Abstract
Drugs may act in a number of ways to exert their effect. These range from relatively simple non-specific actions that depend on the physicochemical properties of a drug to highly specific and stereoselective actions on proteins in the body, namely enzymes, voltage-gated ion channels and receptors.
Mechanisms of Drug Action
Drugs may act in a number of ways to exert their effect. These range from relatively simple non-specific actions that depend on the physicochemical properties of a drug to highly specific and stereoselective actions on proteins in the body, namely enzymes, voltage-gated ion channels and receptors.
Actions Dependent on Chemical Properties
The antacids exert their effect by neutralising gastric acid. The chelating agents are used to reduce the concentration of certain metallic ions within the body. Dicobalt edetate chelates cyanide ions and may be used in cyanide poisoning or following a potentially toxic dose of sodium nitroprusside. The reversal agent sugammadex (a γ-cyclodextrin) selectively chelates rocuronium and reversal is possible from deeper levels of neuromuscular block than can be effected with the anticholinesterases.
Enzymes
Enzymes are biological catalysts, and most drugs that interact with enzymes are inhibitors. The results are two-fold: the concentration of the substrate normally metabolised by the enzyme is increased and that of the product(s) of the reaction is decreased. Enzyme inhibition may be competitive (edrophonium for anticholinesterase), non-competitive or irreversible (aspirin for cyclo-oxygenase and omeprazole for the Na+/H+ ATPase). Angiotensin-converting enzyme (ACE) inhibitors such as ramipril prevent the conversion of angiotensin I to II and bradykinin to various inactive fragments. Although reduced levels of angiotensin II are responsible for the therapeutic effects when used in hypertension and heart failure, raised levels of bradykinin may cause an intractable cough.
Voltage-gated Ion Channels
Voltage-gated ion channels are involved in conduction of electrical impulses associated with excitable tissues in muscle and nerve. Several groups of drugs have specific blocking actions at these ion channels. Local anaesthetics act by inhibiting sodium channels in nerve membrane, several anticonvulsants block similar channels in the brain, calcium channel blocking agents act on vascular smooth muscle ion channels and anti-arrhythmic agents block myocardial ion channels. These actions are described in the relevant chapters in Sections II and III.
Receptors
A receptor is a protein, often integral to a membrane, containing a region to which a natural ligand binds specifically to bring about a response. A drug acting at a receptor binds to a recognition site where it may elicit an effect (an agonist), prevent the action of a natural ligand (an inhibitor), or reduce a constitutive effect of a receptor (an inverse agonist). Natural ligands may also bind to more than one receptor and have a different mechanism of action at each (e.g. ionotropic and metabotropic actions of γ-aminobutyric acid (GABA) at GABAA and GABAB receptors).
Receptors are generally protein or glycoprotein in nature and may be associated with or span the cell membrane, be present in the membranes of intracellular organelles or be found in the cytosol or nucleus. Those in the membrane are generally for ligands that do not readily penetrate the cell, whereas those within the cell are for lipid-soluble ligands that can diffuse through the cell wall to their site of action, or for intermediary messengers generated within the cell itself.
Receptors may be grouped into three classes depending on their mechanism of action: (1) altered ion permeability; (2) production of intermediate messengers; and (3) regulation of gene transcription (Figure 3.1).
Figure 3.1 Mechanism of action of the three groups of receptors.
Altered Ion Permeability: Ion Channels
Receptors of this type are part of a membrane-spanning complex of protein subunits that have the potential to form a channel through the membrane. When opened, such a channel allows the passage of ions down their concentration and electrical gradients. Here, ligand binding causes a conformational change in the structure of this membrane protein complex, allowing the channel to open and so increasing the permeability of the membrane to certain ions (ionotropic). There are three important ligand-gated ion channel families: the pentameric, the ionotropic glutamate and the ionotropic purinergic receptors.
The Pentameric Family
The pentameric family of receptors has five membrane-spanning subunits. The best-known example of this type of ion channel receptor is the nicotinic acetylcholine receptor at the neuromuscular junction. It consists of one β, one ε, one δ and two α subunits. Two acetylcholine molecules bind to the α subunits, resulting in a rapid increase in Na+ flux through the ion channel formed, leading to membrane depolarisation.
Another familiar member of this family is the GABAA receptor, in which GABA is the natural ligand. Conformational changes induced when the agonist binds cause a chloride-selective ion channel to form, leading to membrane hyperpolarisation. The benzodiazepines (BDZs) can influence GABA activity at this receptor but augment chloride ion conductance by an allosteric mechanism (see below for explanation).
The 5-HT3 receptor is also a member of this pentameric family; it is the only serotonin receptor to act through ion-channel opening.
Ionotropic Glutamate
Glutamate is an excitatory neurotransmitter in the central nervous system (CNS) that works through several receptor types, of which N-Methyl-D-aspartate (NMDA), α-amino-3-hydroxy-5-methyl-4-isoxazolepropionic acid (AMPA) and kainate are ligand-gated ion channels. The NMDA receptors are comprised of two subunits, one pore-forming (NR1) and one regulatory that binds the co-activator, glycine (NR2). In vivo, it is thought that the receptors dimerise, forming a complex with four subunits. Each NR1 subunit has three membrane-spanning helices, two of which are separated by a re-entrant pore-forming loop. NMDA channels are equally permeable to Na+ and K+ but have a particularly high permeability to the divalent cation, Ca2+. Ketamine, xenon and nitrous oxide are non-competitive antagonists at these receptors.
Ionotropic Purinergic Receptors
This family of receptors includes PX1 and PX2. Each has two membrane-spanning helices and no pore-forming loops. They form cationic channels that are equally permeable to Na+ and K+ but are also permeable to Ca2+. These purinergic receptors are activated by ATP and are involved in mechanosensation and pain. These are not to be confused with the two G-protein coupled receptor forms of purinergic receptors, which are distinguished by selectivity for adenosine or ATP.
Production of Intermediate Messengers
There are several membrane-bound systems that transduce a ligand-generated signal presented on one side of the cell membrane into an intracellular signal transmitted by intermediate messengers. The most common is the G-protein coupled receptor system but there are others including the tyrosine kinase and guanylyl cyclase systems.
G-protein Coupled Receptors and G-proteins
G-protein coupled receptors (GPCRs) are membrane-bound proteins with a serpentine structure consisting of seven helical regions that traverse the membrane. G-proteins are a group of heterotrimeric (three different subunits, α, β and γ) proteins associated with the inner leaflet of the cell membrane that act as universal transducers involved in bringing about an intracellular change from an extracellular stimulus. The GPCR binds a ligand on its extracellular side and the resultant conformational change increases the likelihood of coupling with a particular type of G-protein resulting in activation of intermediate messengers at the expense of guanylyl triphosphate (GTP) breakdown. This type of receptor interaction is sometimes known as metabotropic in contrast with ionotropic for ion-channel forming receptors. As well as transmitting a stimulus across the cell membrane the G-protein system produces signal amplification, whereby a modest stimulus may have a much greater intracellular response. This amplification occurs at two levels: a single activated GPCR can stimulate multiple G-proteins and each G-protein can activate several intermediate messengers.
G-proteins bind GDP and GTP, hence the name ‘G-protein’. In the inactive form GDP is bound to the α subunit but on interaction with an activated GPCR, GTP replaces GDP, giving a complex of α-GTP-βγ. The α-GTP subunit then dissociates from the βγ dimer and activates or inhibits an effector protein, either an enzyme, such as adenylyl cyclase or phospholipase C (Figure 3.2) or an ion channel. For example, β-adrenergic agonists activate adenylyl cyclase and opioid receptor agonists, such as morphine, depress transmission of pain signals via inhibition of N-type Ca2+ channels through G-protein mechanisms. In some systems, the βγ dimer can also activate intermediary mechanisms.
Figure 3.2 Effect of ligand-binding to G-protein coupled receptor (GPCR). Ligand binding to the 7-TMD GPCR favours association with the G-Protein, which allows GTP to replace GDP. The α unit then dissociates from the G-protein complex to mediate enzyme and ion-channel activation/inhibition.
The α-subunit itself acts as a GTPase enzyme, splitting the GTP attached to it to regenerate an inactive α-GDP subunit. This then reforms the entire inactive G-protein complex by recombination with another βγ dimer.
The α subunit of the G-proteins shows marked variability, with at least 17 molecular variants arranged into three main classes. Gs type G-proteins have α subunits that activate adenylyl cyclase, Gi have α subunits that inhibit adenylyl cyclase and Gq have α subunits that activate phospholipase C. Each GPCR will act via a specific type of G-protein complex and this determines the outcome from ligand-receptor coupling. It is known that the ratio of G-protein to GPCR is in favour of the G-proteins in the order of about 100 to 1, permitting signal amplification. Regulation of GPCR activity involves phosphorylation at the intracellular carboxyl-terminal that encourages binding of a protein, β-arrestin, which is the signal for removal of the receptor protein from the cell membrane. The binding of an agonist may increase phosphorylation and so regulate its own effect, accounting for tachyphylaxis seen with β-adrenergic agonists.
Adenylyl cyclase catalyses the formation of cAMP, which acts as a final common pathway for a number of extracellular stimuli. All β-adrenergic effects are mediated through Gs and opiate effects through Gi. The cAMP so formed acts by stimulating protein kinase A, which has two regulatory (R) and two catalytic (C) units. cAMP binds to the R unit, revealing the active C unit, which is responsible for the biochemical effect, and it may cause either protein synthesis, gene activation or changes in ionic permeability.
cAMP formed under the regulation of G-proteins is broken down by the action of the phosphodiesterases (PDEs). The PDEs are a family of five isoenzymes, of which PDE III is the most important in heart muscle. PDE inhibitors, such as theophylline and enoximone, prevent the breakdown of cAMP so that intracellular levels rise. Therefore, in the heart, positive inotropy is possible by either increasing cAMP levels (with a β-adrenergic agonist or a non-adrenergic inotrope such as glucagon), or by reducing the breakdown of cAMP (with a PDE III inhibitor such as milrinone).
Phospholipase C is also under the control of G-proteins, but the α subunit is of the Gq type. Activation of Gq-proteins by formation of an active ligand–receptor complex promotes the action of phospholipase C. This breaks down a membrane lipid, phosphatidylinositol 4,5-bisphosphate (PIP2), to form inositol triphosphate (IP3) and diacylglycerol (DAG).
The two molecules formed have specific actions; IP3 causes calcium release in the endoplasmic reticulum, and DAG causes activation of protein kinase C, with a variety of biochemical effects specific to the nature of the cell in question. Increased calcium levels act as a trigger to many intracellular events, including enzyme action and hyperpolarisation. Again, the common messenger will cause specific effects according to the nature of the receiving cellular subcomponent.
α1-Adrenoceptors, the muscarinic cholinergic types 1, 3 and 5 as well as angiotensin II type 1 receptors exert their effects by activation of Gq-proteins.
Membrane guanylyl cyclase Some hormones such as atrial natriuretic peptide mediate their actions via membrane-bound receptors with intrinsic guanylyl cyclase activity. As a result cGMP levels increase and it acts as a secondary messenger by phosphorylation of intracellular enzymes.
Nitric oxide exerts its effects by increasing the levels of intracellular cGMP by stimulating a cytosolic guanylyl cyclase rather than a membrane-bound enzyme.
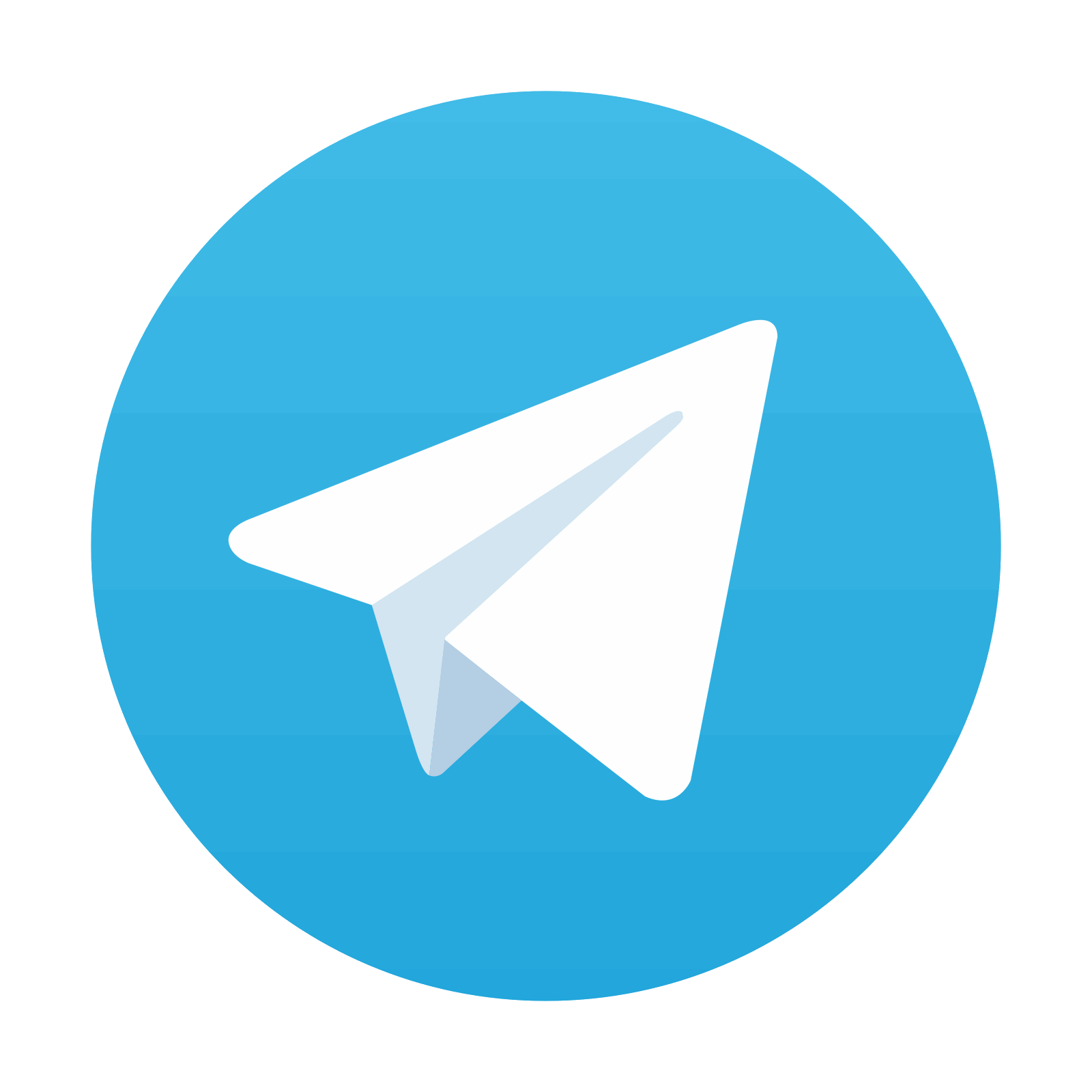
Stay updated, free articles. Join our Telegram channel

Full access? Get Clinical Tree
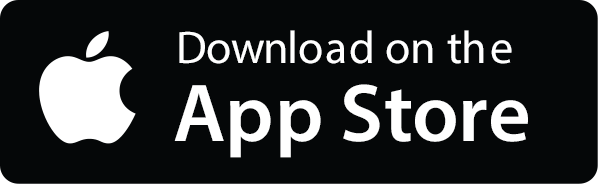
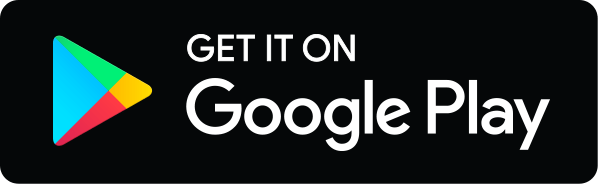
