Chronic Pain Management
Honorio T. Benzon
Robert W. Hurley
Timothy Deer
Asokumar Buvanendran
Key Points











Related Matter
Nociceptive Pathways
Pain Sensitization

Primary Afferents and Peripheral Stimulation
A variety of mechanical, thermal, electrical, or chemical stimuli can result in the sensation and perception of pain. Information about these painful or noxious stimuli is transmitted to higher brain centers by receptors and neurons that are often distinct from those that carry innocuous somatic sensory information. The mammalian somatosensory system is subserved by four groups of afferent fibers differentiated by their anatomy, rate of transmission, and sensory modality transduced (Table 57-1).
The first group, the heavily myelinated large-diameter A alpha (Aα) fibers, have specialized terminals incorporated within muscle spindles, Golgi tendon organs, and joints. These fibers and their respective end organs transduce proprioceptive information. The second group, the heavily myelinated large-diameter A beta (Aβ) fibers, have specialized encapsulated nerve endings including the Meissner, Pacinian, and Ruffini corpuscles and the Merkel disc, which transduce innocuous or low-threshold mechanical stimulation. Aα fibers do not ordinarily participate in signaling pain sensations to the central nervous system. However, the activation of Aβ and, possibly, Aα fibers has been invoked as a part of the mechanism for the production of pain relief by transcutaneous electrical nerve stimulators, which may implicate both fibers in a role in pain signal processing.1 As well, it is becoming increasingly apparent that in chronic pain states, these fibers may indeed participate in pain signaling by adopting a “phenotype” similar to that of a C fiber (vide infra).2

The majority of Aδ and C nociceptors are polymodal and therefore are responsible for the transduction of noxious stimuli of different modalities. Nociceptive nerve endings are also located in muscle, the fascia, and adventitia of blood vessels, the knee joint, the dura, and the viscera. Recent evidence suggests that sensory transduction in the skin can include mediation by nonneural skin cells including keratinocytes and epithelial cells. These cells are thought to directly participate in touch and thermal sensation and are believed to communicate with the nerve ending through paracrine transmission.5 The keratinocytes and epithelial cells may also play a role in neuropathic and inflammatory pain conditions though the release of neuronal activating chemical mediator, or neurotransmitter, including calcitonin gene–related
peptide (CGRP).6 Chemical mediators of pain are numerous. These mediators come from sources intrinsic to the neuron, including various neurotransmitters such as serotonin and substance P, and extrinsic to the nervous system, including substances from inflammatory/immune cells and red blood cells such as prostaglandins, kinins, cytokines, chemokines, and adenosine triphosphate that are released following injury to the tissue.
peptide (CGRP).6 Chemical mediators of pain are numerous. These mediators come from sources intrinsic to the neuron, including various neurotransmitters such as serotonin and substance P, and extrinsic to the nervous system, including substances from inflammatory/immune cells and red blood cells such as prostaglandins, kinins, cytokines, chemokines, and adenosine triphosphate that are released following injury to the tissue.
Table 57-1. Primary Afferent Fibers and their Function | ||||||||||||||||||||||||||||||||||||||||||
---|---|---|---|---|---|---|---|---|---|---|---|---|---|---|---|---|---|---|---|---|---|---|---|---|---|---|---|---|---|---|---|---|---|---|---|---|---|---|---|---|---|---|
|
The primary afferent peripheral (distal) terminals express a variety of specific transducer channels that are sensitive over a range of stimulus intensities. When they are activated by the appropriate stimulus (thermal, chemical, or mechanical) these channels activate voltage-sensitive cation channels (NaV and CaV) and initiate an action potential. The sodium channel is of special interest following the discovery of its involvement in patients that are insensitive to pain. An epidemiologic study identified three Pakistani families with congential insensitivity to pain. All were found to possess nonsense mutations of the SCN9A gene resulting in truncation of the Nav1.7 isoform of the sodium channel.7 Other mutations of this gene result in the impaired inactivation of this channel resulting in paroxysmal extreme pain disorder or hyperexcitability resulting in erythromelalgia.8,9
The understanding of the molecular underpinning of the sensory processing of pain has primarily come from studying two families of proteins, the TRP and the DEG/ENaC channels. The cloning and characterization of the “capsaicin” receptor of the transient receptor potential (TRP) family of nonselective cation channels expanded the field immensely10 (Table 57-2). Members of this molecular family transduce thermal, mechanical, and chemical information in the periphery. The capsaicin receptor named TRP vanilloid 1 (TRPV1), which responds not only to capsaicin and other vanilloid compounds, but which is also activated by acid and heat, provides an excellent example of the integration of multiple sensory modalities within a single neuron and is localized to nociceptors.10 Furthermore, acidic environments can lower the activation threshold of the channel to heat stimuli. Therefore, the TRPV1 receptor may represent an important therapeutic target in inflammatory (acidic) pain conditions. Mice lacking the TRPV1 receptor are deficient in their response to thermal, but not mechanical or other noxious stimuli.11 These data suggest that this member of the family of TRP channels may play a role in the integration of noxious chemical and thermal stimuli while having relatively less to do with mechanical transduction. Although mechanical transduction has been less well characterized than either thermal or chemical nociceptive transduction, there is evidence of mechanically activated channels in the degenerins family of the nematode Caenorhabditis elegans. In mammals, studies have provided evidence that the transduction channel is a complex of degenerin and the epithelial Na+ channel (DEG/ENaC)12 and may have a role in the transduction of mechanical stimuli in humans. The subunit composition of the DEG/ENaC channel may play a role in determining whether a mechanical stimuli is sensed as noxious or innocuous.13
Neurochemistry of Peripheral Nerve and the Dorsal Root Ganglion
The nociceptive primary afferents, the Aδ and C fibers, represent the principal target of pharmacologic manipulation by the physician treating pain. Glutamate receptors, as well as opioid, substance P, somatostatin, and vanilloid receptors, have been identified on the peripheral endings of these nerve fibers. Although the transmission of acute nociceptive information is primarily by the Aδ and C fibers, a subset of the Aδ and C fibers are “thermoreceptors” that transduce innocuous cold and warm information, respectively. The cell bodies of primary afferents, regardless of the structure they innervate, make up the dorsal root ganglia (DRG) located just outside the spinal cord within the bony foramen.
Primary afferent activation results in a postsynaptic excita-tory event in the spinal cord. Glutamate is the primary
neurotransmitter serving this function. Acute activation events are mediated by the AMPA-type (α-amino-3-hydroxy-5-methyl-4-isoxazole propionic acid) glutamate receptor present on the dorsal horn neurons. This receptor produces a robust, but short-lasting depolarization of the postsynaptic membrane by increasing sodium conductance and augmenting the activation of the NMDA-type (N-methyl-D-aspartate) glutamate receptor. In addition to glutamate, populations of primary afferents contain and release a variety of neuropeptides including substance P, calcitonin gene–related peptide, adenosine triphosphate, adenosine, galanin, and somatostatin and growth factors including brain-derived nerve growth factor.14
neurotransmitter serving this function. Acute activation events are mediated by the AMPA-type (α-amino-3-hydroxy-5-methyl-4-isoxazole propionic acid) glutamate receptor present on the dorsal horn neurons. This receptor produces a robust, but short-lasting depolarization of the postsynaptic membrane by increasing sodium conductance and augmenting the activation of the NMDA-type (N-methyl-D-aspartate) glutamate receptor. In addition to glutamate, populations of primary afferents contain and release a variety of neuropeptides including substance P, calcitonin gene–related peptide, adenosine triphosphate, adenosine, galanin, and somatostatin and growth factors including brain-derived nerve growth factor.14
Table 57-2. Mechanosensory and Thermosensory Transduction Channels Found in Mammals | ||||||||||||||||||||||||||||||||||||||||||||||||||||||||||||||||||||||||||||||
---|---|---|---|---|---|---|---|---|---|---|---|---|---|---|---|---|---|---|---|---|---|---|---|---|---|---|---|---|---|---|---|---|---|---|---|---|---|---|---|---|---|---|---|---|---|---|---|---|---|---|---|---|---|---|---|---|---|---|---|---|---|---|---|---|---|---|---|---|---|---|---|---|---|---|---|---|---|---|
|
Neurobiology of the Spinal Cord and Spinal Trigeminal Nucleus
Primary afferent fibers enter the gray matter of the spinal cord through the dorsal root entry zone and innervate the spinal cord. The majority of heavily myelinated primary afferent fibers (Aα, Aβ) carrying sensory information, including tactile, pressure, and vibratory sense, enter in dorsal roots, traverse across the top of the dorsal horn of the spinal cord (Lissauer’s tract), and ascend ipsilaterally within the dorsal column and provide collateral branches into the gray matter of the dorsal horn. The small-diameter lightly myelinated and the small-diameter unmyelinated fibers transmitting temperature and nociceptive information enter Lissauer’s tract and innervate the gray matter of the spinal cord. Unlike the heavily myelinated fibers, these fibers may also ascend rostrally or descend caudally through Lissauer’s tract before they innervate adjacent spinal levels.
The gray matter of the spinal cord is made up of synaptic terminations of primary afferents and the second-order neurons that form the first stage of processing and integration of sensory information. The gray matter of the spinal cord is divided into 10 laminae on the basis of histologic appearance. The dorsal horn includes laminae I to VI and represents the primary sensory complement of the spinal cord (Fig. 57-1). The ventral horn, including laminae VII to IX and lamina X, is involved in somatic motor and autonomic functions, respectively. Somatic C-fiber nociceptive afferent endings primarily terminate in the laminae I and II of the same and/or one to two adjacent spinal segments from which they entered from the periphery, whereas visceral C-fiber nociceptive afferents can terminate in the dorsal horn more than five segments rostrally or caudally. They not only terminate in laminae I, II, V, X ipsilaterally but also in laminae V and X contralaterally. Therefore, visceral afferents have a wider branching pattern and the nociceptive information they transmit is less localizable to a particular area of the body.
In addition to the primary afferent endings, neurons of the descending pathways and local interneurons also innervate the superficial dorsal horn (laminae I and II). The outer marginal layer or lamina I contain interneurons and cells that send axonal projections to the brainstem and midbrain structures. The substantia gelatinosa, or lamina II, also contains excitatory and inhibitory interneurons but fewer projection neurons. Laminae III and IV contain interneurons and the second-order neurons that make up the dorsal column pathways relaying nonnociceptive sensory and proprioceptive information. Laminae IV to VI contain interneurons and a modest portion of nociceptive projection neurons that distribute input to the brainstem and thalamus.
Nociceptive somatic input is primarily transmitted by second-order lamina I, IV, and V projection neurons as the contralateral spinothalamic tract (STT) pathway traveling to numerous brainstem regions and the thalamus.15 There is a nociceptive visceral processing area in laminae III, IV, V, VII, and X. The visceral nociceptive input is relayed by second-order neurons whose axons travel within the dorsal column. Like the fibers transmitting nonnoxious sensory information, these fibers remain uncrossed until relayed with the crossed medial lemniscal fibers to the thalamus. The visceral pain information transmitted by the ventral STT is likely originating from cells also receiving somatic nociceptive input. Nociceptive and nonnociceptive sensory information from the head, neck, and dura transmitted via the trigeminal nerve innervates the dorsal horn of the spinal trigeminal nucleus in the caudal medulla. The organization and neurotransmitter complement of the spinal trigeminal nucleus is similar to that of the spinal dorsal horn.
Neurobiology of Ascending Pathways
Dorsal Column Tracts
The dorsal column contains the axons of second-order spinal cord projection neurons in addition to the ascending axons of primary afferent neurons relaying touch, pressure, and vibratory sensation. Second-order dorsal column cells in the central visceral processing region of the spinal cord around lamina X also respond to noxious visceral stimulation and converge on some of the thalamic cells receiving nociceptive information from the skin and other somatic structures.
Spinothalamic Tract (STT)
STT neurons are the primary relay cells providing nociceptive input from the spinal cord to supraspinal levels. The axons of
STT cells cross the midline of the spinal cord through the anterior white commissure and ascend primarily in the contralateral and anterolateral tracts. The axons of STT cells terminate primarily in the posterior complex of the thalamus including the ventral posterior lateral and ventral posterior medial nuclei. Nonnoxious sensory input from the same body region converges on the same target thalamic neurons providing somatotopic encoding for localization of the input onto the cortical representation of the specific body region, allowing the ability to locate the source of the nociceptive input. The STT cells receiving noxious somatic input are predominantly situated in lamina I and the lateral aspect of the dorsal horn in laminae IV to V.15 However, other STT neurons are scattered throughout the deep dorsal horn, intermediate region including lamina X, and even in lamina VII of the ventral horn. These STT cells receive both somatic and visceral nociceptive information.
STT cells cross the midline of the spinal cord through the anterior white commissure and ascend primarily in the contralateral and anterolateral tracts. The axons of STT cells terminate primarily in the posterior complex of the thalamus including the ventral posterior lateral and ventral posterior medial nuclei. Nonnoxious sensory input from the same body region converges on the same target thalamic neurons providing somatotopic encoding for localization of the input onto the cortical representation of the specific body region, allowing the ability to locate the source of the nociceptive input. The STT cells receiving noxious somatic input are predominantly situated in lamina I and the lateral aspect of the dorsal horn in laminae IV to V.15 However, other STT neurons are scattered throughout the deep dorsal horn, intermediate region including lamina X, and even in lamina VII of the ventral horn. These STT cells receive both somatic and visceral nociceptive information.
Spinobulbar Pathways
Major ascending lateral axonal projections relaying information about noxious stimuli terminate in the reticular formation of the ventrolateral medulla. This spinal projection pathway traverses brainstem regions containing catecholaminergic neurons, including the locus coeruleus and A7 nuclei of the dorsolateral pontine tegmentum (DLPT). These catecholaminergic neurons are involved in multiple functions, including modulation of nociceptive transmission through the spinal cord via descending inhibitory input. Connections between the rostral ventromedial medulla (RVM) and the catecholamine cells of the pons and brainstem serotonergic cells also play a role in descending modulation of noxious transmission in the spinal cord.16 Spinobulbar pathways relaying information about pain also terminate in the parabrachial nucleus, periaqueductal gray (PAG), the RVM, and midline midbrain reticular formation.17
Spinohypothalamic, Limbic, and Cortical Connections
Pain is a sensory experience but also has an affective component to the perception of noxious stimuli. Pain can provoke fear, anxiety, and depression, resulting in autonomic responses including increased heart rate and blood pressure as well as the endocrine stress response. These responses to noxious stimuli are thought to be mediated by the spinohypothalamic and spinoamygdalar pathways. In addition to their affective function, these regions are also thought to be involved in antinociception. Ascending axonal projections of these pathways arise predominantly from the spinal cord laminae I and X.
Neurobiology of Descending Pathways
The primary components of this descending pain inhibition system, but certainly not all-inclusive, is the “triad” of the PAG, the RVM, and the DLPT.17 The PAG is an important site for the production of antinociception following electrical or chemical activation, or the injection of opioid receptor agonists. The endogenous opioid enkephalin is present within this nucleus, and opioid receptors of each subtype are present in this region. The PAG provides dense projections to the RVM, the locus coeruleus, and A7 nuclei.18 Although each of these regions has direct projections to the spinal cord, it has been proposed that their projections to the RVM are important components in the modulation of nociception. Chemical or electrical inactivation of the RVM results in the attenuation of the antinociceptive effects produced by the activation of these midbrain structures.19 Although the RVM can function as a relay nucleus in the production of antinociception by more cephalad midbrain structures including the PAG, it also has a primary role in the suppression of nociceptive transmission at the level of the spinal cord. The suppression of nociceptive reflex behavior is mediated by the axons of RVM neurons that descend within the dorsolateral funiculus and terminate bilaterally in laminae I, II, V, VI, and VII of the spinal cord. Anatomic studies have shown that these axons terminate coincident with interneurons of the dorsal horn that are related to nociceptive transmission.20 Consistent with the anatomic terminations of the RVM axons, physiologic studies have shown that stimulation of the RVM results in the inhibition of a population of nociceptive-specific neurons within the dorsal horn as well as selective inhibition of the nociceptive responses of wide-dynamic range neurons.21 The neurotransmitters found in the RVM neurons include enkephalin, γ-aminobutyric acid (GABA), glutamate, and substance P.22 The DLPT is also an important component of spinal cord nociceptive modulation. It contains all of the noradrenergic neurons that project to the RVM and the spinal cord, and electrical stimulation of the DLPT sites produces spinal cord α2-adrenergic receptor–mediated analgesia.23
Neurobiology of Supraspinal Structures Involved in Higher Cortical Processing
Higher cortical centers play a role in the perception of painful stimuli as well as the integration of the sensory-discriminative and affective components of the noxious stimulation. The localization of the neural structures involved in this perception and integration is still in its adolescence. The development of positron emission tomography and functional magnetic resonance imaging technologies has moved this research forward. These imaging technologies produce indirect evidence of neural activity related to pain stimulation. They look for areas of increased blood flow as an indicator of regions of increased activity resulting from the stimulation. The primary and secondary somatosensory cortexes, the anterior cingulate gyrus, the insula, and the prefrontal cortex appear to be involved in the higher processing of somatic and visceral pain.24 As the primary and secondary cortexes are known to be somatosensory processing regions, the imaging studies are consistent with a sensory-discriminative role of these structures. The insula and frontal cortex may contribute to memory and learning of events related to painful stimuli. The anterior cingulate cortex is thought to be involved in the analysis of the emotional significance of the painful input. Finally, the lentiform nucleus and cerebellum may be involved in the learning of self-protective reflexive motor responsiveness to painful input.
Transition from Acute to Persistent or Chronic Nociception
Pain sensation is unique among the somatosensory modalities in that it does not rapidly adapt to prolonged stimulation as do the other sensory modalities, such as fine touch. In fact, continued stimulation may produce greater noxious sensation or reduce the stimulus threshold or intensity that is necessary for the appreciation of the sensation as noxious. For instance, previously innocuous thermal or mechanical stimulation may be perceived as painful following a prior noxious stimulus. For example, warm water of the shower or the light touch of the towel across sunburned skin produces a painful sensation that may persist for a few minutes
following the stimulation. This is termed allodynia. Another example of an altered pain state that may follow an acute injury is that of hyperalgesia, in which a previously noxious stimulus is perceived as more painful. The sensation of increased intensity of noxious stimulation at the site of the injury is the result of the sensitization of the peripheral nociceptors.
following the stimulation. This is termed allodynia. Another example of an altered pain state that may follow an acute injury is that of hyperalgesia, in which a previously noxious stimulus is perceived as more painful. The sensation of increased intensity of noxious stimulation at the site of the injury is the result of the sensitization of the peripheral nociceptors.

In addition to the alteration of the chemical milieu surrounding the primary afferent distal terminal that results from injury or persistent high-intensity stimulation, axonal sprouting and the formation of neuroma may occur. The neuroma may have an altered complement of ion channels including an up-regulation of sodium channels or a down-regulation of potassium channels that has the net result of increasing neuronal excitability and increasing nociceptive transmission. It has been shown that, following nerve damage, an increase in the expression of sodium channels occurs in the neuroma and the DRG. Numerous sodium channels exist on primary afferents; Nav1.8 and 1.9 subtypes are primarily found on C-fiber DRG cells. Genetic “knock-down” or removal of the Nav1.8 channel had no effect on baseline pain thresholds; however, it reversed nerve injury evoked nociception.26 Also following nerve damage, potassium currents have been shown to be reduced, suggesting a reduction in these channels contributing to spontaneous nociceptive activity. Consistent with this notion, it has been observed that potassium channel antagonists increase and potassium channel agonists decrease ectopic firing after peripheral nerve injury.27 The HCN2 ion channel which carries an inward current Ih that is activated by neuronal hyperpolarization may have a significant role in the sensation of chronic pain from neuropathy or inflammation.28 When this gene product was knocked out of nociceptive primary sensory neurons in mice, the mice failed to express pain behaviors after inflammatory injuries. The mice did not show hyperalgesia or allodynia to mechanical or thermal stimuli in a model of neuropathic injury. The pharmacologic blockade of this channel, or disruption of this gene, could be a potential therapy for those suffering from some conditions of chronic pain.
Neuromas of injured primary afferents have altered sensitivity to a number of humoral factors, including cytokines, prostaglandins, and catecholamines. These factors are released from a variety of cell types including inflammatory cells and neuronal support cells. Cytokines directly activate the nerve and neuroma through receptors that become expressed in the membrane after the nerve injury. A molecule that has been shown to have a prominent role following nerve damage is tumor necrosis factor subunit alpha (TNF-α).29 Shortly after injury, TNF-α decreases potassium conductance, increasing neuronal excitability, while the long-term changes may be produced through the activation of second messenger systems, resulting in altered protein production. Application of TNF-α to the peripheral nerve results in hyperalgesia, while systemic delivery of antibodies to TNF-α or TNF-α–binding protein reduces neuropathic pain.
Prostaglandins are also released from inflammatory cells following nerve and tissue damage. They can enhance the opening of Nav1.8 channels by acting though receptors on the afferent terminal. Nerve growth factor is also released from glial and inflammatory cells after nerve damage, resulting in sprouting of postganglionic sympathetic efferents into the site of injury. Protease-activated receptors isoforms 1, 2, and 4 appear to sensitize TRPV1 channels in nociceptive neurons and are involved in mechanisms of pain and inflammation.30 These receptors are responsive to thrombin activation and could account for pain resulting from a hematoma. The up-regulation of α1-adrenergic receptors has been demonstrated in animals with nerve injuries.31 Stimulation of the postganglionic axons results in the release of catecholamines and excites the injured axon and DRG of the injured axon via α-adrenergic receptors.
Although acute noxious stimuli are transmitted to the spinal cord via Aδ and C fibers, the presence of allodynia is thought to be mediated by the activation of large-diameter Aβ fibers through what has been termed a phenotypic switch.2 Prior to this peripheral injury, the Aβ fibers, unlike the C fibers, do not express substance P. However, following injury these fibers were able to express this neuropeptide.32 These data therefore implicate Aβ fibers in the transmission of noxious peripheral stimulation and provide further support for the involvement of somatic Aβ fibers in at least some form of the allodynic pain states. Furthermore, the blockade of Aβ fibers results in a reduction in light-touch evoked allodynia.33 This phenotypic switch of Aβ fibers may represent another avenue for therapeutic intervention; however, the difficulty will be in differentiating between those Aβ fibers involved in noxious versus nonnoxious sensory information.
Management of Common Pain Syndromes
Low Back Pain: Radicular Pain Syndromes
The common causes of low back pain include radicular pain/radiculopathy from herniated disc or spinal/foraminal stenosis, facet syndrome, and internal disc disruption. Myofascial pain syndrome also causes back pain, whereas sacroiliac joint syndrome and piriformis syndrome cause mostly buttock pain but can present as low back pain or radicular pain. Radicular symptoms of pain, paresthesias, and numbness in a typical dermatomal distribution in the presence of objective signs of weakness, diminished reflexes, and positive straight-leg raise are secondary to pathology or dysfunction of the sensory spinal nerve roots. Low back pain, with or without radicular pain, is mostly due to lesions of the intervertebral discs and degenerative spinal disorders. Other causes include spinal metastasis, vertebral body fractures, infections, abdominal aortic aneurysm, and chronic pancreatic lesions.
Low back and radicular pain secondary to a herniated disc is due to mechanical nerve root compression and the subsequent inflammatory process. The presence of a herniated disc does not necessarily result in pain. Up to 36% of the general population34 and up to 53% of pregnant women35 can have an asymptomatic
herniated disc. Follow-up studies on patients with a herniated disc show spontaneous regression without treatment, absence of symptoms in the presence of more abnormalities, and partial or complete resolution with treatment that includes medications, bed rest, physical therapy, traction, or epidural steroids.36 If symptomatic, the patient usually presents with low back pain and radicular symptoms that include paresthesias as well as numbness and weakness in the distribution of the involved nerve root. Radicular pain typically travels along a narrow band and has a sharp, shooting, and lancinating quality. Gait disturbances, loss of sensation, reduced muscle strength, and diminished reflexes involve the appropriate affected dermatomal distribution.
herniated disc. Follow-up studies on patients with a herniated disc show spontaneous regression without treatment, absence of symptoms in the presence of more abnormalities, and partial or complete resolution with treatment that includes medications, bed rest, physical therapy, traction, or epidural steroids.36 If symptomatic, the patient usually presents with low back pain and radicular symptoms that include paresthesias as well as numbness and weakness in the distribution of the involved nerve root. Radicular pain typically travels along a narrow band and has a sharp, shooting, and lancinating quality. Gait disturbances, loss of sensation, reduced muscle strength, and diminished reflexes involve the appropriate affected dermatomal distribution.
Inflammation in the spinal canal secondary to a herniated disc plays an important role in the causation of back and radicular pain. Herniated nucleus pulposus results in local release of cytokines and other inflammatory mediators that cause a chemical radiculitis. High levels of phospholipase A2 activity were noted in human disc fragments removed at surgery from patients with symptomatic radiculopathy. Increased levels of the inflammatory cytokines interleukin-6 and interleukin-8 were noted from disc material taken from patients with known disc disease.37 The application of disc material onto spinal nerve roots can induce functional and morphologic changes in the nerves. Disc cells express TNF-α, which, when applied to spinal nerve roots, causes similar changes to those seen after application of disc material; selective inhibition of TNF-α may reduce the intraneural edema.38 A double-blind, placebo-controlled study showed that an intradiscal injection of 1.5 mg of etanercept, a TNF-α inhibitor, in a pain-generating disc did not reduce the pain scores or disability scores of patients with chronic discogenic pain or lumbosacral radiculopathy.39

A transforaminal approach can be employed to deposit steroid in the anterolateral epidural space where the herniated disc is located, through the intervertebral foramina, and distally along the nerve root (Fig. 57-2). This approach is especially indicated in radicular pain specific to a single nerve root. Prospective, randomized studies on transforaminal ESIs show the same results as with the interlaminar approach, that is, short-term efficacy of the injection.47,48,49 The transforaminal approach has a better rationale than the midline interlaminar approach, and studies that compared the two approaches show better efficacy with the transforaminal approach.50,51 A study comparing the parasagittal (paramedian approach) and transforaminal approach showed a more ideal spread of the contrast dye in the parasagittal approach although the efficacy was similar between the two techniques.52
It is advisable that fluoroscopy be used in ESI, especially with the transforaminal approach, to assure insertion of the needle at the affected vertebral level and document the flow of the contrast
medium (and the drug). Reassessment should be carried out 2 to 3 weeks after the initial injection. The use of multiple ESIs in a patient, with a short interval between injections, is not advised. If there is no response to an initial injection, it can be repeated once as some patients require a second injection before they respond. If there is partial response, up to three injections can be performed.
medium (and the drug). Reassessment should be carried out 2 to 3 weeks after the initial injection. The use of multiple ESIs in a patient, with a short interval between injections, is not advised. If there is no response to an initial injection, it can be repeated once as some patients require a second injection before they respond. If there is partial response, up to three injections can be performed.
![]() Figure 57.2. Right L5 transforaminal epidural injection. Note the spread of the contrast medium proximally into the lateral epidural space and distally along the nerve root. |
The complications of ESI may be due to the technique or from the injected drug, as well as the vehicle and additives. Complications related to the technique include needle trauma, vasospasm, and infection. Glucocorticoids reduce the hypoglycemic effect of insulin and interfere with blood glucose control in patients with diabetes mellitus. Insulin sensitivity may be impaired, there may be no change in the HbA1C levels, or the blood glucose can be increased for 1 week after ESIs. A single dose of 80 mg of methylprednisolone can suppress plasma cortisol levels and the ability to secrete cortisol in response to synthetic corticotropin for up to 3 weeks. Epidural triamcinolone, 80 mg, can suppress serum cortisol and corticotropin levels for up to 7 days after injection. The median recovery to normal levels occurs within 1 month after the last injection, and full recovery is at 3 months.
Injury to the brain or spinal cord can occur with transforaminal ESIs.53 The cerebral/cerebellar events can be ascribed to trauma to the vertebral artery, vasospasm from the injected steroid or dye, or embolism of the particulate steroid via the vertebral artery.54,55 The spinal cord injuries can be ascribed to injury to the radicular artery accompanying the nerve root, spasm of the radicular artery from the injected dye or steroid, embolism of the particulate steroid, or from proximal intraneural spread of the injectate. The injection of contrast medium through a radicular artery that passed to the spinal cord or the anterior spinal artery has been demonstrated. The occurrence of adverse events at the lumbar level has been ascribed to intra-arterial injection into an abnormally low-lying artery of the Adamkiewicz. These adverse events have also been described after injection of local anesthetic or dye, without steroid.55 The use of computed tomography (CT), instead of fluoroscopy, does not assure avoidance of the adverse events.
Huntoon56 noted that the vertebral, ascending cervical, and deep cervical arteries supply segmental medullary vessels and that the ascending and deep cervical arteries are within 2 mm of the site of insertion of the needle for cervical transforaminal ESIs. The proximity of these arteries to the site of needle placement makes these blood vessels vulnerable to trauma or unintentional sites of injection of the steroid. Occlusion of the vessels occurs from the particulate steroids. Methylprednisolone acetate has the largest particle size, betamethasone the smallest particles, and triamcinolone acetonide is in between55 (Fig. 57-3). Dexamethasone has no identifiable particles. Dexamethasone appears to be ideal for transforaminal ESIs; however, it is easily washed out from the epidural space, and studies on its efficacy are only preliminary.57 The following steroids are recommended for ESIs: (1) Methylprednisolone, triamcinolone, or betamethasone for interlaminar injections; (2) betamethasone (preferably) or triamcinolone for lumbar transforaminal injections; and (3) dexamethasone for cervical transforaminal injections.
ESIs are more effective in patients with acute radicular symptoms; however, they are not effective in patients with chronic lumbar radiculopathy.58 There have been several prospective, randomized controlled studies on the efficacy of surgery in relieving back pain secondary to herniated disc. It appears that surgery for herniated disc produces only short-term relief, whereas the long-term results are comparable with those with conservative management.59 For spinal stenosis, surgery is associated with greater improvements in most outcome measures.
Low Back Pain: Facet Syndrome
Patients with low back pain secondary to facet pathology have pain in the low back that radiates to the ipsilateral posterior thigh and usually ends at the knee. On physical examination there is paraspinal tenderness and reproduction of pain with extension–rotation maneuvers of the back. The diagnosis of facet syndrome is arrived at by a combination of the patient’s history, physical examination findings, and a positive response to diagnostic medial branch blocks or facet joint injections (Fig. 57-4). For medial branch blocks, some investigators recommend the use of local anesthetics with different durations of effect (e.g., lidocaine and bupivacaine) and to correlate the duration of relief with the known duration of effect of the drug.
Some patients may have a prolonged response to facet joint injections, that is, up to 3 to 6 months. If the patient has a prolonged response, it is best to wait for recurrence of the pain. If the relief is short-lived, especially after medial branch blocks, then thermal
radiofrequency (RF) rhizotomy of the medial branches should be performed. It appears that there is no relationship between the mean sensory stimulation threshold (which denotes proximity of the electrode to the nerve) during lumbar facet rhizotomy denervation and treatment outcome.60 Randomized controlled studies have shown improvements after thermal RF of the lumbar medial branches that lasted 3 to 12 months61,62,63,64,65,66,67 (Table 57-3). With regard to cervical facet syndrome, controlled studies on thermal RF of the cervical medial branches have shown different results. Lord et al.68 showed better results after RF (return to work and relief of pain) while Stovner et al.69 showed no difference between the RF and sham procedures.
radiofrequency (RF) rhizotomy of the medial branches should be performed. It appears that there is no relationship between the mean sensory stimulation threshold (which denotes proximity of the electrode to the nerve) during lumbar facet rhizotomy denervation and treatment outcome.60 Randomized controlled studies have shown improvements after thermal RF of the lumbar medial branches that lasted 3 to 12 months61,62,63,64,65,66,67 (Table 57-3). With regard to cervical facet syndrome, controlled studies on thermal RF of the cervical medial branches have shown different results. Lord et al.68 showed better results after RF (return to work and relief of pain) while Stovner et al.69 showed no difference between the RF and sham procedures.
Buttock Pain: Sacroiliac Joint Syndrome and Piriformis Syndrome
The pain of sacroiliac joint syndrome is located in the region of the affected sacroiliac joint and the medial buttock. The pain may
radiate to the groin, posterior thigh, and occasionally below the knee. Physical examination usually reveals tenderness over the sacroiliac sulcus, reduction in the joint mobility, and reproduction of the pain when the affected sacroiliac joint is stressed. The most commonly used tests for sacroiliac joint dysfunction include the FABER Patrick, Gaenslen, Yeoman, sacroiliac shear, and Gillet tests. The FABER Patrick and the Yeoman test do not rule out hip pathology while the Yeoman and the shear tests are more specific for sacroiliac joint syndrome. The presence of symptoms and physical examination findings suggestive of SI joint syndrome, pain on three of the tests, and a positive response to sacroiliac joint injection are adequate to make the diagnosis of sacroiliac joint syndrome.
radiate to the groin, posterior thigh, and occasionally below the knee. Physical examination usually reveals tenderness over the sacroiliac sulcus, reduction in the joint mobility, and reproduction of the pain when the affected sacroiliac joint is stressed. The most commonly used tests for sacroiliac joint dysfunction include the FABER Patrick, Gaenslen, Yeoman, sacroiliac shear, and Gillet tests. The FABER Patrick and the Yeoman test do not rule out hip pathology while the Yeoman and the shear tests are more specific for sacroiliac joint syndrome. The presence of symptoms and physical examination findings suggestive of SI joint syndrome, pain on three of the tests, and a positive response to sacroiliac joint injection are adequate to make the diagnosis of sacroiliac joint syndrome.
Table 57-3. Randomized, Controlled Studies on Radiofrequency Denervation of the Medial Branches (Facet Nerves) | |||||||||||||||||||||||||||
---|---|---|---|---|---|---|---|---|---|---|---|---|---|---|---|---|---|---|---|---|---|---|---|---|---|---|---|
|
The treatments for sacroiliac joint syndrome include physical therapy, manipulation, intra-articular steroid injections (Fig. 57-5), radiofrequency denervation, and surgical fusion of the joint. Physical therapy and chiropractic manipulations are used extensively for the treatment of sacroiliac joint disease; however, there is no large outcome study validating their use. Intra-articular injections of steroid (40 to 80 mg of methylprednisolone or other depot steroid) and local anesthetic into the sacroiliac joint results in a few months of pain relief, but again, no prospective controlled studies support their use.
Denervation of the lower portion of the sacroiliac joint may be achieved by the creation of bipolar RF strip lesions along the dorsal border of the sacroiliac joint in a leapfrog manner. Local anesthetic blockade of the sensory innervation of the dorsal portion of the sacroiliac joint—the medial branch of the dorsal rami of L5 and the lateral branches of the dorsal rami of S1 to S3—can be performed initially or when the relief from the sacroiliac joint injection is temporary. Relief from the local anesthetic block may last weeks to months when combined with physical therapy. Monopolar and bipolar thermal radiofrequency lesioning of the lateral branches is performed for a more lasting relief. The bigger lesions created by the water-cooled radiofrequency technique (Fig. 57-6)70 are inherently more effective since it accommodates for the variations in the location of the lateral branches along the lateral border of the sacral foraminas.71
Piriformis Syndrome
Piriformis syndrome, another pain syndrome that originates in the buttock, comprises 5% to 6% of patients referred for the treatment of back and leg pain. It occurs after trauma, surgery, and infection, or from compression of one of the components of the sciatic nerve as it runs between two divisions of the piriformis muscle.72 Patients with piriformis syndrome complain of buttock pain with or without radiation to the ipsilateral leg. The buttock pain usually extends from the sacrum to the greater trochanter of the femur whereas irritation of the sciatic nerve results in a buttock pain that radiates to the ipsilateral leg. Prolonged sitting, as in driving or biking, or getting up from a sitting position aggravates the pain. The pain is aggravated by hip flexion, adduction, and internal rotation. Neurologic examination is usually negative. There may be leg numbness when the sciatic nerve is irritated; the straight-leg test may be normal or limited. Three signs confirm the presence of piriformis syndrome72: (1) The Pace sign wherein there is pain and weakness on resisted abduction of the hip in a patient who is seated with the hip flexed; (2) the Lasègue sign wherein there is pain on flexion, adduction, and internal rotation of the hip in a patient who is supine (note that some clinicians call pain on straight-leg raise the Lasègue sign also); and (3) the Freiberg sign wherein there is pain on forced internal rotation of the extended thigh. Note that the piriformis is an internal rotator of the flexed hip and an external rotator of the extended hip. The diagnosis of piriformis syndrome is made on clinical grounds. Electromyography may detect myopathic and neuropathic changes including a delay in the H-reflex with the affected leg in a flexed, adducted, and internally rotated (FAIR) position as compared with the same H-reflex in the normal anatomic position.73 The CT scan of the soft tissues of the pelvis may show an enlarged piriformis muscle, or abnormal uptake by the muscle, and the MRI confirms an enlarged piriformis muscle.
The treatments of piriformis syndrome include physical therapy combined with medications including muscle relaxants, anti-inflammatory drugs, and analgesics to reduce the spasm, inflammation, and pain. Local anesthetic and steroid injections into the piriformis muscle may break the pain/muscle spasm cycle. Although blind injections can be done, more specific techniques involve identification of the piriformis muscle with muscle electromyography, CT guidance, use of a nerve stimulator, or combined fluoroscopy–nerve stimulator guidance.72 If relief from the local anesthetic does not last, then the piriformis muscle is injected with 100 units of botulinum toxin A in 2 to 3 mL of local anesthetic. The reported complications of botulinum toxin injection include brachial plexopathy, polyradiculoneuritis, and local psoriasiform dermatitis, so precautions should be followed to assure injection of the botulinum toxin into the belly of the piriformis muscle.
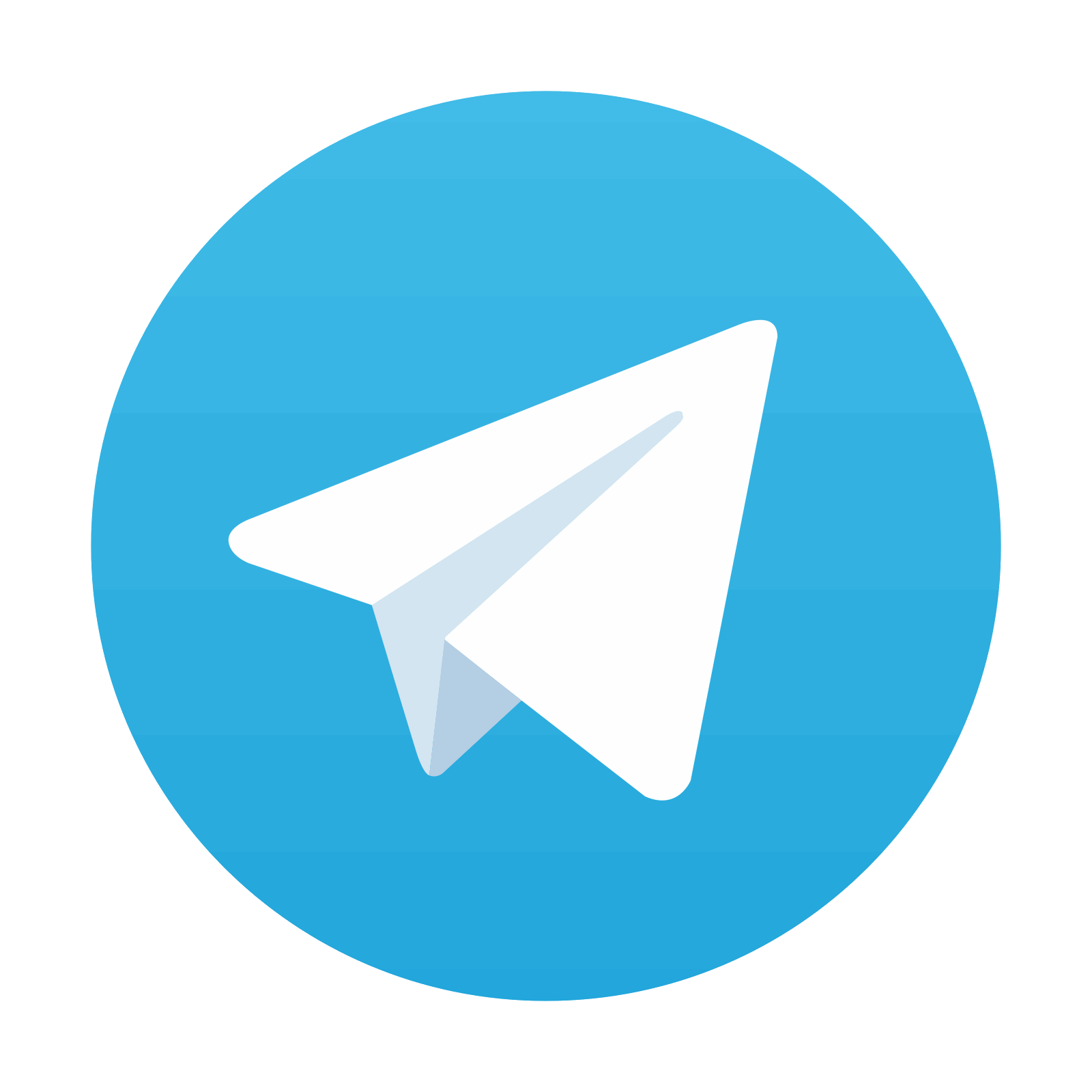
Stay updated, free articles. Join our Telegram channel

Full access? Get Clinical Tree
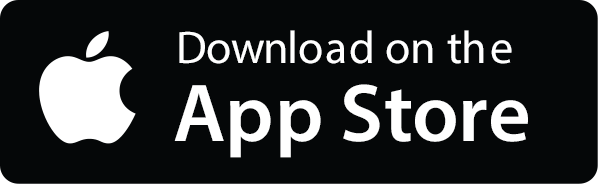
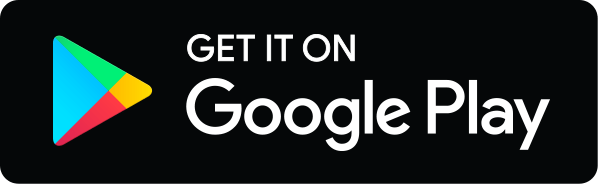
