Abstract
Exercise is a major physiological challenge to the body, affecting all the main body systems. An accompanying increase in muscle metabolic rate results in an increase in O2 demand and a requirement for an increased rate of removal of CO2 and other metabolites, including lactic acid and ketone bodies. Exercise thus requires substantial increases in muscle blood flow with maintenance of mean arterial pressure (MAP). In addition, despite the increased rate of energy metabolism, normoglycaemia must be preserved. Finally, exercising muscle generates a large amount of heat, yet core temperature must be controlled.
Exercise is a major physiological challenge to the body, affecting all the main body systems. An accompanying increase in muscle metabolic rate results in an increase in O2 demand and a requirement for an increased rate of removal of CO2 and other metabolites, including lactic acid and ketone bodies. Exercise thus requires substantial increases in muscle blood flow with maintenance of mean arterial pressure (MAP). In addition, despite the increased rate of energy metabolism, normoglycaemia must be preserved. Finally, exercising muscle generates a large amount of heat, yet core temperature must be controlled.
What is the difference between dynamic and static exercise?
Exercise involves the activation of skeletal muscle. It may be classified as:
Dynamic (or isotonic), where muscles rhythmically contract and relax, moving joints (e.g. running);
Static (or isometric), where muscles contract against a resistance but do not lengthen or shorten (e.g. weight lifting).
Both types of exercise may involve the same muscles, but they differ in their effects on muscle blood flow and metabolism:
During dynamic exercise, there is marked muscle capillary bed vasodilatation in response to aerobic metabolic activity. Accordingly, systemic vascular resistance (SVR) falls. The baroreceptors initiate a tachycardia in response to the consequent reduction in diastolic blood pressure (DBP).
During static exercise, the muscle capillaries are externally compressed by sustained muscle contraction, with a resultant increase in anaerobic metabolism. Vessel compression results in an increase in SVR, which causes an increase in DBP. In addition, sympathetic nervous activity increases heart rate (HR), but to a lesser extent than dynamic exercise.
What types of skeletal muscle fibres are there?
There are two main types of skeletal muscle fibre:
Type I (slow-twitch or fatigue-resistant) fibres. This type of muscle fibre derives its metabolic energy from the oxidative metabolism of triglycerides and is therefore rich in myoglobin (hence its red appearance), mitochondria and capillaries. Type I fibres are found in groups of muscles where sustained contraction is required, such as the postural muscles. Type I fibres contract more slowly but are resistant to fatigue.
Type II (fast-twitch) fibres. There are two types:
– Type IIa (fast-twitch oxidative) fibres;
– Type IIb (fast-twitch glycolytic) fibres.
Type IIb fibres are more reliant on anaerobic metabolism (glycolysis) for ATP generation and thus have larger glycogen stores; their lack of myoglobin leads to a white appearance. These fibres occur in muscles that require short, rapid, powerful movements for activities such as sprinting. Because of the limited capacity of anaerobic metabolism to generate ATP, type IIb muscle fibres are more prone to fatigue. Type IIa fibres are functionally intermediate between type IIb and type I fibres. They utilise both aerobic and anaerobic metabolism using large glycogen stores and contract more slowly, but are more resistant to fatigue than type IIb fibres.
Individual muscles contain varying mixtures of type I, IIa and IIb fibres, with the proportions of each fibre type depending on the muscle’s function. The percentage of each fibre type also differs widely between individuals. Individuals genetically equipped with a greater proportion of type IIb fibres will be better sprinters than those who have a higher proportion of type I fibres, who make better endurance athletes.
Which substrates are used by skeletal muscle to generate energy for contraction?
In common with other cells, skeletal muscle uses ATP as the direct energy source to power contractions. However, only a small amount of ATP is stored in the muscles, enough for 1–2 s of muscle contraction. It is therefore essential that ATP can be rapidly regenerated. There are a number of means by which the muscles do this:
Breakdown of phosphocreatine. This is a high-energy molecule that is used to rapidly re-synthesise ATP from adenosine diphosphate (ADP). Despite muscles only having sufficient phosphocreatine stores for around 7 s of intense exercise, it acts as an important energy buffer that allows time for the more definitive energy-generating processes to occur.
Glycolysis utilises either glucose or the muscle glycogen store to generate ATP without the need for O2. Considering the number of steps involved (see Chapter 77), glycolysis results in a rapid but relatively inefficient increase in ATP synthesis:
– The maximum glycolysis rate is achieved within a few seconds of the onset of exercise.
– The glycolytic breakdown of glucose generates two ATP molecules, whilst that of glycogen generates three ATP molecules.
Oxidative phosphorylation. This is the most efficient mechanism of generating ATP. Complete breakdown of glucose (through glycolysis, the citric acid cycle and oxidative phosphorylation by the electron transport chain) generates 36 ATP molecules.
Fat metabolism. Metabolism of fats through β-oxidation (see Chapter 77) generates a greater number of ATP molecules than an equivalent weight of carbohydrate. However, such fat mobilisation takes place over a longer time course than the corresponding carbohydrate metabolism and therefore contributes little in the early phases of exercise. Fat metabolism becomes more important during sustained exercise, when muscle and liver glycogen stores become depleted. In marathon running, the sudden fatigue associated with glycogen store depletion is called ‘hitting the wall’.
What is muscle fatigue?
Muscle contraction cannot be sustained indefinitely; muscle fatigue is defined as the decline in the ability of a muscle to generate a force. Peak muscle tension and the velocity of contraction may be reduced.
Muscle fatigue is a protective mechanism; it stops the muscle from contracting to the point where it runs out of ATP, which would result in rigor mortis or even cell apoptosis. The exact mechanism underlying muscle fatigue is unknown, with a number of factors thought to be involved:
Increased ADP and phosphate as a result of ATP breakdown, which has been variously suggested to reduce Ca2+ reuptake into the sarcoplasmic reticulum (see Chapter 54) or to trigger the opening of ATP-sensitive K+ channels, which reduces muscle membrane excitability.
Increased extracellular K+. In severe exercise, arterial K+ concentration can rise as high as 8 mmol/L (the exercising heart seems to be protected from the effects of hyperkalaemia). The muscle interstitial K+ concentration rises even higher, up to 12 mmol/L. Hyperkalaemia is thought to contribute to muscle fatigue.
Accumulation of lactate within the muscle. Intense exercise may inhibit enzymes involved in aerobic metabolism.
Exhaustion of muscle glycogen stores following prolonged exercise.
What physiological changes occur in anticipation of exercise?
Some physiological changes occur before the onset of exercise. Merely anticipating exercise results in greater sympathetic outflow and parasympathetic nervous system inhibition, causing:
In the venous system: venoconstriction, which causes an increase in the venous return to the heart.
In the heart: an increase in HR and myocardial contractility, which, in conjunction with the increased venous return, leads to an increase in cardiac output (CO).
In skeletal muscle: vasodilatation of capillary beds through activation of sympathetic cholinergic fibres. This is an important mechanism that prevents large increases in blood pressure following the anticipatory increase in CO.
In the peripheries: vasoconstriction in the gut and skin.
The cardiovascular effects vary depending on the anticipated duration and intensity of the exercise. For example, anticipation of a short sprint results in a greater increase in HR than anticipation of an endurance run. This is because it would be impossible to increase HR to near-maximal levels over the short duration of a sprint distance, whereas in a marathon there is ample time to instigate the cardiovascular response. This is an example of feedforward control.
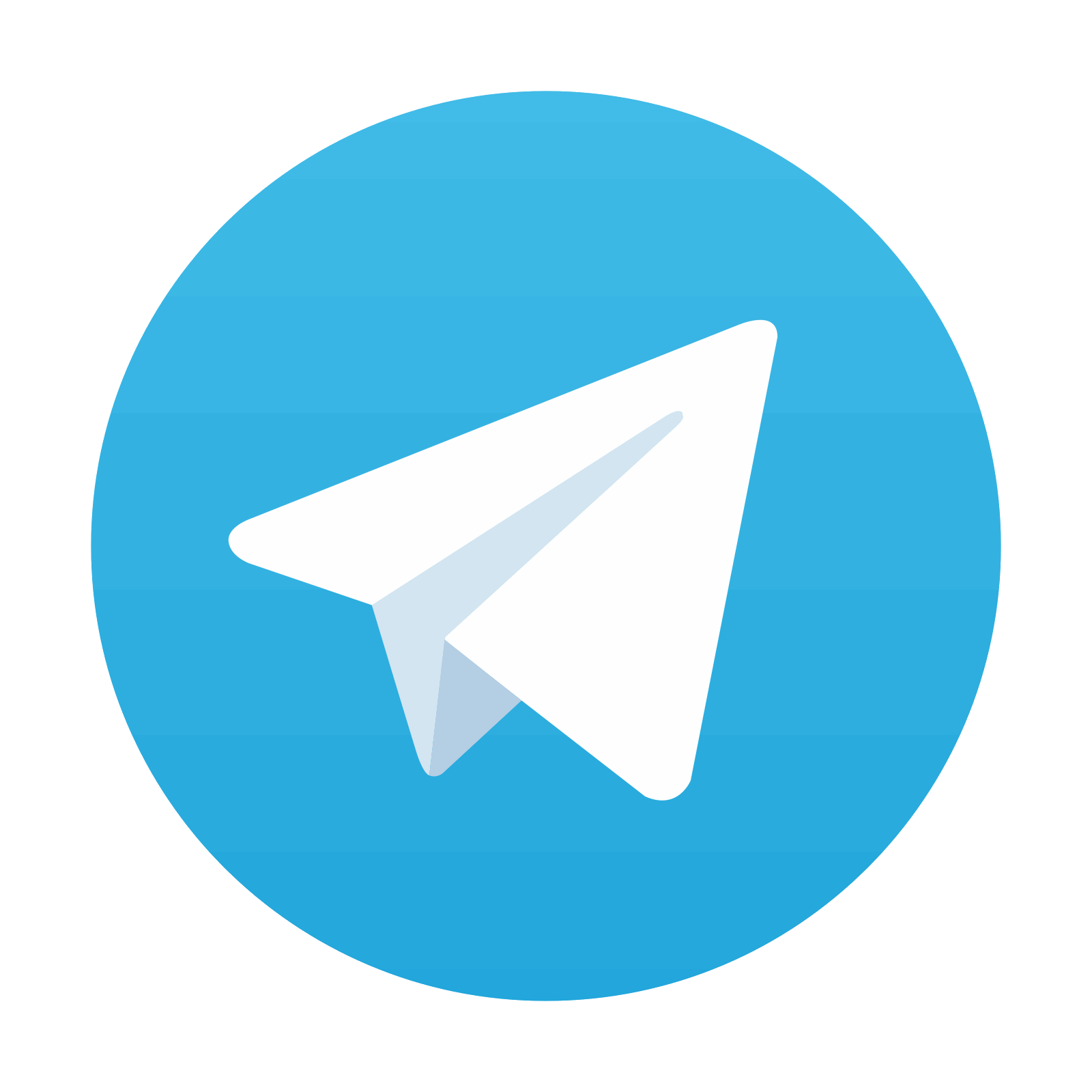
Stay updated, free articles. Join our Telegram channel

Full access? Get Clinical Tree
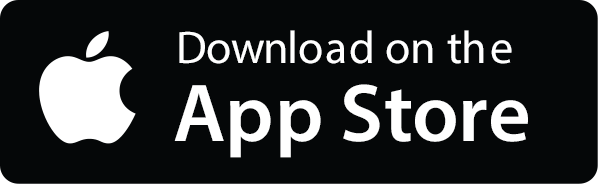
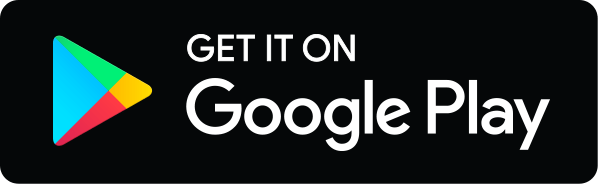