Abstract
In the previous chapter we explored the mathematical principles and models that can be used to predict plasma concentrations of drugs, particularly compartment models. This chapter will discuss the use of drugs for which commercial infusion devices are available that are programmed with kinetic models, in particular propofol and remifentanil.
In the previous chapter we explored the mathematical principles and models that can be used to predict plasma concentrations of drugs, particularly compartment models. This chapter will discuss the use of drugs for which commercial infusion devices are available that are programmed with kinetic models, in particular propofol and remifentanil.
Ideal Drugs for Intravenous Use in Anaesthesia
Total intravenous anaesthesia (TIVA) describes the provision of anaesthesia by intravenous infusions alone. Anaesthesia may be induced and maintained either by an infusion of a hypnotic alone or by a combination of hypnotic and analgesic. An ideal drug for induction and maintenance of anaesthesia should have the following properties:
predictable plasma concentrations according to a known pharmacokinetic model
predictable link between pharmacokinetic and pharmacodynamic effects
no active metabolites
a rapid onset of action
a rapid offset of action
stable in a plastic syringe.
The delivery of TIVA has been improved by pumps that contain algorithms specific to the anaesthetic agents with the most favourable kinetic profiles. These pumps deliver infusions to maintain a constant (plasma or effect site) concentration – target-controlled infusions (TCIs). Propofol and remifentanil are the commonest combination of hypnotic and analgesic, each being delivered by a separate pump so that either can be altered independently of the other. A mixture of propofol and alfentanil has been used via a single pump for shorter cases, although the target concentration of alfentanil is impossible to control directly with this technique.
Propofol
The intravenous anaesthetic propofol can be used both for induction and maintenance of anaesthesia, largely due to its favourable ‘wake up’ profile. Although it is possible to give a bolus dose of propofol without understanding its pharmacokinetics, to use it sensibly by infusion requires some understanding of kinetics.
Propofol is a sterically hindered phenol: the potentially active hydroxyl (-OH) group is shielded by the electron clouds surrounding the attached isopropyl ((CH3)2CH-) groups, which reduces its reactivity. So, unlike phenol, propofol is insoluble in water but highly soluble in fat hence its preparation as a lipid emulsion. The phenolic hydroxyl group makes propofol a weak acid, but significant ionisation occurs only at a pH greater than 10. High lipid solubility means that after long infusions there is the risk of accumulation in fatty tissues. There are no active metabolites of propofol. The combination of high lipid solubility and insignificant ionisation means that once propofol distributes into fat it will redistribute back into plasma relatively slowly. The extremely large volume of distribution of propofol is accounted for mainly by the third compartment and although a relatively large amount of drug, in terms of milligrams, may distribute into this volume, the concentration rises only very slowly.
An induction dose of propofol is extensively bound to plasma proteins (98% bound to albumin) following intravenous injection. After the initial bolus dose there is rapid loss of consciousness as the lipid tissue of the central nervous system (CNS) takes up the highly lipophilic drug. Over the next few minutes propofol then distributes to peripheral tissues and the concentration in the CNS falls (see Figure 7.1), such that in the absence of further doses or another anaesthetic agent the patient will wake up. Its distribution half-life is 1–2 minutes and accounts for the rapid fall in plasma levels with a short duration of action. The very rapid elimination of propofol by hepatic and extra-hepatic metabolism also contributes significantly to this rapid reduction in plasma concentration. The terminal elimination half-life is very much longer (5–12 hours) reflecting relatively slow redistribution from the fatty tissues and plays an insignificant role in offset of clinical action following a bolus dose.
Figure 7.1 Time course of a bolus dose of propofol.
The pharmacodynamic profile of propofol lags behind plasma concentration due to the short delay in access to and from the CNS. Time-to-peak effect of a bolus dose of propofol is in the order of a few minutes (‘t’, Figure 7.1) and has been described by different models as 1.7–3.9 minutes, so the brain concentration of propofol is lower than that of plasma on induction but higher during the elimination phase. There is a relationship between plasma concentration and effect when equilibrium is reached between brain and plasma, but the lag-time before equilibrium is reached must be accounted for in a kinetic model. Modelling this lag-time requires use of depth of anaesthesia monitoring, usually the bispectral monitor (BIS), as a surrogate measure of brain (effect compartment) concentration.
Anaesthesia with propofol can be induced and maintained by its delivery according to a three-compartment model with an effect compartment that equilibrates with the central compartment. The lag-time referred to above between plasma and effect compartments is included by considering the effect compartment as one with no volume but a rate constant of elimination, keo. The half-life for equilibration is known as the t1/2keo.
Remifentanil
Remifentanil is a fentanyl derivative that is a pure µ agonist. It has an ester linkage, which is very rapidly broken down by plasma and non-specific tissue esterases, particularly in muscle. The metabolites have minimal pharmacodynamic activity. With increasing age, muscle bulk decreases and this significantly influences the rate of remifentanil metabolism. The offset of clinical activity is rapid and not greatly affected by duration of infusion; as discussed below remifentanil has a relatively constant context-sensitive half-time between 3 and 8 minutes. Therefore, a patient may be maintained on a remifentanil infusion for a long period, without significant drug accumulation as seen with other opioids. The advantage of this pharmacokinetic profile is that a patient may be given prolonged infusions of remifentanil for analgesia during surgery, with rapid offset of action when this is no longer required. The potential disadvantage is that its analgesic effect wears off so rapidly that pain may be a significant problem immediately post-operatively. Inadequate provision of analgesia before discontinuing remifentanil may be associated with hyperalgesia in the recovery area that can be difficult to treat without using high doses of other opiates, which may lead to adverse consequences. Post-operative pain must therefore be anticipated, either by using a regional technique or by the administration of a longer-acting opioid before the end of surgery.
Total Intravenous Anaesthesia
A strict definition of total intravenous anaesthesia (TIVA) refers to the induction and maintenance of anaesthesia using intravenous agents and an oxygen/air mix. Nitrous oxide has been used as an adjunct, but now this is much less common as the familiarity of TIVA increases and the environmental impact of nitrous oxide is better understood. The intravenous agent(s) may be given by manual infusion or by an infusion device programmed with pharmacokinetic model(s) to target either plasma (TCI plasma) or brain (TCI effect) concentration of drug. When using an infusion of propofol or remifentanil there is no ‘point-of-delivery’ measure of the target concentration comparable to the end-tidal monitoring of inhalational agents. A TCI will display a calculated value for plasma concentration based on the software model used and the information it has been given, which may include some or all of the patient’s weight, height, age and sex.
Manual Total Intravenous Anaesthesia: The Bristol Model
The Bristol algorithm provides a simple manual infusion scheme for propofol to achieve a target blood concentration of about 3 µg.ml−1 within 2 minutes and to maintain this level for the duration of surgery (see Figure 7.2). This is based on plasma concentrations obtained from healthy patients premedicated with temazepam and induced with fentanyl 3 µg.kg−1 followed by a propofol bolus of 1 mg.kg−1. Induction is followed by a variable-rate infusion of propofol at 10 mg.kg−1.h−1 for 10 minutes, 8 mg.kg−1.h−1 for 10 minutes then 6 mg.kg−1.h−1 thereafter. This ‘10–8–6’ infusion scheme was supplemented with nitrous oxide and patients were ventilated. Clearly, if higher plasma concentrations of propofol are needed to maintain adequate anaesthesia, then the 10–8–6 algorithm should be adjusted appropriately or an additional intravenous supplement used, such as a remifentanil infusion.
Although anaesthesia can be delivered using a manual infusion, studies have shown that using a TCI provides anaesthesia using a lower total dose of propofol with the associated benefits of more haemodynamic stability and a more rapid awakening.
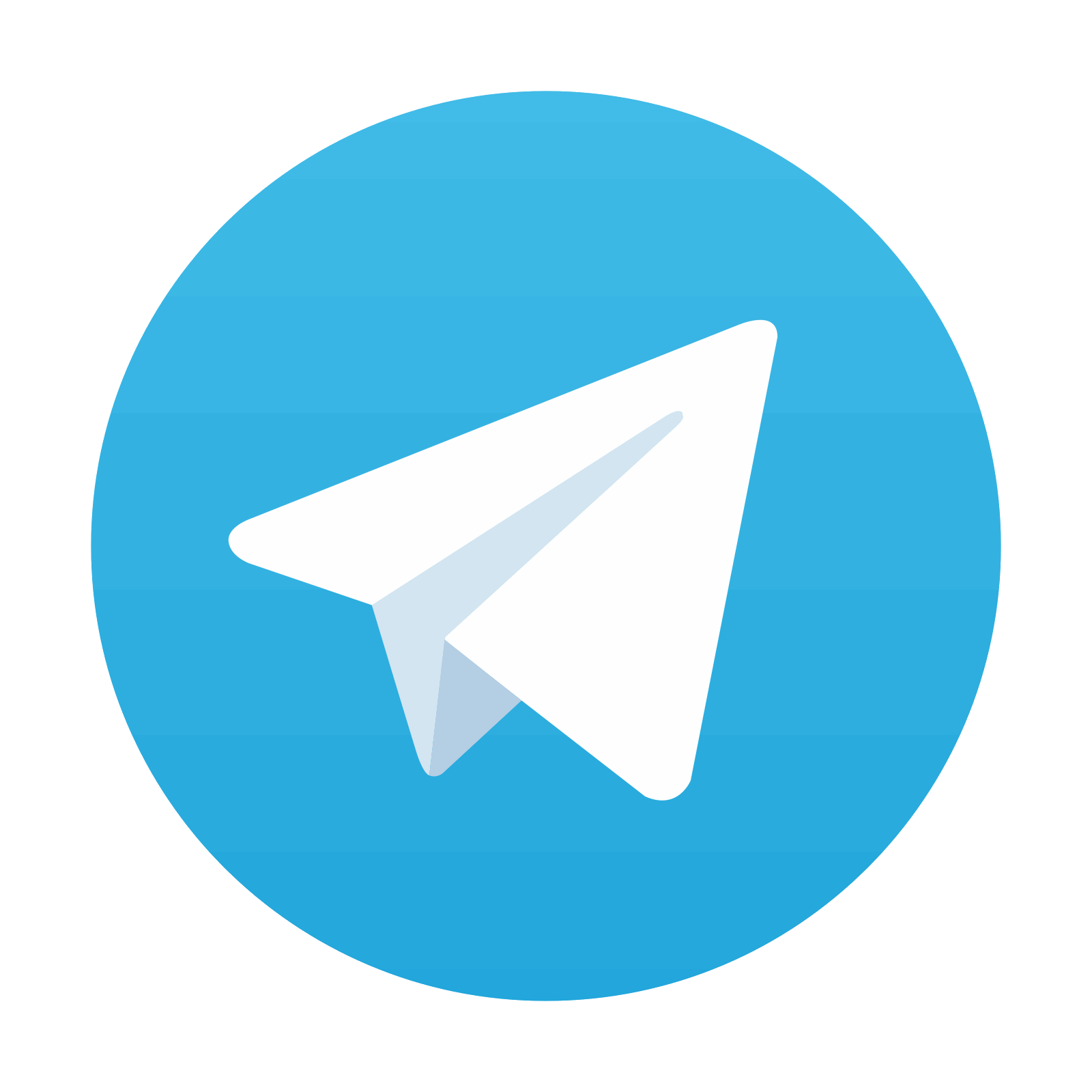
Stay updated, free articles. Join our Telegram channel

Full access? Get Clinical Tree
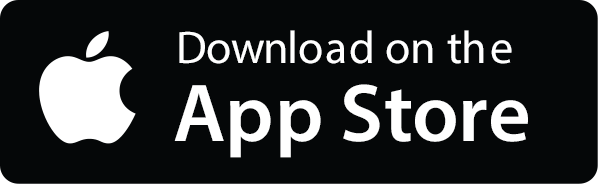
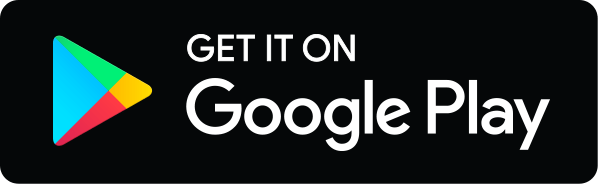
