Abstract
The cardiac output (CO) is the volume of blood ejected by the left ventricle (LV) or right ventricle (RV) per minute.
Which factors affect the cardiac output?
The cardiac output (CO) is the volume of blood ejected by the left ventricle (LV) or right ventricle (RV) per minute.
CO is directly dependent on two factors: heart rate (HR) and stroke volume (SV).
At rest, the typical SV is 70 mL and HR is 75 bpm.
Typical resting CO is therefore 70 × 75 = 5.25 L/min, but CO may increase fivefold during maximal exercise.1
In turn, SV is determined by three factors: preload, myocardial contractility and afterload.
Preload is defined as the intraluminal pressure that stretches the RV or LV to its end-diastolic dimensions. Preload is therefore related to the diastolic length of the cardiac myocyte. According to Starling’s law, the force of cardiac myocyte contraction depends on the resting diastolic length of the ventricular fibres (see Chapter 30): increased preload produces an increased SV.
In practice, preload is very difficult to measure. It is impossible to measure sarcomere length in vivo, so surrogate measurements are used: left ventricular end-diastolic volume (LVEDV; measured by echocardiography) or, more commonly, left ventricular end-diastolic pressure.2 Similarly:
– Central venous pressure provides an indication of right ventricular preload, as it affects right ventricular end-diastolic volume (RVEDV).
– Pulmonary capillary wedge pressure provides an indication of left ventricular preload, as it affects LVEDV.
Afterload is the stress developed in the left ventricular wall during ejection, and it reflects the force opposing the shortening of cardiac myocytes. As afterload increases, both the rate and extent of sarcomere shortening decrease, resulting in a reduction in SV. Like preload, afterload is not easily measured in vivo and is assessed through surrogate markers:
– Mean arterial pressure (MAP) and systemic vascular resistance (SVR) reflect left ventricular afterload.
– Pulmonary vascular resistance reflects right ventricular afterload.
Myocardial contractility is the intrinsic ability of cardiac myocytes to generate mechanical power at a given preload and afterload. Factors that increase myocardial contractility are said to exert a positive inotropic effect, whilst factors that decrease contractility exert a negative inotropic effect. Contractility is difficult to measure directly, but an index of myocardial contractility is provided by the rate of change of pressure (i.e. the gradient) during the isovolumetric contraction phase of the cardiac cycle (seeChapter 28 and Figure 28.1).
How does the body regulate CO?
CO is not static; it varies depending on the changing requirements of the body. For example:
Age. Taking into account their proportionately greater body surface area (BSA, m2), children have a greater CO than adults.
Exercise. CO may increase up to fivefold in normal individuals.
Pregnancy is associated with an increase in resting CO of up to 50% at term.
Eating is associated with an increase in CO of around 25%.
As discussed above, the main factors that influence CO are HR, preload, myocardial contractility and afterload. The regulation of these four factors is complex, as changes in each rarely occur in isolation. However, HR may undergo a threefold increase (e.g. from 60 bpm to 180 bpm), whereas SV may only increase by around 50% (e.g. from 70 mL to 105 mL). Under normal circumstances, the major factor that influences CO is therefore HR.
HR is set by sinoatrial (SA) node pacemaker activity, which is in turn modulated by the autonomic nervous system (ANS):
– A denervated heart has a basal HR of around 100–120 bpm.
– At rest, the parasympathetic nervous system (via the vagus nerve) is tonically active in the heart (note: this is an exception for the ANS; elsewhere, it is the sympathetic nervous system that is tonically active). Acetylcholine is continually released from parasympathetic nerve terminals, reducing the resting HR to 60–70 bpm through its effect at muscarinic M2 receptors.
– At the onset of exercise, parasympathetic tone is withdrawn, which increases HR. Noradrenaline is released from sympathetic nerve terminals and adrenaline is released from the adrenal medulla, both of which increase HR through activation of β1-adrenoceptors.
– Up to around 140 bpm, CO increases with increasing HR.
– Above 150 bpm, the diastolic cardiac filling time becomes very short (~0.15 s). As ventricular filling can only occur during diastole, SV falls with a consequent reduction in CO (see Chapter 28).
– When HR is rapid, as may occur with ventricular tachycardia, the fall in CO may be sufficient to cause myocardial ischaemia, thus further reducing myocardial contractility; a vicious cycle ensues.
Preload. Starling’s law ensures that the cardiac outputs of the RV and LV are exactly matched. Therefore, the main determinant of both right and left ventricular preloads is the venous return to the RV; that is, RVEDV. Venous return depends on a number of factors, which are discussed in detail in Chapter 37.
Myocardial contractility is affected by four factors:
– The sympathetic nervous system. Release of noradrenaline from cardiac sympathetic neurons (and to a lesser extent circulating noradrenaline and adrenaline) increases myocardial contractility through its action on β1-adrenoceptors.
– Tachycardia. Intrinsic myocardial contractility is increased when the HR is high. This is known as the ‘Bowditch effect’.
– Drugs with positively inotropic effects include dobutamine, isoprenaline, glucagon, enoximone and digoxin. Drugs with negatively inotropic effects include β-blockers, Ca2+ channel blockers and anaesthetic agents.
– Disease states may reduce myocardial contractility, such as sepsis, myocarditis, ischaemic heart disease, electrolyte and acid–base disturbance.
Positive inotropy increases myocardial O2 demand. As myocardial contractility increases, there may come a point where O2 delivery becomes insufficient, resulting in myocardial ischaemia. This is especially so in patients with coronary artery disease, where atherosclerosis limits coronary blood flow. Ischaemic myocardium cannot contract as effectively, and this compromises SV.
Afterload, which is governed by SVR. As discussed above, an increase in afterload results in a reduction in SV. As less blood is ejected from the heart per beat, there is a greater volume of blood remaining in the ventricle at end-systole and therefore (following the ventricular filling phase) a greater LVEDV. According to Starling’s law, a greater LVEDV produces a greater SV. Overall, following a sudden increase in afterload, SV transiently decreases before gradually returning to normal.
In addition, an increase in afterload causes an intrinsic increase in inotropy. This ‘Anrep effect’ ensures that increases in afterload cause smaller reductions in SV than would be predicted from the Frank–Starling mechanism alone.
What is the Bowditch effect?
The Bowditch effect (also known as the ‘Treppe effect’ or the ‘staircase effect’) is an intrinsic autoregulatory phenomenon in which tachycardia leads to increased myocardial contractility. The mechanism for this is thought to be:
As HR increases, the time period for each cardiac cycle falls, with the diastolic interval shortened more than the systolic interval.
At high HR, there is increased systolic Ca2+ influx through the L-type Ca2+ channels.
In addition, the diastolic Na+ efflux due to the Na+/K+-ATPase cannot keep pace with the systolic influx of Na+.
The Na+/Ca2+ exchanger is normally responsible for the low intracellular Ca2+ concentration. However, with tachycardia, the increase in cytosolic Ca2+ and Na+ concentrations leads to an accumulation of intracellular Ca2+, with a consequent positive inotropic effect. This is also seen with digoxin therapy, where the Na+/K+-ATPase is blocked.
What is the cardiac index?
The typical resting CO in a 70‑kg adult is said to be 5–6 L/min, but varies with body size. Cardiac index (CI) is a means of standardising CO based on BSA.
Key equation: CI

Normal resting CI is 3.0–3.5 L min–1 m–2.
Likewise, SV can be standardised based on BSA, resulting in the SV index (SVI):
Key equation: SVI

Normal resting SVI is 33–47 mL m–2 beat–1.
How is MAP related to CO?
MAP describes the average arterial pressure during a single cardiac cycle. Pressure and flow are related by Darcy’s law:
Pressure = flow × resistance
In the case of MAP and CO:
MAP ≈ CO × SVR
NB: This equation holds if right atrial pressure is assumed to be much smaller than MAP. The equation is an oversimplification – in formal terms, arterial and venous circulations should be considered separately, because pressure generation in each circulation occurs by different mechanisms.
In turn, SVR is dependent on the radius of the arterioles, and (to a much lesser extent) the blood viscosity.
Therefore, at a given CO:
MAP is increased by:
– Vasoconstriction, as might take place following catecholamine release.
– Increased blood viscosity, as occurs in patients with paraproteinaemia or polycythaemia rubra vera, who are consequently often hypertensive.
MAP is decreased by:
– Vasodilatation, as occurs in septic shock or following general anaesthesia.
– Decreased blood viscosity.
Aortic stenosis is a degenerative disease of the aortic valve. Lifetime incidence is estimated at 1%. The most common cause of aortic stenosis is repeated mechanical stress causing fibrosis and calcification of a previously normal trileaflet aortic valve, usually presenting over the age of 70. Patients with a congenital bicuspid aortic valve tend to develop aortic stenosis at a younger age.
The normal aortic valve area is 2.6–3.5 cm2. As the area of the aortic valve decreases over time, there is an initial compensatory hypertrophy of the LV: systolic function is maintained, preserving SV and therefore CO. However, this pathological left ventricular hypertrophy has adverse effects:
A decrease in left ventricular compliance, which impairs ventricular relaxation and diastolic filling. Atrial contraction becomes ever more important in left ventricular filling, contributing up to 40% of LVEDV. The development of AF may have devastating consequences in patients with aortic stenosis and occurs more frequently due to increased atrial stretch.
Increased myocardial O2 demand. As the aortic valve becomes increasingly stenotic, a greater left ventricular pressure is required to maintain SV. Therefore, left ventricular O2 demand increases. At the same time, the increase in left ventricular mass and higher left ventricular pressure reduces coronary blood flow. The mismatch between myocardial O2 delivery and demand is why patients with severe aortic stenosis develop angina, despite often having normal coronary arteries.
Patients with severe aortic stenosis (below 1.0 cm2) effectively have a fixed CO. In consequence:
Exertional syncope. Exercise causes a decrease in SVR, which is normally compensated for by an increase in CO, to maintain MAP. In severe aortic stenosis, the required increase in CO cannot be met; MAP falls, leading to a decrease in cerebral blood flow and thus syncope.
Central neuraxial blockade. The decrease in SVR caused by sympathetic blockade cannot be met by an increase in CO, resulting in a devastating reduction in MAP.
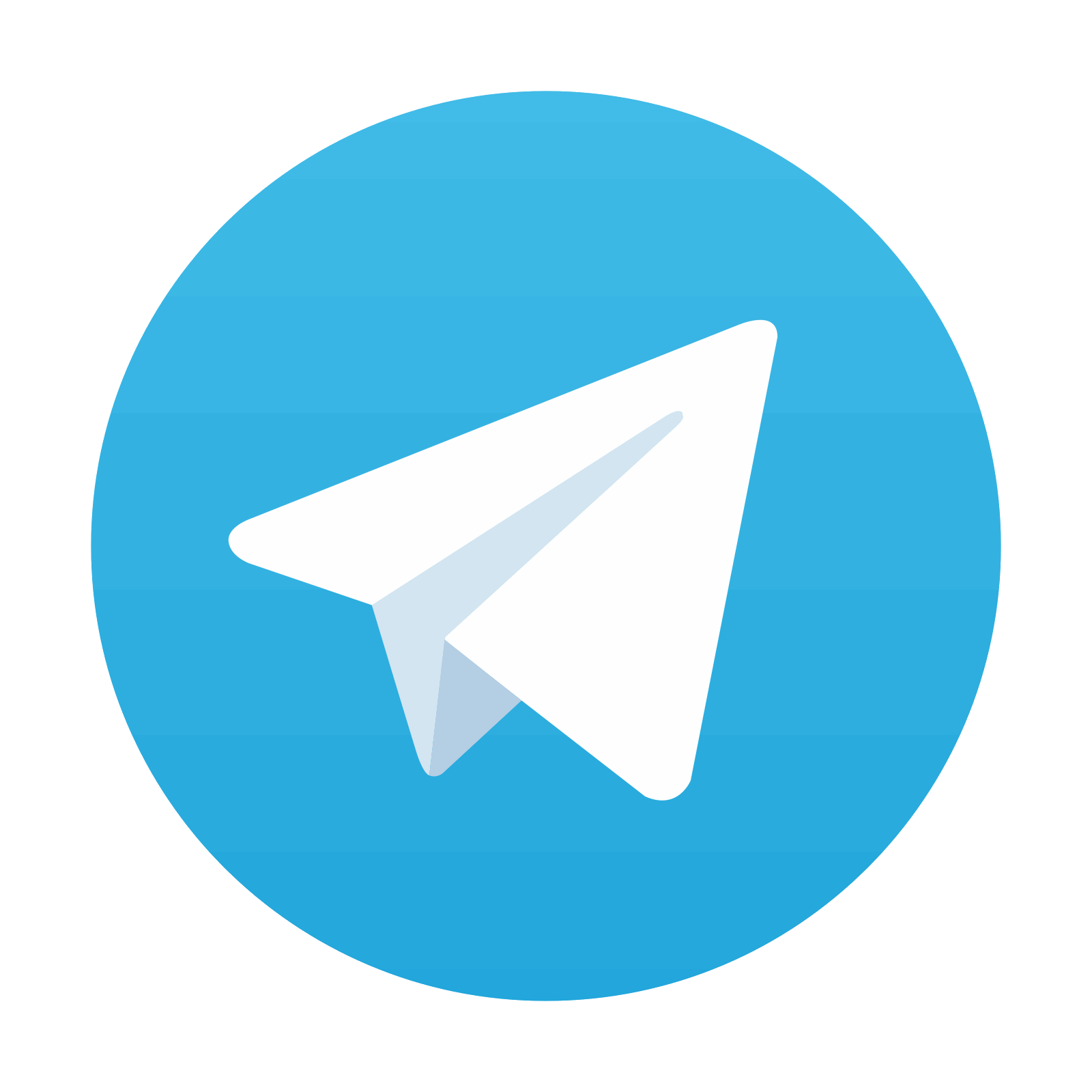
Stay updated, free articles. Join our Telegram channel

Full access? Get Clinical Tree
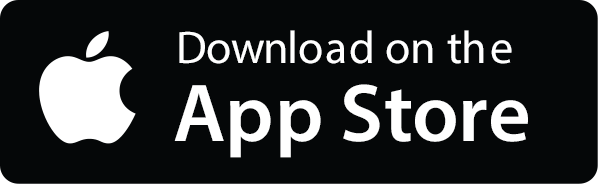
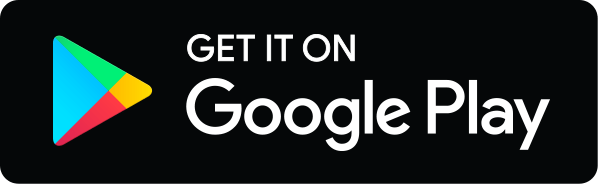
