyzed the thromboelastographic profile triggered by kaolin of whole-blood samples of orthopedic patients treated with prophylactic doses of fondaparinux (2.5 mg once a day). Samples were taken at baseline (before first postoperative injection of fondaparinux), 2 h after administration, 17 h after administration (half-life of fondaparinux), and 24 h after administration of the drug. The R-time was into the normal range at any phase of the study, and there were not significant differences between the R mean value at the different phases. Moreover, the reaction time did not correlate with antifactor Xa levels at any phase. Only the parameter MA had a significant variation over time [66].
In conclusion, further studies are necessary to establish the real capability of viscoelastic point-of-care assays to monitor the anticoagulant effect of pentasaccharides.
12.4 Parenteral Direct Thrombin Inhibitors
As well known, heparin is far to be the ideal anticoagulant. Its main drawbacks are that heparin can inhibit only fluid-phase thrombin as opposed to other thrombin inhibitors (e.g., bivalirudin) that can inhibit clot-bound thrombin. Heparin is not a direct thrombin inhibitor and requires antithrombin (AT) as a cofactor, thus heparin efficacy is susceptible to pharmacodynamic differences based on AT level and activity (heparin resistance). Finally, heparin binds to endothelial surfaces and has high affinity to platelets (platelet factor 4), potentially leading to heparin-induced thrombocytopenia [4, 5].
In order to overcome these limitations, parenteral direct thrombin inhibitors (DTI) have been developed. The current marketed parenteral DTIs are bivalirudin, argatroban, and danaparoid. The FDA-approved indications of bivalirudin are anticoagulation during percutaneous transluminal coronary angioplasty (PTCA), percutaneous coronary intervention (PCI) with use of glycoprotein IIb/IIIa inhibitors, and for patients with or at risk of heparin-induced thrombocytopenia (HIT) and HIT with thrombosis (HITT) undergoing PCI. In Canada, bivalirudin is also approved for patients with or at risk of HIT undergoing cardiac surgery [68]. Moreover, bivalirudin has been proposed as an off-label alternative to UFH during on-pump and off-pump cardiac surgery in patients affected by HIT [69–72].
Bivalirudin is a potent reversible bivalent direct inhibitor of free and clot-bounded thrombin. It inhibits the activation of fibrinogen and the thrombin-mediated activation of factors V, VIII, and XIII and platelets. One of its main advantages is its low immunogenic potential. Bivalirudin has a unique pharmacologic profile: it undergoes predominant non-organ elimination by plasmatic proteolysis with only a 20 % of renal elimination that imposes, in any case, a dose reduction in case of renal insufficiency. Bivalirudin is the parenteral DTI with the shortest half-life (25 min) [73]. Bleeding is the major adverse effect, more commonly found in patients with renal impairment.
Regarding laboratory monitoring, PT, ACT, aPTT, and TT are all prolonged in a linear fashion with increased doses of bivalirudin, but this linearity is lost at high doses [74].
Bivalirudin is usually monitored by aPTT in case of anticoagulation for HIT/HITT [68] maintaining a therapeutic range from 1.5 to 2.5 times the upper limit of normal range.
INR rises in the presence of bivalirudin, so attention must be paid during DTI shift to vitamin K antagonist (VKA) when concomitant administration of both drugs occurs, as thrombosis risk exits with premature discontinuation of DTI due to wrong INR interpretation [75].
ACT is generally used to monitor bivalirudin during interventional cardiology and in cardiac surgery (with and without CPB) [69–72]. However, the ecarin thrombin time would be the preferred assay, if available [75].
Monitoring bivalirudin with a point-of-care ecarin clotting time (ECT) during cardiac surgery has been anedoctically proposed too [76].
Argatroban is the second parenteral DTI currently on the market. It is a synthetic DTI that reversibly binds to activated thrombin. It exerts its anticoagulant effects by inhibiting thrombin-induced reactions including fibrin formation and activation of factors V, VIII, and XIII and platelet aggregation. Its half-life is longer than that of bivalirudin (45 min). It has a hepatic clearance, so no dose modification is necessary in case of renal impairment [77]. It has been approved for prophylaxis and treatment of thrombosis complicating HIT and in patients with or at risk of HIT undergoing PCI.
As bivalirudin, argatroban dose dependently increases aPTT/ACT/PT/INR and TT. The aPTT is the recommended assay for monitoring the anticoagulant effect of argatroban in patients with HIT. The suggested aPTT target is 1.5–3 times the baseline value and it should never exceed 100 s. aPTT loses its linear relationship with argatroban plasma concentrations if aPTT is higher than 60 s. In this case, shift to ECT is necessary. ACT is the suggested test in patients undergoing PCI with a therapeutic target of 300–450 s [77].
Regarding the capacity of viscoelastic point-of-care tests to monitor parenteral DTI, an in vitro study has demonstrated that argatroban at supra-therapeutic and therapeutic concentrations prolongs the INTEM-CT and the EXTEM-CT [65]. In a clinical study involving patients undergoing PCI the R-time of a modified thromboelastograph, ecarin clotting time had a better correlation (r 2 = 0.746) with bivalirudin level than the standard ACT (r 2 = 0.306) [78]. Again, ecarin modified rotational thromboelastometry (EMT) has been proposed as a point-of-care monitor of argatroban. Ecarin acts as a direct thrombin activator. It is a metalloprotease isolated from the venom of the saw-scaled viper that activates prothrombin without affecting other coagulation factors and independently of the calcium, phospholipids, factor V, and heparin. In this study, the correlation between argatroban concentration and EMT-CT was high (r 2 = 0.94) and statistically significant [79].
Definitely, the development of a reliable point-of-care monitoring of DTIs still remains a challenging issue because a strict control of the level of anticoagulation is necessary as specific antidotes are not available. Unlike that for argatroban, in case of bleeding due to overdose of bivalirudin, hemodialysis, hemofiltration, and plasmapheresis can be helpful because they are able to remove significant amount of the drug [68].
12.5 Vitamin K Antagonists
Arterial and venous thromboses are common causes of morbidity and mortality for the surgical and medical patients and increase with age. The thrombosis risk stays for about a year after the triggering event [80], and among the drugs used for the long-term anticoagulation in the risk patients, the vitamin K antagonists (VKA), which are used for those up to 60 years, symbolize the cornerstone of the treatment. They are approved for the prevention and/or treatment of venous thrombosis, pulmonary embolism, and thromboembolic complication [81]. These drugs are prothrombin synthesis inhibitors, and they can be divided in two categories: the coumarins and the indandiones [82].
Those belonging to the first class work with the same mechanism of action: they interfere with the carboxylation of glutamate residues on the N-terminal regions of vitamin k-dependent proteins as II, VII, IX, and X. The principal action develops through the cyclic interconversion of vitamin K, so the vitamin K-dependent coagulation factors are produced by the liver partially carboxylated and decarboxylated with reduced coagulation activity. These drugs inhibit also the synthesis of endogenous anticoagulant proteins such as protein C and S [82].
However, the coumarins have a different pharmacokinetic and pharmacodynamic mechanism.
The molecules differ primarily for half-lives 9 h for acenocoumarol, 30–40 h for warfarin, and up 120 h for phenprocoumon [81]. Their use is influenced by pharmacological interactions, particularly the drugs which work through the hepatic P450 cytochrome. Moreover vegetables and also some other food can alter the pharmacokinetics and the pharmacodynamics of these drugs.
Warfarin is the most common used in clinical situations, it is given orally and it is rapidly adsorbed from the gastrointestinal tract. Its mechanism is influenced by metabolic clearance and vitamin K intake.
Acenocoumarol is derivate from coumarin, and the age and its dosage is established by two parameter, body mass index and international normalized ratio (INR). The therapeutic effects are faster than warfarin that gives more steady hematic concentration.
The use of VKA is increasing, but bleeding is a frequent and important complication affecting between 10 and 16 % of the treated patients. The reasons are due to incorrect treatment in 20–40 % of them and to spontaneous bleeding which has a frequency of 14 % in the emergency department’s admissions due to drug-related adverse effects, while during hospitalization is 25 % [83].
Monitoring of VKA therapy is the tool for the effectiveness of these drugs and INR is the only parameter used until now: the typical target of INR range for the anticoagulation is 2–3 [84]. INR (international normalized ratio = patient PT/mean normal PT) is the standardization of the PT based on ISI values derived from the plasma of patients who had received stable anticoagulant doses for at least 6 weeks [82]. It explores the extrinsic or vitamin K-dependent factors of the coagulation cascade. In some settings, such as surgery and trauma, INR is not reliable to predict the coagulopathy and has a relatively long turnaround time [85].
A still open question is if the POCT could result useful to manage or to understand the variations on hemostatic balance induced by VKA.
The POCT can rapidly assess global hemostasis and give information to treat patients at bedside [18], to manage bleeding, and to measure the anticoagulation level in order to prevent thrombotic events.
Among POCT, the coagulometers perform a valuable aid to measure INR. Several devices are available: the CoaguChek XS, INRatio Time, ProTime, and SmartCheck. All start the clotting process by a recombinant thromboplastin, except SmartCheck which uses thromboplastin from rabbit brain. These coagulometers differ in the methodology of clotting detection: respectively, electrochemical detection of thrombin activity, electrochemical detection of changes in impedance of blood, and cessation of blood flow through capillary channel and a magnetic field. These tests are assessed by their accuracy and precision, and the imprecision is expressed by means of coefficient of variation, which should be less than 3 % [86]. Furthermore, the coagulometers are not subject to control and standardization as laboratory measurements: only CoaguChek presents a calibration process of each lot with the corresponding code chip.
The coagulometers are tools to self-monitoring the INR: the frequent control confirms the clinical efficacy and safety of VKA treatment also if the choice of coagulation monitor had no effect on clinical outcome of a large patients’ cohort [87].
Regarding VET POCT, there is not an agreement about indications to the use of TEG or ROTEM for monitoring the VKA effect.
The TEG assesses the global process of hemostasis, analyzing the viscoelastic properties of blood during the clotting process. The role of the activator is very important. The reagents can quicken the coagulation and the kaolin spur the intrinsic via. So clotting times measured by aPTT correspond to coagulation time (R-time) in TEG.
The patients treated with VKA and showing a therapeutic INR presented a TEG signature typically normal, and the literature described several case reports where there is no agreement between the variation of INR and the TEG parameter [88].
The kaolin-TEG is insensitive to warfarin effects and also, when triggered by both kaolin and tissue factor, is not able to show the alteration of hemostasis induced by coumarins, maybe due to that the intrinsic pathway activation may mitigate detection of an extrinsic pathway coagulopathy [88]. Again, Nascimento and associates conclude that in trauma patients, the reaction time of RapidTEG® is not superior to INR to identify vitamin K factors deficiency [85]. A recent analysis [89] confirmed this result, even if the markers of thrombus generation [reaction time (R), maximum rate of thrombus generation (MRTG), and time to maximum range thrombus generation (TMRTG)] correlated with INR in a series of 104 patients.
With regard to the ROTEM, performed in patients treated with VKA and triggered by calcium chlorides and tissue factor, a marked significant increase in clotting time (CT) [4] and higher levels of maximum clot firmness (MCF) and area under curve (AUC) even if their values were in range were obtained. Of special interest is the MCF in FIBTEM, which was significantly higher in VKA patients than in the control group: this feature probably describes a hypercoagulable paradox of the patients anticoagulated, being MCF in FIBTEM a parameter influenced by fibrinogen concentration and fibrin polymerization [90].
In conclusion, among POCTs the coagulometers are safe and adequate for clinical use, even if they need quality control and calibration. Although several papers were published during the last years about the use of the TEG and ROTEM to identify and manage the coagulopathy and antithrombotic therapy, at present these techniques are puzzling to understand the hemostatic alteration induced by VKA and data from literature are few and lacking. Despite all its criticisms, to date the INR remains the gold standard laboratory test for warfarin monitoring.
12.6 Novel Oral Anticoagulants
Agents directly inhibiting thrombin (dabigatran) and activated factor X (rivaroxaban and apixaban) are now increasingly employed in preventing and treating venous thromboembolism in orthopedic surgery and preventing arterial thromboembolism in non-valvular atrial fibrillation [91]. Thrombin and factor Xa have a central role in the coagulation cascade, being the converging point of the extrinsic and the intrinsic pathways. Activated factor X is the enzyme that triggers the prothrombinase to thrombin activation. Thrombin catalyzes the conversion of fibrinogen to fibrin, activates positive feedback signals for coagulation amplification, and is a strong platelet activator, being the final and the most important contributor to clot formation and stability. The development of these novel drugs was aimed at increasing the efficacy and overcome variable dosing and safety issues of the traditional anticoagulants. Indeed, these inhibitors show a faster onset of action, reduced half-lives, a wider therapeutic window, fewer drug interactions, and more predictable pharmacokinetics allowing to get over the routine coagulation monitoring required in patients administered with traditional drugs, such as warfarin and heparin. Noteworthy, the anticoagulant effect of these inhibitors cannot be reversed specifically and antidotes are still to undergo definitive clinical testing and approval [92, 93]. Interindividual variability of patient response to anticoagulant administration and thus a particular need for drug activity monitoring, especially in patients with changing renal function and in the elderly, still remains a not fully clarified matter [94]. Till then, rapidly recognizing and monitoring the extent of the coagulation suppression in settings where bleeding could put the patient in danger of life is of fundamental importance.
Emergency situations, such as urgent invasive surgery, hemorrhage, or overdose, may require a rapid and reliable assessment of the extent of the anticoagulant effect. Monitoring is also required in assessing the patient compliance to the treatment or tracking the anticoagulation reversal in critical settings [95]. To date there are no commercially available antidotes towards these novel anticoagulant agents. Until the promising specific antibodies will be available, prothrombin complex concentrate (PCC), activated prothrombin complex concentrate (aPCC), factor VIII inhibitor bypassing activity (FEIBA), and recombinant activated factor VII (rFVIIa) have been proposed for managing bleeding complications in patients under active treatment [95, 96].
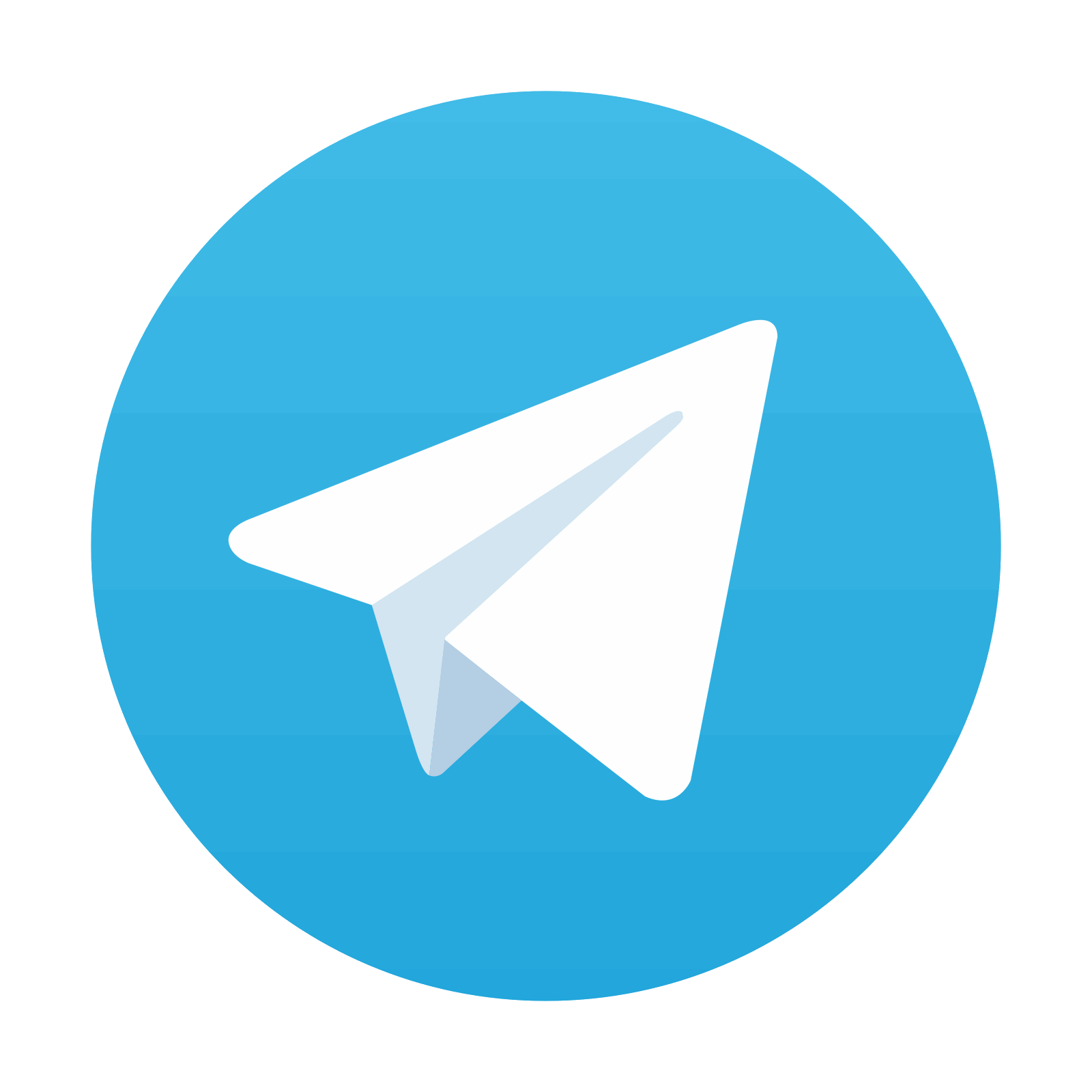
Stay updated, free articles. Join our Telegram channel

Full access? Get Clinical Tree
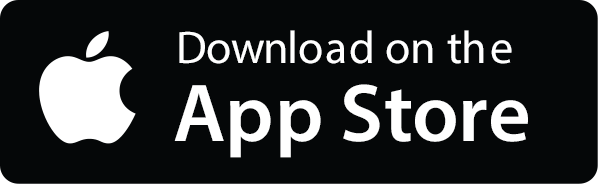
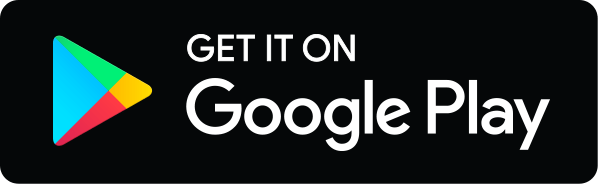